Understanding poststroke upper limb impairment is essential to planning therapeutic efforts to restore function. However, determining which upper limb impairment to treat and how is complex because the impairments are not static and multiple impairments may be present simultaneously. How impairments contribute to upper limb dysfunction may be understood by examining them from the perspective of their functional consequences. There are 3 main functional consequences of impairments on upper limb function: (1) learned nonuse, (2) learned bad use, and (3) forgetting as determined by behavioral analysis of tasks. The impairments that contribute to each of these functional limitations are described.
Key points
- •
Weakness or paresis is the key impairment early on that leads to learned nonuse. Sensory impairment, immobility, and chronic pain may further contribute to learned nonuse.
- •
Spasticity, spastic cocontraction, and abnormal motor synergies occur as recovery proceeds and may lead to abnormal compensatory movements, which if repeated and reinforced lead to learned bad use.
- •
Impairment in sensorimotor adaptation can lead to transient retention of new skills despite extensive practice; this is referred to as forgetting.
The nature of upper limb motor impairment
According to the International Classification of Functioning, Disability and Health (ICF) model, impairments may be described as (1) impairments of body function such as a significant deviation or loss in neuromusculoskeletal and movement-related function related to joint mobility, muscle power, muscle tone, and/or involuntary movements or (2) impairment of body structures such as a significant deviation in structure of the nervous system or structures related to movement, for example, the arm and/or hand. A stroke may lead to both types of impairments. Upper limb impairments after stroke cause the functional limitations in using the affected upper limb after stroke, therefore a clear understanding of the underlying impairments is necessary for restoration of upper limb function. However, understanding upper limb impairments in any given patient is complex for two reasons. (1) The impairments are not static, that is, as motor recovery proceeds, the type and nature of the impairments may change; therefore the treatment needs to evolve to target the impairment(s) contributing to dysfunction at a given point in time. (2) Multiple impairments may be present simultaneously, that is, a patient may present with weakness of the arm and hand immediately after a stroke, which may not have resolved when spasticity sets in a few weeks or months later; hence there may be a layering of impairments over time making it difficult to decide what to treat first. It is useful to review the progression of motor recovery as described by Twitchell and Brunnstrom to understand how impairments may be layered over time ( Fig. 1 ).
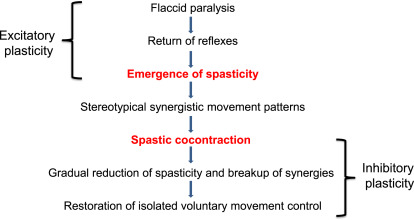
The nature of upper limb motor impairment
According to the International Classification of Functioning, Disability and Health (ICF) model, impairments may be described as (1) impairments of body function such as a significant deviation or loss in neuromusculoskeletal and movement-related function related to joint mobility, muscle power, muscle tone, and/or involuntary movements or (2) impairment of body structures such as a significant deviation in structure of the nervous system or structures related to movement, for example, the arm and/or hand. A stroke may lead to both types of impairments. Upper limb impairments after stroke cause the functional limitations in using the affected upper limb after stroke, therefore a clear understanding of the underlying impairments is necessary for restoration of upper limb function. However, understanding upper limb impairments in any given patient is complex for two reasons. (1) The impairments are not static, that is, as motor recovery proceeds, the type and nature of the impairments may change; therefore the treatment needs to evolve to target the impairment(s) contributing to dysfunction at a given point in time. (2) Multiple impairments may be present simultaneously, that is, a patient may present with weakness of the arm and hand immediately after a stroke, which may not have resolved when spasticity sets in a few weeks or months later; hence there may be a layering of impairments over time making it difficult to decide what to treat first. It is useful to review the progression of motor recovery as described by Twitchell and Brunnstrom to understand how impairments may be layered over time ( Fig. 1 ).
Understanding motor impairment from a functional perspective
The most useful way to understand how impairments contribute to upper limb dysfunction may be to examine them from the perspective of their functional consequences. There are three main functional consequences of stroke on the upper limb: (1) learned nonuse, (2) learned bad use, and (3) forgetting as determined by behavioral analysis of a task such as reaching for a food pellet and bringing it to the mouth in animal models of stroke. These consequences are equally valid for human behavior. Each of the functional consequences and the underlying impairments are elaborated in the following sections.
Learned nonuse
Initially after a stroke, individuals may not use their affected upper limb, eventually leading to learned nonuse. Nonuse can result from several impairments. Initially nonuse may occur because of weakness/paralysis or sensory loss. However, as time progresses, nonuse may become habitual and the limb may not be incorporated into functional activities, even though the individual can move it. Now it becomes a learned behavior and is referred to as learned nonuse.
