Spinal cord stimulation (SCS) and intrathecal drug delivery (IDD) are forms of neuromodulation, meaning that they are reversible and nondestructive. SCS is generally limited to conditions such as radiculopathy and pain of CNS orgin. IDD is primarily effective for nociceptive or mixed pain, and its usage is generally limited to conditions within that realm.
Spinal cord stimulation (SCS) and intrathecal drug delivery (IDD) are forms of neuromodulation. Neuromodulation attempts to alter the nervous system through augmentation rather than ablation, meaning that it is reversible and nondestructive. In terms of chronic pain treatment, SCS is primarily effective for neuropathic rather than nociceptive pain. Therefore, in low back-associated pain, it is generally limited to conditions such as radiculopathy and pain of CNS origin. IDD is primarily effective for nociceptive or mixed pain, and its usage is generally limited to conditions within that realm.
Spinal cord stimulation
History
In 1967, Shealy and colleagues opened the door for a new intervention in pain treatment when they reported the ability to inhibit pain by subdural electrical stimulation of the dorsal columns of the spinal cord. Their surgical implantation of a unipolar stimulator directly on the spinal cord was based, in large part, on the theory of Melzack and Wall proposed 2 years earlier. The gate control theory suggested that stimulation of large-diameter primary afferent (Aβ) cutaneous nerves in the spinal cord might inhibit pain transmission and therefore suppress pain perception.
SCS has since evolved at many levels. Perhaps the most important step occurred in 1975, when placement of an epidural stimulator and the development of a system of ring electrodes on a flexible lead capable of percutaneous implantation led to a notable change in the use of the systems. The ability to implant these units without the need for a laminotomy increased the number of practitioners with the technical expertise to permanently place SCS systems.
It became clear that focusing stimulation within a certain region of the spinal cord while avoiding stimulation of unintended spinal structures would improve effectiveness. This notion has evolved in large part through computer modeling developed in the Netherlands in the early 1990s, and is based on electrode spacing, anatomic lead location, and the lead-cord cerebrospinal fluid (CSF) separation. The results of the work by Holsheimer and others helped illuminate several aspects of SCS: the relationship between dorsal column and dorsal root fiber stimulation thresholds; the effect of increasing CSF depth on decreasing the focal intensity of stimulation; and the value of narrow longitudinal fields and diminution in center-to-center contact separation in minimizing dorsal root fiber stimulation.
Microtechnology has contributed significantly over the past decade to improving the efficacy of SCS. Multiple configurations of percutaneous and surgical leads are available. Pulse generators have evolved from external units transmitting via radio to self-contained, rechargeable, implanted units. Microcomputer technology has provided an opportunity for patient-controlled programming, allowing multiple programs to address postural changes, thereby increasing patient satisfaction.
Description
A spinal cord stimulator is essentially an electrical circuit opening at a battery source and closing at the tissues, and in its simplest form is based on Ohm’s law (I = V/R). The current or voltage is converted into pulses and transmitted to the various electrodes of the SCS leads. The circuit is closed by the tissues in the spinal canal creating stimulation to nervous tissue. As low-threshold primary cutaneous afferents in the dorsal columns are stimulated, the perception of pain is reduced. Theoretically, stimulating the dorsal columns closes the gate over wide areas of the body. In fact, spinal cord stimulation is more complex. For example, SCS is effective for chronic but not acute pain, for neuropathic and sympathetically mediated pain but not nociceptive pain.
Primary afferent spinal neurons must be depolarized and must propagate an action potential to close the gate on peripheral painful input. This outcome occurs when the spinal neuron is made more electrically positive, which is achieved when a negatively charged external electrical stimulus called the cathode is applied and produces a cathodal effect .
Likewise, hyperpolarization occurs when a spinal neuron is made more electrically negative, which prevents propagation of an action potential; such a positively charged external stimulus is called an anode and produces an anodal effect .
