Key points
- •
The split-belt paradigm can be used to examine motor learning or potentially as a rehabilitation intervention after stroke.
- •
After stroke, patients retain the ability to adapt their walking pattern to new constraints.
- •
Locomotor adaptation and learning may be slowed after stroke.
- •
Exaggeration of spatial gait asymmetries using the split-belt treadmill results in improved spatial gait symmetry.
Introduction
Stroke is the leading cause of long-term disability in the United States, with approximately 795,000 people experiencing a new or recurrent stroke each year. A primary concern of individuals experiencing a stroke is the ability to regain ambulatory function. Moreover, improved ambulatory function after stroke is linked to increased community participation, improved cardiovascular fitness, and decreased risk of stroke recurrence. As such, gait retraining is a major component of rehabilitation.
Gait after stroke is characterized by pronounced asymmetry. Following stroke, individuals increase reliance on the nonparetic lower extremity in static standing as well as during ambulation. This results in a shortened nonparetic swing phase and increased stance phase on the nonparetic lower extremity. The resulting spatiotemporal asymmetries (stance time, swing time, and step length asymmetries) are well documented in individuals after stroke. Step length asymmetry, in particular, has been shown to influence other gait deviations. By taking a shorter nonparetic step, the propulsive force of the paretic limb is decreased, thereby limiting forward propulsion of the body. Step length asymmetry and its associated gait deviations have been linked to decreased walking speed and efficiency, as well as decreased dynamic balance, thereby limiting safe functional ambulation. Various novel rehabilitation interventions have attempted to target these asymmetries to improve safe locomotion. In particular, several studies have utilized principles of motor learning to target specific gait deviations utilizing a split-belt treadmill.
The split-belt treadmill has 2 independent belts, one under each leg, so that subjects can walk with belts moving at the same speed, tied, or with the belts moving at different speeds, split-belt. By splitting the treadmill belt speeds in a 2 to 1 ratio, the paradigm requires both neurologically intact subjects and subjects after stroke to alter their coordination while walking. Initially, both spatial and temporal characteristics of step symmetry are altered; however, over a period of 10 to 15 minutes, this asymmetry will be reduced with the use of trial-and-error practice. When returning the treadmill to a normal walking condition or a tied-belt configuration, both neurologically intact subjects and poststroke subjects demonstrate after effects, with a reversal of the initial asymmetry induced by the split-belt treadmill configuration. The presence of this after effect indicates that the nervous system has learned and stored a new locomotor pattern. The use of trial-and-error practice, or adaptation, to a perturbing environment provides important insight into the ability of the poststroke central nervous system (CNS) to temporarily store and recall a motor memory.
Thus, the split-belt treadmill paradigm allows exploration of various aspects of motor learning, including adaptation and retention of a novel locomotor pattern, but also allows exploration of the capacity of the nervous system for error recognition and correction. Recent evidence suggests exaggeration of poststroke gait asymmetry using the split-belt treadmill can lead to after effects, resulting in a more symmetric pattern of walking on the treadmill as well as over ground. With repeated exposure to split-belt treadmill walking subjects after stroke demonstrate longer-term improvements in step length symmetry. Consequently, the split-belt treadmill can be utilized to facilitate improvements in asymmetric gait after stroke, or can be utilized as a specific probe of motor learning. This article discusses the current role of the split-belt treadmill in the examination of locomotor learning as well as a potential therapeutic tool for intervention in individuals after stroke.
Introduction
Stroke is the leading cause of long-term disability in the United States, with approximately 795,000 people experiencing a new or recurrent stroke each year. A primary concern of individuals experiencing a stroke is the ability to regain ambulatory function. Moreover, improved ambulatory function after stroke is linked to increased community participation, improved cardiovascular fitness, and decreased risk of stroke recurrence. As such, gait retraining is a major component of rehabilitation.
Gait after stroke is characterized by pronounced asymmetry. Following stroke, individuals increase reliance on the nonparetic lower extremity in static standing as well as during ambulation. This results in a shortened nonparetic swing phase and increased stance phase on the nonparetic lower extremity. The resulting spatiotemporal asymmetries (stance time, swing time, and step length asymmetries) are well documented in individuals after stroke. Step length asymmetry, in particular, has been shown to influence other gait deviations. By taking a shorter nonparetic step, the propulsive force of the paretic limb is decreased, thereby limiting forward propulsion of the body. Step length asymmetry and its associated gait deviations have been linked to decreased walking speed and efficiency, as well as decreased dynamic balance, thereby limiting safe functional ambulation. Various novel rehabilitation interventions have attempted to target these asymmetries to improve safe locomotion. In particular, several studies have utilized principles of motor learning to target specific gait deviations utilizing a split-belt treadmill.
