The Use of Scaffolds in the Treatment of Osteochondral Lesions in the Knee: Current Concepts and Future Trends
The treatment of chondral and osteochondral lesions has become a major interest to orthopedic surgeons because most lesions do not heal spontaneously and may predis-pose the joint to the subsequent development of secondary osteoarthritis.1 This poor repair capacity of articular cartilage has led to the development of various surgical techniques.2 Several bone marrow-stimulating procedures directed at the recruitment of bone marrow cells have been widely used to treat local cartilage defects. In this type of procedure, mesenchymal stem cells (MSCs) migrate in the fibrin network of the blood clot.3 However, this fibrin clot is not mechanically stable to withstand the tangential forces.4 The most popular and frequently used is the microfracture technique including abrading the tidemark and creating small holes perpendicular to the subchondral bone plate to allow bleeding into the defect.5 Microfracture usually results in a fibrous–fibrohyaline unstructured repair tissue. This tissue lacks the biomechanical and viscoelastic features of hyaline cartilage. The potential short-term improvement in symptoms is usually followed by repair tissue failure and potentially by gradual deterioration to osteo-arthritis and return of symptoms.6
Since 1987, autologous chondrocytes have been implanted in chondral lesions of the human knee.7 To this end, cartilage is harvested from a non–weightbearing area of the knee joint—for example, the intercondylar notch or the edge of the trochlea—then digested, and the isolated chondrocytes are propagated in vitro in the monolayer culture condition. This procedure, proposed by Brittberg et al in 1994 and called autologous chondrocyte implantation (ACI), is gaining wide scientific and clinical support for use in the repair of focal articular cartilage lesions.7,8 However, during in vitro propagation of the chondrocytes, dedifferentiation of the cells can occur, and afterwards these fibroblast-like chondrocytes show different biosynthetic properties than the original cartilage cells in the knee joint.9
Recently, there has been an increasing interest and awareness of the importance of subchondral bone for its role in cartilage repair. One should carefully consider the subchondral bone in the treatment of articular surface damage, in the evaluation of the results over time and in the determination of the patient′s prognosis. In fact, the conditions of articular cartilage and its supporting bone are tightly coupled and should be viewed as a connected osteochondral unit.10 The ultimate aim of the treatment is the restoration of normal knee function by regenerating hyaline cartilage in the defect and complete integration of the regenerated cartilage with the surrounding cartilage and underlying bone. The treatment should restore the physiological properties of the entire osteochondral unit.
However, none of the currently available treatment options achieve this goal. Ideally, future cartilage repair strategies should (1) be easy and quick to implant, (2) reduce surgical morbidity, (3) not require harvesting of other tissues (e.g., periosteum), (4) exhibit enhanced cell proliferation and maturation, (5) have easier phenotype maintenance, and (6) allow for efficient and complete integration with surrounding articular cartilage.2
Tissue engineering could be an alternative and promising option for the treatment of cartilage defects. Tissue engineering is based on three basic ingredients: scaffolds, growth factors, and cells.11 Scaffolds should be bio-degradable to anchor, deliver, and orient the cells. Growth factors are the instructional cues to guide cartilage growth and differentiation. Finally, cells must be present that are capable of proliferating, producing cartilage matrix, and ultimately reacting as normal cartilage does.2
Scaffolds Design Criteria
Ideally, scaffolds should be versatile in terms of applications and be suitable for resurfacing full-thickness lesions as well as repairing partial-thickness lesions. The latest recommendations on scaffolds’ biochemical and structural requirements and their corresponding function for tissue engineering are as follows2,12:
Biologic compatibility: Inciting ideally no or minimal inflammatory response.
Noncytotoxic: The scaffold should not be cytotoxic to the cells or surrounding tissues.
Three-dimensional (3D) matrix architecture: Allowing a physiologically relevant environment to hold cells and support cell function.
Void space: Highly porous and interconnected pores that allow cell infiltration, diffusion of nutrients and humoral factors as well as waste products to promote cellular proliferation and the production of extracellular matrix.
Surface chemistry and topography: For cell attachment and cell–matrix interactions between grafted and native cartilage.
Biodegradation rate: Scaffold serves as a temporary support for the cells and gives way to functional matrix formation.
Structural anisotropy: Anisotropic mechanical behavior to influence orientation of cells and extracellular matrix (ECM) deposition.
Appropriate mechanical behavior: To allow seamless integration with surrounding cartilage, to withstand in vivo forces, and to avoid stress shielding.
Availability: To be reproducible and easily fabricated into a variety of shapes and sizes to adapt to each patient scenario.