Weakness or paralysis is the predominant impairment that contributes to dysfunction after stroke ; it is a direct consequence of the lack of signal transmission from the motor cortex, which generates the movement impulse, to the spinal cord, which executes the movement via signals to muscles. This lack of transmission results in delayed initiation and termination of muscle contraction, and slowness in developing force, manifested as an inability to move or move quickly with negative functional consequences. Abnormally increased electromyographic (EMG)-force slopes are seen on the affected side compared with the contralateral side as well as compared with neurologically intact subjects, suggesting that greater EMG activity is necessary to generate a given force in patients with stroke. This requirement is thought to result from a combination of abnormal firing rate patterns and changes in motor unit control. Weakness may affect all muscle groups of the upper limb, or may be selective, affecting some muscle groups more than the others. Large intersubject differences exist in the pattern of muscle weakness across muscle groups, but research has shown that no consistent proximal to distal gradient or greater extensor relative to flexor weakness exists. The absolute strength in any particular muscle group has not been shown to predict function, whereas the rate of change of force development in wrist extensor and handgrip strength are good predictors of upper limb function. Isolated motor deficits in a single arm can occur after a stroke without other cranial or sensory dysfunction; these are rare and easily misdiagnosed, requiring a high index of suspicion and assessment of risk factors for stroke. In cases in which trauma accompanies the vascular lesion, the pattern of weakness may need to be examined carefully to rule out spinal cord injury or peripheral nerve injury. Weakness leads to immobility, which can initiate a cascade of problems that can further contribute to motor impairment ( Fig. 2 ), as described in the following discussion.
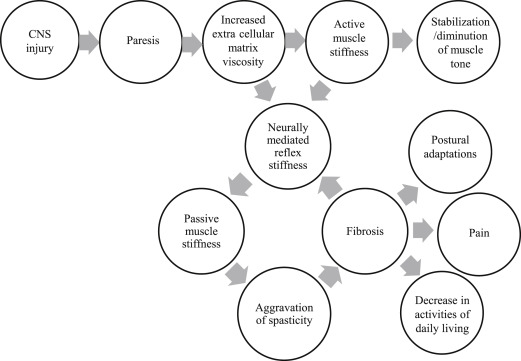
Sensory loss across tactile, proprioceptive, and/or higher-order sensory modalities such as deficits in 2-point discrimination, stereognosis, and graphesthesia are common after stroke and may be associated with the degree of weakness and the degree of stroke severity, as well as mobility, independence in activities of daily living, and recovery. Sensory impairments without motor weakness may also occur from specific lesions in the parietal cortex. In studies that compared the ability of hemiparetic and healthy subjects to produce symmetric forces with both upper limbs, it was found that joint maximum voluntary forces and proprioceptive impairments in the affected limb predicted the errors in force matching. These results suggest that sensory impairments may lead to inaccurate motor output, even though motor capacities were adequate to perform the task. In fact the term learned nonuse was coined from observations in deafferented monkeys that could move but did not do so voluntarily. Chronic loss of sensation may contribute to motor impairment because of inaccurate internal representations of the task and/or inability to control the motor output appropriately owing to lack of feedback about the consequences of the motor action.
Weakness leads to immobility, which can be considered a functional impairment according to the ICF classification. Immobility in turn can begin a vicious circle of problems including peripheral soft tissue changes that reduce tissue compliance, potentiation of reflex mechanisms, and spasticity, eventually leading to muscle fibrosis and contributing to abnormal limb posturing, pain, and decreased function (see Fig. 2 ). Spasticity is now thought to arise as a consequence of contractures rather than being a cause of contractures. Hence early interventions to reduce immobility and preserve range of motion either passively or actively despite paresis, and prevent contractures may be critical to prevent spasticity and its ensuing complications. Passive tissue restraint and agonist weakness, rather than antagonist restraint, have been shown to be the most common contributors to decreased active range of motion. Immobility also leads to changes in bone mineral density with increased risk of developing osteoporosis on the paralyzed side particularly in the upper limbs. In fact, fractures are common on the paretic side after stroke. Practitioners need to pay more attention to changes in bone mineral density after stroke and take active measures to prevent problems arising from immobility.
Motor and sensory impairments and immobility are associated with an increased risk for stroke-related pain. Stiffness in the connective tissue of the immobilized limb may stimulate free nerve endings and proprioceptors, such as Pacini and Ruffini corpuscles in the tissue producing pain. Shoulder pain on the paretic side is common after stroke and is strongly associated with abnormal shoulder joint examination, ipsilateral sensory abnormalities, and arm weakness. Deafferentation and sensory loss may also lead to the development of neuronal hypersensitivity, and eventually, chronic central pain which is often difficult to treat. Pain can lead to learned nonuse, which may persist even after the pain has resolved.