The split-belt treadmill has 2 independent belts, one under each leg, so that subjects can walk with belts moving at the same speed, tied, or with the belts moving at different speeds, split-belt. By splitting the treadmill belt speeds in a 2 to 1 ratio, the paradigm requires both neurologically intact subjects and subjects after stroke to alter their coordination while walking. Initially, both spatial and temporal characteristics of step symmetry are altered; however, over a period of 10 to 15 minutes, this asymmetry will be reduced with the use of trial-and-error practice. When returning the treadmill to a normal walking condition or a tied-belt configuration, both neurologically intact subjects and poststroke subjects demonstrate after effects, with a reversal of the initial asymmetry induced by the split-belt treadmill configuration. The presence of this after effect indicates that the nervous system has learned and stored a new locomotor pattern. The use of trial-and-error practice, or adaptation, to a perturbing environment provides important insight into the ability of the poststroke central nervous system (CNS) to temporarily store and recall a motor memory.
Thus, the split-belt treadmill paradigm allows exploration of various aspects of motor learning, including adaptation and retention of a novel locomotor pattern, but also allows exploration of the capacity of the nervous system for error recognition and correction. Recent evidence suggests exaggeration of poststroke gait asymmetry using the split-belt treadmill can lead to after effects, resulting in a more symmetric pattern of walking on the treadmill as well as over ground. With repeated exposure to split-belt treadmill walking subjects after stroke demonstrate longer-term improvements in step length symmetry. Consequently, the split-belt treadmill can be utilized to facilitate improvements in asymmetric gait after stroke, or can be utilized as a specific probe of motor learning. This article discusses the current role of the split-belt treadmill in the examination of locomotor learning as well as a potential therapeutic tool for intervention in individuals after stroke.
Adaptation
Research employing principles of motor learning, specifically adaptation, have recently gained interest because of the ability to target specific gait abnormalities in individuals after stroke. Within these studies, adaptation may be defined as the process of modifying or adjusting an already well-learned movement or motor skill based on error feedback. This process of adaptation occurs over a period of trial-and-error practice in response to novel task demands. Given this definition, motor adaptation can be considered as 1 specific component of motor skill learning. Once fully adapted, storage of a new motor pattern within the CNS is reflected through after effects. Upon removal of the stimulus, the subject is not able to retrieve the previous motor behavior. The subject must de-adapt, during a period of continued practice without the perturbation, in order to return to his or her previous baseline motor performance.
Given that motor adaptation involves relearning an already well known movement pattern, the process strongly reflects the relearning process of subjects after stroke early within a therapeutic intervention. As a short-term learning process, adaptation has gained interest within motor learning research, particularly locomotor learning. Locomotor adaptation affords the ability to learn and unlearn a given locomotor pattern rapidly depending on the environment, allowing for flexibility and efficiency. The capacity of the nervous system to adapt to and store a new locomotor pattern that approximates an already stored walking pattern may provide insight into the ability of the damaged nervous system to regain a more normal walking pattern.
When subjects are asked to walk on a split-belt treadmill, participants with chronic stroke and neurologically intact participants demonstrate an immediate reaction in which the leg on the slow belt will immediately spend more time in stance, and the fast leg will spend less time in stance to accommodate the difference in belt speeds. When the belts are returned to normal conditions, tied belts, subjects immediately return stance times to the baseline walking pattern. Additional intralimb characteristics, including swing time, stride length, and intralimb joint kinematics, appear to demonstrate a similar pattern of reactive change to the split-belt treadmill condition with absence of adaptation and after effects. These reactive changes require utilization of peripheral feedback and do not appear to rely on adaptive processes as indicated by lack of after effects. In contrast, interlimb gait parameters of step length, double support time, and center of oscillation have been found to change slowly during locomotor adaptation and demonstrate appreciable after effects. Specifically, step length asymmetry exaggerated by the split-belt paradigm requires a period of trial-and-error practice in order to reduce this asymmetry toward baseline. During the split-belt condition, an adaptive response occurs via feedforward changes in interlimb coordination in order to allow a return to normal step symmetry, despite the treadmill belts going 2 different speeds. When the belts are returned to tied, neurologically intact subjects demonstrate step asymmetry in the opposite direction, while chronic stroke subjects may demonstrate an improved symmetry relative to baseline.