One can see that the ability to direct discrete electrical charges via electrodes placed close to the primary afferent spinal neurons allows focused depolarization and impedes the transmission of peripheral nociception and the perception of pain.
The dorsal column target for stimulation is typically several spinal levels higher than the exiting spinal nerves of the dermatome or dermatomes to be covered. This target is medial but in close proximity to the dorsal root structures, both running longitudinally. Because the propagation threshold of neurons in each of these regions is similar, the difference between producing pain-relieving paresthesia and unpleasant dorsal root stimulation is small. This hurdle is cleared by focusing the cathodal effect over the more midline dorsal columns, causing depolarization. Frequently, an anodal effect can be achieved over the dorsal root fibers, preventing their propagation and increasing the effect of the cathode at the dorsal columns.
A single SCS lead placed in the midline decreases the risk of stimulating lateral cord structures. Also, reduced center-to-center contact spacing diminishes the sphere of stimulation at the cathode. Conversely, lost paresthesia from lead migration and decreased ability to obtain bilateral and more complex pain patterns place limits on single midline leads.
Dual lead systems allow a greater amount of electronic steering that theoretically can focus stimulation more precisely at the target site and deeply into the cord structures. The concept of guarding the cathode by placing anodes on either side of it led to tripolar stimulation. Later, this evolved to transverse tripole stimulation using 3 parallel leads, 2 lateral anodes protecting a midline cathode from the dorsal root region and driving stimulation more deeply into the dorsal columns. Unfortunately, power consumption is increased using bi- or tripolar leads and closely spaced electrodes. Current stimulation technology also achieves focused stimulation through interleaved or multichannel stimulation. All these techniques were developed in the hope of increasing axial spine pain coverage, but its clinical effect remains unproven.
Paresthesia must successfully be superimposed over the area of pain for SCS to be an effective treatment. However, even if paresthesia can be superimposed over the area of a patient’s pain, that is not necessarily sufficient to elicit pain relief. Furthermore, computer modeling predicts that the likelihood of achieving paresthesia over the low back is, at best, 45%. This reflects the complicated nature of SCS and the clinical evidence of its greater effectiveness for neuropathic and sympathetic rather than nociceptive pain.
The most important limit to paresthesia seems to be the proximity of the stimulator electrodes to the spinal cord, which in turn is limited by the depth of CSF and epidural fat at any point along the cord. Physiologic impedance is suspected as a secondary effect. Within 3 weeks of implantation of percutaneous leads, the electrodes are generally encapsulated, theoretically stabilizing impedance. However, that is not consistently the case, and further understanding of this phenomenon is needed.
Failed Back Surgery Syndrome
Perhaps the best studied use of SCS in low back-related pain occurs with failed back surgery syndrome (FBSS). FBSS represents a continuum of symptoms with a common genesis—the unfavorable response of lower back and/or radicular symptoms to surgical intervention. Patients with FBSS may have radicular or axial symptoms and commonly have both. Causes include recurrent pathology, surgical failure, postsurgical scarring, and instability. Furthermore, FBSS symptoms are not independent of emotional, psychological, and social influence. Evaluating the efficacy of SCS in FBSS from available literature is an elusive quest, because the definition of FBSS is imprecise.
Despite the obscure definition, many published studies reflect various experiences with SCS in patients with persistent spine-related pain after surgical intervention, and FBSS is a common indication for SCS.
As indicated previously, SCS has largely been effective for neuropathic pain but less so for nociceptive pain. One might suppose this premise would act as a guide for the use of SCS for patients falling under the generous umbrella of FBSS; however, the literature is difficult to interpret.
Several studies have focused primarily on patients with a greater radicular component to their FBSS. After long-term follow-up (mean range from 2.9 to 8 years), pain relief of 50% or greater ranged from 47% to 86%.