Types of Scaffolds
The use of scaffolds for cartilage repair came into practice when monolayered cultured chondrocytes showed progressive dedifferentiation.9,13 This phenomenon was reverted when they were recultured in 3D media and has led to the development of various scaffolds that can be grouped into four main classes14: (1) protein-based scaffolds (e.g., fibrin, collagen, gelatin…), (2) carbohydrate-based scaffolds (e.g., hyaluronan, agarose, alginate, polylactic/polyglycolic acids,…), (3) synthetic or artificial polymer-based scaffolds (e.g., hydroxyapatite, polyethylene glycol,…), and (4) combination of different scaffolds types.
Experience with Currently Available Scaffold Types
Protein-Based Scaffolds
Fibrin
The protein-based scaffolds include fibrin, collagen, and gelatin. Chondrocytes multiply, retain their morphology, and produce matrix as long as they are surrounded by fibrin gel in vitro.15 Choi et al used a fibrin gel–type autologous chondrocyte (Chondron) implantation for human chondral knee defects during several years without using periosteum or membrane. They concluded that this method appeared to be safe and effective for both decreasing pain and improving knee function.16 Fibrin glues (FGs) are used extensively to secure other tissue-engineered cartilage in clinical settings. FG has also been used in combination with autologous chondrocytes for the treatment of deep chondral defects in humans. When combined with commercial FG (Tissucol; Baxter, Deerfield, IL), autologous chondrocytes showed better clinical outcomes during the treatment of deep chondral defects compared with abrasive techniques after 1 year in terms of subjective scores.17 Alternatively, minced cartilage in combination with FG known as DeNovo NT (natural tissue) grafts (Zimmer, Warsaw, IN; ISTO Technologies, St. Louis, MO) is currently under clinical investigation, where cartilage pieces obtained from juvenile allograft donor joint are aseptically minced and mixed intraoperatively with FG and then implanted in the prepared lesions.18 Juvenile cartilage is chosen depending on the assumption that it would have higher anabolic capability and better expandability. Disease transmission and limited supply are the drawbacks of this technique. A similar product known as DeNovo ET (engineered tissue) graft (ISTO Technologies) is based on the use of juvenile allogeneic cartilage cells. These cells produce in vitro a hyaline-like, scaffold-free, disc-shaped graft that is implantable in a single-stage procedure.19 The product is implanted into the prepared lesion site and secured via FG. Small cartilage pieces are placed in the chondral defect with the DeNovo NT technique, whereas DeNovo ET uses individual allogenic chondrocytes to form a hyaline-like cartilage disk in vitro, which is then implanted into the cartilage defect.
Collagen
Collagen gels have been evaluated for the treatment of cartilage lesions in animal studies. Promising histological results at 24 weeks have been reported in the treatment of osteochondral defects filled with chondrocytes suspended in a type I collagen gel in the knee joint of full-grown rabbits. However, these gel types showed poor integration with the surrounding host cartilage and a lack of regeneration of a proper subchondral base.20,21 Recently, predifferentiated MSCs embedded in a collagen I hydrogel were used for the treatment of a chronic osteochondral defect in an ovine stifle joint. This repair strategy showed no signs of degradation after 1 year in vivo. In addition, these gels led to partially superior histological results compared with articular chondrocytes. A similar approach was recently performed in two patients, indicating the feasibility of this approach.14 Bovine collagen I in combination with bioadhesive is currently used clinically and is known as NeoCart; this material is produced by Histogenics (Waltham, MA). The harvested cartilage samples are processed and chondrocytes grown into collagen; the construct is then applied to the lesion and secured by bioadhesive.22 Another similar product known as CaReS has been introduced by Arthro Kinetics (Esslingen, Germany) and is also available for clinical application. CaReS utilizes rat tail collagen I, instead of bovine collagen (NeoCart). VeriCart, another product of Histogenics, has been introduced to the market as an adjuvant to microfracture. VeriCart uses a double-structured collagen scaffold.