In patients after stroke, improved step length symmetry is only achieved through exaggeration of the subjects’ initial step length asymmetry. Specifically, an individual with stroke who ambulates with a longer paretic step length relative to the nonparetic step will walk on the split-belt treadmill with the paretic leg on the slow belt. When the treadmill is set to a 2 to 1 speed ratio, split-belt, the subject will initially walk with an even longer paretic step than demonstrated with baseline tied belt walking, thus exaggerating his or her step length asymmetry or error. Over a period of 10 to 15 minutes, individuals after stroke are able to reduce this asymmetry. When the belts are returned to the tied belt condition, after effects are evident, with subjects maintaining a more symmetric walking pattern. The improved gait pattern also transfers to overground walking. As in a true adaptation paradigm, however, the improved symmetry is short-lived, and subjects return to baseline asymmetry within several minutes. Despite the brevity of improved symmetry, the current findings demonstrate that individuals after stroke maintain the ability to ambulate with a more symmetric walking pattern.
The studies presented demonstrate that individuals after stroke retain the basic capacity to adapt their walking pattern to novel environmental conditions. Recent locomotor adaptation studies also indicate, however, that the rate of adaptation is slowed after stroke. Rate deficits appear to be specific to spatial rather than temporal gait parameters in those after stroke. Differences in adaptation rates of temporal versus spatial characteristics of gait have been previously demonstrated in neurologically intact subjects. Specifically, Malone and Bastian demonstrated that neurologically intact subjects walking on a split-belt treadmill were able to adapt limb phasing at a rate twice that of step length. In addition, temporal characteristics of gait appear to be much more resistant to manipulations of practice structure as well as developmental stage. Young children demonstrate rates of adaption of center of oscillation and step length symmetry that improve with age, while rates of limb phase adaptation are similar regardless of age. These differences in temporal versus spatial gait characteristics have been postulated to be caused by differing sites of neural control, with temporal characteristics thought to be under subcortical control.
Recent exploration of locomotor adaptation in neurologically intact subjects utilizing the split-belt treadmill highlight additional constructs that may be utilized to enhance the rate of adaptation of spatial parameters after stroke. Providing visual feedback in order to allow conscious correction of step symmetry during split-belt adaptation in neurologically intact subjects resulted in an increased rate of adaptation, however also increased the rate of de-adaptation, thereby limiting the potential for increased retention of the split-belt pattern. Performance of a secondary task while walking on the split-belt treadmill, however, resulted in a decreased rate of adaptation and de-adaptation, thereby allowing enhanced retention of the split-belt pattern. It is currently unknown whether this paradigm could be utilized in those after stroke given that individuals after stroke demonstrate difficulties with interference between cognitive tasks and motor control activities including gait. Further research identifying specific task parameters that may improve or limit adaptation and retention of a novel locomotor pattern are required in order to develop targeted and effective interventions in those after stroke.
Although individuals with stroke retain the basic ability to adapt to the split-belt treadmill, recent evidence indicates that damage to the cerebellum, whether due to stroke or other causes, interferes with this adaptive capacity. When exposed to the split-belt walking condition, patients with cerebellar lesions are able to make reactive, feedback-driven changes to intralimb characteristics including stride length, stance, and swing time. Subjects with cerebellar damage, however, are unable to utilize trial-and-error practice to adapt characteristics of interlimb coordination that require feedforward, predictive changes in gait. Similar to previously cited studies, Morton and Bastian indicated that differing levels of locomotor adaptability, feedforward versus reactive, may be under separate neural control. Given that the control of motor learning is suggested to occur in multiple brain areas that may be affected by stroke, it is plausible that specific deficits in locomotor adaptation may be a result of deficits in acquisition of a motor skill within particular damaged cortical areas.