In a retrospective study examining SCS for FBSS for predominately chronic axial lower back pain with follow-up ranging from 5 to 19 months, 70% of the patients were satisfied with the outcome. Initial results using dual leads supported diminution in visual analog scale pain levels and narcotic usage, even in patients with significant axial pain complaints. However, other studies reflect, at best, an inconsistent ability for SCS to reduce axial low back pain.
Nevertheless, it remains very difficult to compare any of the studies, because patient selection criteria, rates of permanent implantation, outcome measurement, and follow-up are not equitable. Furthermore, rarely are these studies prospective, randomized, and controlled; they are more commonly retrospective. Duration of SCS trials also varies, extending from several days to 4 weeks. Rates of permanent implantation from these trials also vary from 65% to 96% of patients.
There have been several studies that have attempted to evaluate the relative cost-effectiveness of using SCS for FBSS. SCS is an expensive intervention; however, when compared with total health-care outlays and lost productivity, that cost is most often equivocated. One study reported a substantial improvement in health-care costs when comparing successful SCS for FBSS versus treatment with conventional medical management (CMM). Others have found SCS to be cost-effective after 2.5 years and 2.1 years of use when compared with CMM. A systematic review found that SCS may increase quality of life and function, and it can be more cost-effective in the long term, despite the high initial device implantation costs.
Technique
Perhaps the greatest benefit of SCS over other interventions is the ability to test the efficacy of the treatment before committing to permanent implantation. Because each trial is specific to the patient, success can more reliably be measured.
Percutaneous trials are straightforward and involve placing a lead or leads into the epidural space through a spinal needle and attaching the exposed leads to an external pulse generator. Permanent percutaneous lead placement is similar to the trial, with subcutaneous surgical placement of the leads and stimulator unit; it is beyond the scope of this chapter to provide greater detail.
Patient Selection and Risks
As with all pain-related interventions, SCS should only be considered after thorough and thoughtful evaluation. Perhaps the single most important aspect is patients accepting their responsibility in the rehabilitation process. Every intervention performed on a patient with low back-related disorders should be considered a rehabilitation process, intended to maximize the patient’s function. Although achieving reduction of symptoms is a significant step in that rehabilitation process, it should not be the final goal.
Patients with major psychiatric disorders, inability to comprehend the goals of the procedure, substance abuse problems, and secondary gain issues are generally excluded from consideration for SCS. In appropriate cases that have not progressed despite conservative pharmacologic and percutaneous interventions, SCS may allow an opportunity for rehabilitation.
Commonly, SCS trial success is defined as pain relief of 50% or greater following implantation. This arbitrary designation is used as a minimal threshold toward pursuing permanent implantation.
Unlikely but potential adverse events include hematoma, epidural hemorrhage, seroma, CSF leakage, infection, erosion, allergic response, lead migration, implant-site pain, loss of pain relief, chest wall stimulation, or paralysis.
Any sources of electromagnetic interference, such as defibrillators, diathermy, electrocautery, magnetic resonance imaging, radiofrequency ablation, and therapeutic ultrasonography, could interact with SCS systems causing damage or stimulation changes.
Patients should avoid aggressive activities that might place stress on spinal cord stimulator leads because they could cause fracture or migration, and postural changes might cause temporary changes in stimulation.