Ochi et al used autologous chondrocytes, cultured in atelocollagen gel, for the treatment of full-thickness defects of cartilage in human knees.23,24 Atelocollagen, from which telopeptides have been removed, was chosen because the antigenic determinants on the peptide chains of type I collagen reside mainly in the telopeptide regions.25,26 In vitro and in vivo experimental results supported the hypothesis that transplanting chondrocytes cultured in atelocollagen gel would be effective in repairing articular cartilage defects, not only in animals but also in humans, by maintaining the chondrocyte phenotype, reducing the risk of leakage, and distributing grafted cells evenly throughout the grafted site.27–29 It was shown that this technique promoted the restoration of the articular cartilage in the knee.24
Gelatin
Allogeneic bone matrix gelatin (BMG) is prepared through the process of defatting, demineralization, and extraction to remove 95% of the noncollagen proteins which would eliminate antigenic materials inside the BMG, rendering it weakly immunogenic and more biocompatible with the host.30–32 It was suggested that BMG could induce differentiation of mesenchymal cells into chondroblasts in vitro and form hyaline-like cartilage in osteochondral defects in vivo.33–35 Considering the large supply of banked bone allografts and relatively convenient preparation, allogeneic cancellous BMG should be considered as a promising scaffold for cartilage tissue engineering.36 Human data concerning the use of gelatin for cartilage repair are currently not available.
Carbohydrate-Based Scaffolds
Agarose
Promising macroscopic and histological results have been reported with the use of agarose as a scaffold for chondrocytes in the treatment of osteochondral defects in 6-week-old rabbits.37 An increase in proteoglycan and type II collagen synthesis by the transplanted cells was seen, and the implant merged well with the sides of the lesion. The best results were obtained from 18 months on. However, difficulties have been encountered with the use of agarose gels because of their consistency and possible immunological reactions against these matrix molecules. Currently, no human data are available.
Poly-L-Lactic Acid and Polyglycolic Acid
Neocartilage was formed both in vitro and in vivo (subcutaneously in nude mice) when chondrocytes and polyglycolic acid (PGA) or poly-L-lactic acid (PLLA) were used.38,39 An overall repair frequency of 85% in osteochondral defects in the rabbit was reported, but the cartilage-like quality was variable and none of the specimens appeared normal after 1 year.40 Osteochondral defects in the trochlea of adult rabbits have also been treated with PGA-scaffolds seeded with allogenic chondrocytes.41 At 6 months, the total contents of glycosaminoglycans and type II collagen was only one-third of that in parent rabbit cartilage. In contrast, osteochondral defects created in the knee joint of goats with a scaffold (PLLA as a base material and PGA as an additive) seeded with chondrocytes were repaired with hyaline-like cartilage and good underlying bone at 16 weeks.42 However, its degradation products can eventually lead to the death of the implanted cells, mainly in PGA because of its more rapid degradation when compared with PLLA.43 These types of scaffolds have not yet been tested in humans in their original forms.
Hyaluronan
Because of its multiple functions in regulating and stabilizing the internal environment of cartilage, hyaluronan is a promising scaffold to promote cartilage repair.44 HYAFF 11 is the esterified derivative of hyaluronate, and when combined with autologous articular chondrocytes it forms Hyalograft C (Fidia Advanced Biopolymers, Abano Terme, Italy). In terms of the quality of regenerated cartilage and production of chondrocytic markers, trials with Hyalograft C (Fidia) yielded comparable results to that of ACI in animal models and in humans.44,45 The long-term clinical outcomes of Hyalograft C (Fidia) grafting in humans was similar to that of ACI as indicated by magnetic resonance imaging (MRI) and objective and subjective knee scoring systems. However, one advantage of Hyalograft (Fidia) grafting over ACI is that it can be performed using a less-complicated and minimally invasive surgical procedure.
Alginate
It has been shown that human chondrocytes keep their phenotype in alginate with neo-synthesis of an extracellular cartilage matrix and that this chondrocyte/alginate culture setup can be biologically frozen without any impairment in the total of overall aggrecan synthesis rates or its cartilage-specific aggrecan subtypes once thawed.46,47 A short-term pilot study showed that the alginate-based scaffold containing human mature allogenic chondrocytes is feasible and safe for the treatment of symptomatic cartilage defects of the knee in humans. This technique provided clinical and histologic outcomes that are equal but not superior to those of other cartilage repair techniques.48,49
Chitosan and Chitin
Chitosan is the deacetylated derivative of chitin. Chitosan alone or in combination with a wide variety of scaffolds has been used extensively in the tissue engineering of articular cartilage.50 The seeding of chondrocytes on chitosan-coated coverslips maintained the spherical chondrocyte morphology and promoted expression of collagen II and aggrecan.51 In addition, chitosan can serve as a cytocompatible space-filling scaffold that can gelate and adhere to cartilage when injected in situ in combination with chondrocytes.52 In contrast, it has been shown that the rate of cell proliferation and the production of collagen II were lower in the chitosan scaffold compared with chitosan–alginate hybrid after 2 weeks of culturing.53 These types of scaffolds have not yet been tested in humans in their original forms.