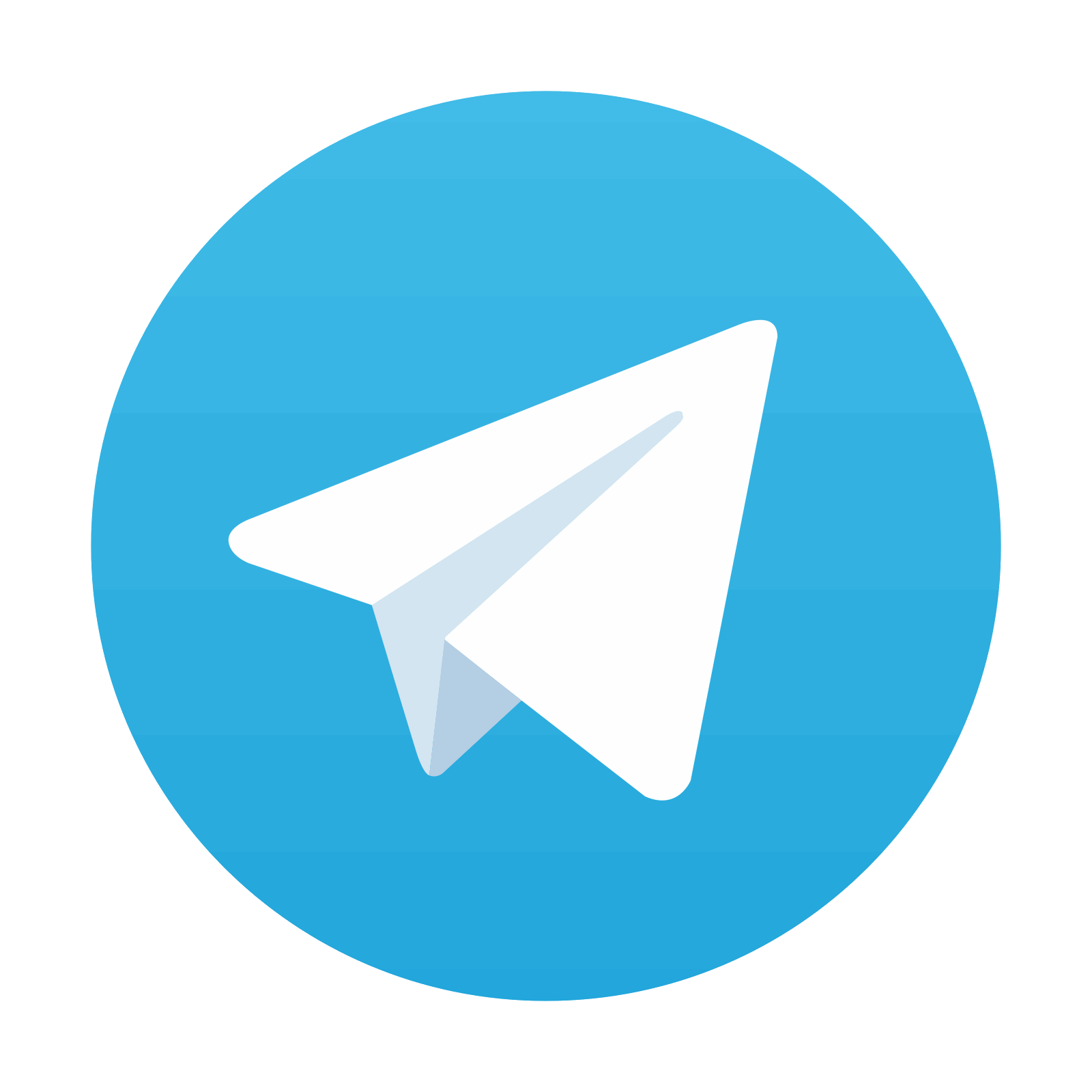
Stay updated, free articles. Join our Telegram channel

Full access? Get Clinical Tree
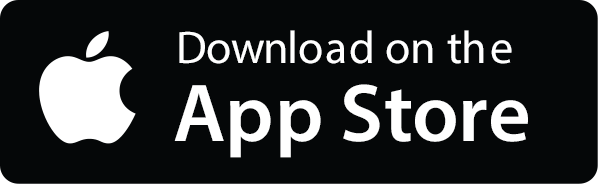
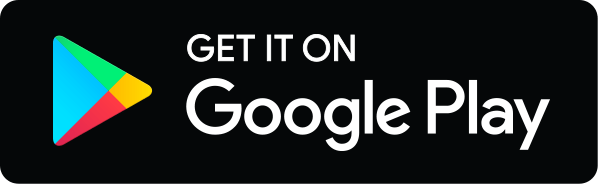