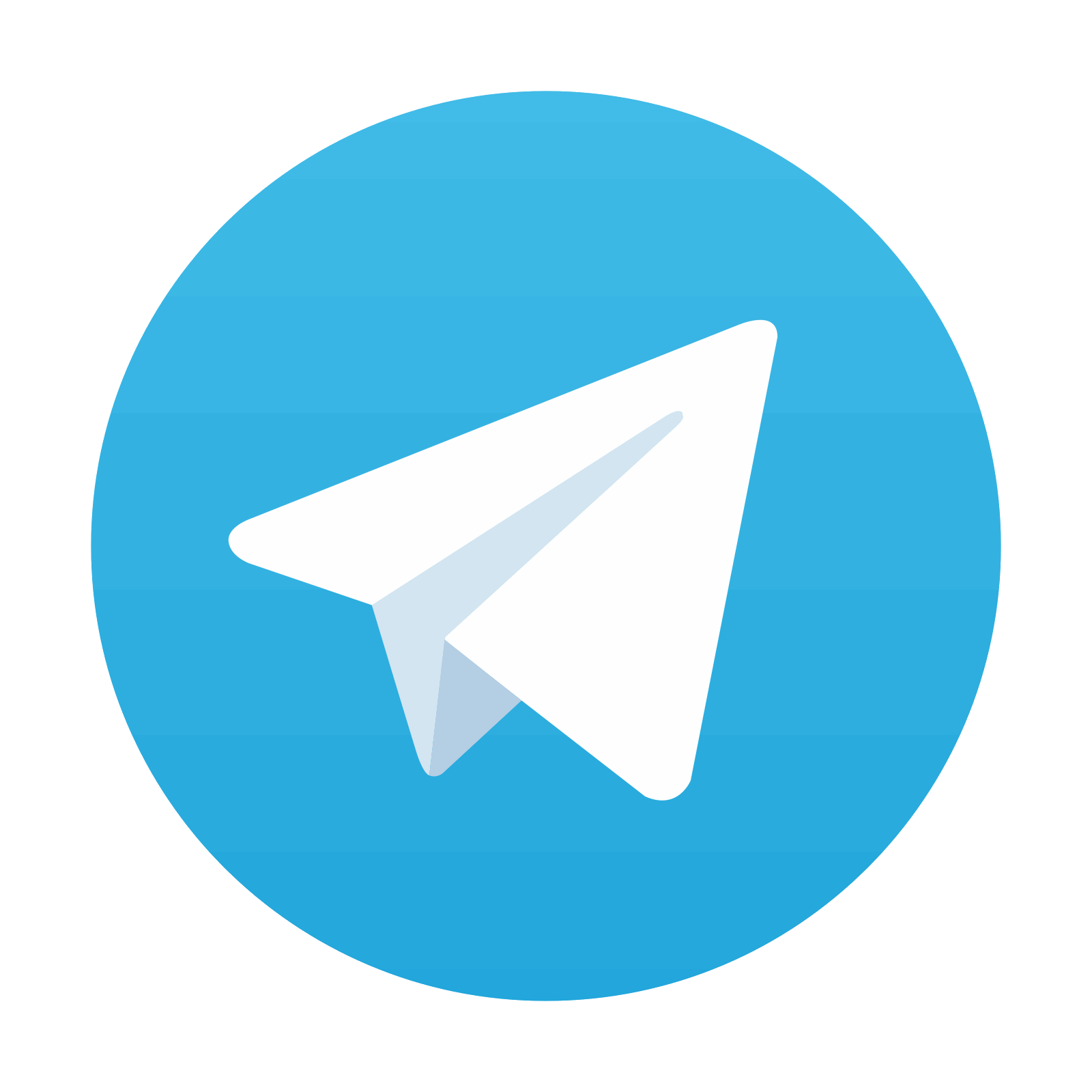
Stay updated, free articles. Join our Telegram channel

Full access? Get Clinical Tree
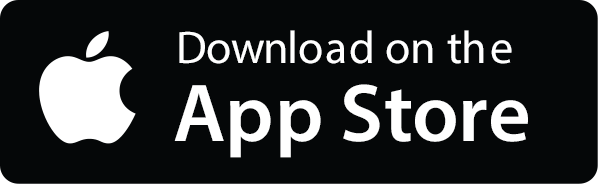
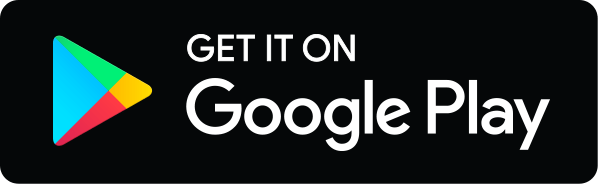