Learned bad use
When the paretic limb is forced to move, weakness, sensory impairments, and pain can prevent normal movement; instead compensatory strategies are used to complete the task or tasks. Furthermore, stiffness and contractures resulting from immobility and the development of spasticity and abnormal motor synergies can contribute to compensatory movements. The use of compensatory strategies has been well described for human reaching and grasping after stroke. Patients with stroke use trunk flexion rather than elbow extension to reach for objects, forearm pronation and wrist flexion rather than neutral forearm position and wrist extension to orient the hand for grasping, and metacarpophalangeal joint flexion rather than proximal interphalangeal joint flexion to grasp objects. Although the use of compensatory strategies may lead to initial success in completing a task, over time success is reduced because of poor accuracy, which increases the probability of failure. Reinforcement of the abnormal strategy by occasional successes can lead to it becoming a bad habit over time, and performance declines despite extended training because the abnormal behavior is repeated and reinforced at the cost of the correct pattern of behavior. Thus in the absence of appropriate feedback and correction of the abnormal motor behavior, learned bad use develops. When the focus of training is to reduce compensatory behaviors, for example, when the trunk is restrained during reach practice, the typical use of a more normal pattern of reaching by extending the elbow is restored along with a reduction in overall impairment.
Spasticity and incoordination because of abnormal motor synergies can lead to the development of learned bad use. Spasticity is defined as a motor disorder characterized by a velocity-dependent increase in muscle tone with exaggerated tendon jerks, resulting from hyperexcitability of the stretch reflex, as one component of the upper motor neuron syndrome. The prevalence of spasticity increases with time since stroke and is related to the secondary effects of weakness and immobility on skeletal muscles. Initially, spasticity is considered a positive development because it suggests that the nervous system is beginning to initiate repair mechanisms to restore muscle tone and movement. Indeed, patients who demonstrate spasticity are further along in their recovery than individuals who are more flaccid. However, when the threshold for reflex activity continues to reduce because of progressive reorganization of the supraspinal descending drive to the spinal cord, peripheral structures of the muscle, muscle spindles, and fascia are further shortened and spasticity evolves into stretch-sensitive forms such as spastic cocontraction. Spastic cocontraction refers to inappropriate antagonist recruitment triggered by volitional command. Clinically, spastic cocontraction leads to involuntary movement in the opposite direction of the intended voluntary movement and contributes to impairment in active function. The degree of spastic cocontraction has been shown to be positively related to the Fugl-Meyer score, suggesting that individuals with spastic cocontraction, although significantly impaired, are further along in their recovery process. Spasticity and spastic cocontraction may, however, lead to learned bad use. Reduction in spasticity with botulinum toxin injections has been shown to improve kinematic parameters such as velocity and smoothness, without significant changes in clinical outcomes such as hand function. It is possible that weakness and atrophy produced by the injections negates the benefit of improvement in the form of the movement, or that functional improvement requires aggressive concurrent therapy.
Abnormal motor synergies have been well described after stroke. For example, during reaching, the shoulder must flex while the elbow extends. However, in patients with stroke, attempts at voluntary forward reaching often result in shoulder abduction and elbow flexion because of the constraining effect of abnormal descending motor commands. The abnormal muscle synergies were not related to proximal weakness or abnormalities in the elbow flexor-extensor strength balance. More recently, it has been found that the common drive to muscles that are functionally coupled during reaching in healthy individuals, for example, the anterior deltoid and triceps brachii, is weakened after stroke likely because of interruption of information flow in the corticospinal pathway. Abnormal muscle synergies have been shown to be independent of weakness, slowness of muscle activation, excessive cocontraction, and spasticity, and instead reflect a loss of skill in generating spatial and temporal muscle activation patterns that conform with environmental demands. Training strategies that promote movement outside of the abnormal motor patterns may be needed to retrain more normal movement patterns.
Forgetting
Once a motor skill is attained through training, there is an expectation that it will be retained forever, despite intervals of no training (in the same way that one never forgets how to ride a bicycle). However, rats with motor cortex injury show a decline in performance during intervals of no training and additional training is required to get performance back to pretraining levels. Breaks in rehabilitation similarly lead to forgetting of upper extremity motor skills in humans after stroke. Thus new skills, although reasonably stable in healthy individuals, are more transient after stroke. Skill learning requires that at least three independent processes occur across multiple timescales. First, precise task-specific sensorimotor mappings occur through trial-and-error adaptation during practice with appropriate error sensing. Adaptation is a fast learning process, which leads to a rapid reduction in movement error, and typically takes only a few trials; however it is easily forgotten. The second process is repetition, which alters movement biases depending on what is repeated. Repetition leads to a slow tuning of directional biases toward the repeated movement. A task can be repeated with or without adaptation to error and does not require error sensing. The third process is reinforcement, whereby movements are rewarded intrinsically or extrinsically and reward leads to faster relearning or savings on subsequent attempts. Although these three processes occur independently, it has been shown that learning is most successful when sensorimotor adaptation is combined effectively with repetition ( Fig. 3 ). For instance, appropriate sensorimotor mappings learned through adaptation must be repeated over time for sustained and appropriate changes in skill to occur. Impaired sensorimotor adaptation and lack of opportunities for long-term practice can lead to unlearning or forgetting after stroke.