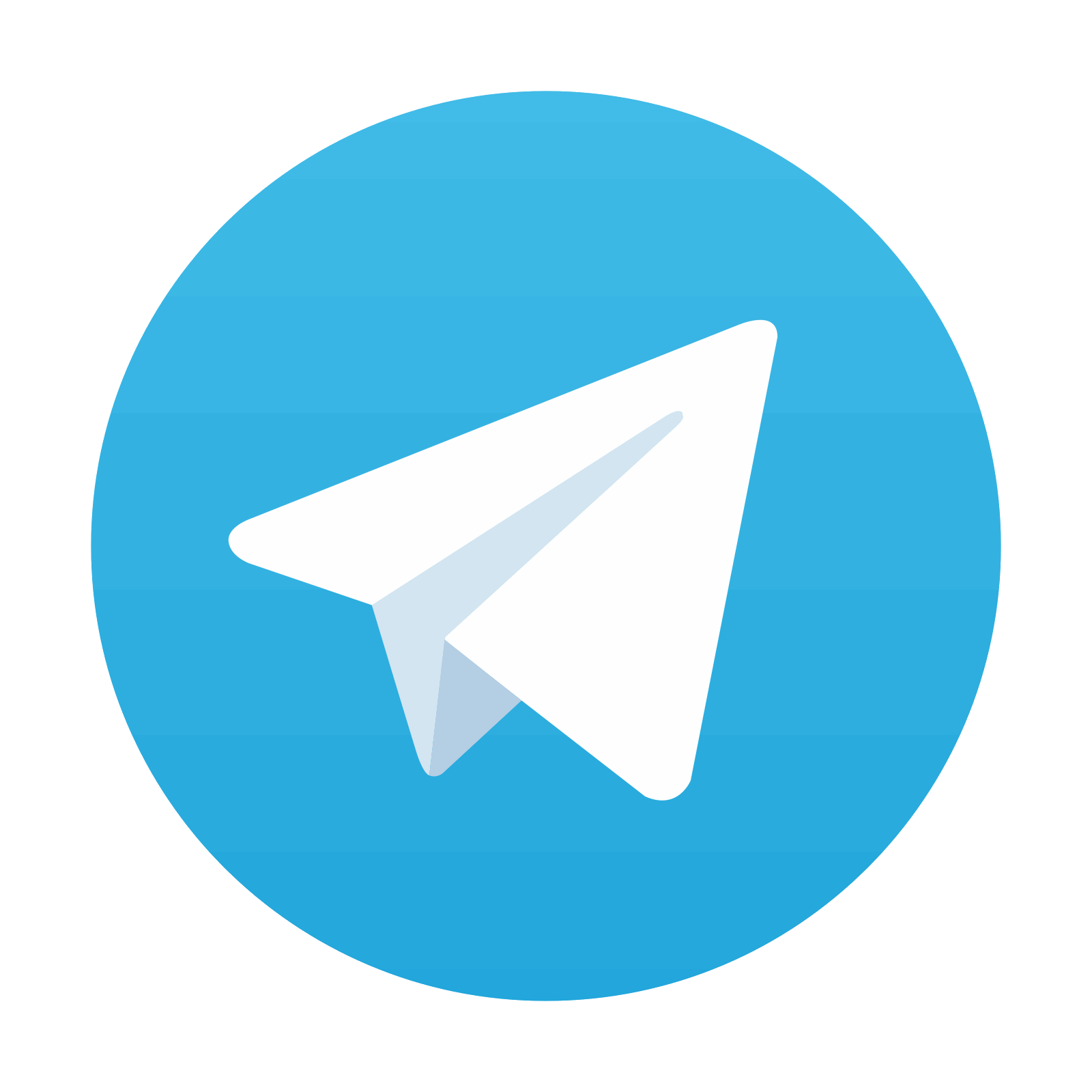
Stay updated, free articles. Join our Telegram channel

Full access? Get Clinical Tree
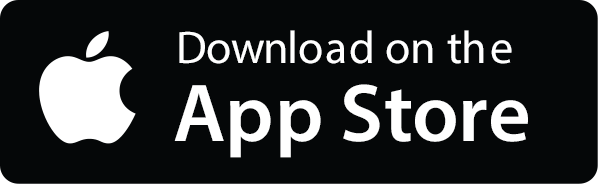
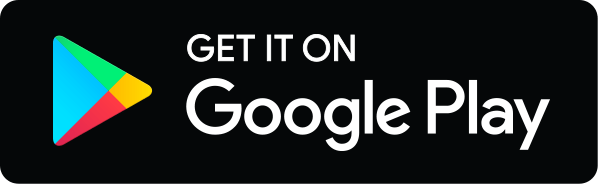
