- •
Ultrasound can be used to scan and evaluate most musculoskeletal tissues.
- •
Articular and periarticular structures may only be visualized if they fall within an acoustic window.
- •
Transducers, presets, and settings should be selected according to the chosen joint component or tissue.
Human fetuses have approximately 270 bones, but by the time of birth, many of them have fused together, leaving the adult with 206 bones. The average number of joints in the human body is less exact. If we aim to count every joint, including large synovial joints such as the hip, sutures connecting the bones of the skull, or gomphoses connecting the roots of teeth to their sockets, we must define a single joint . Human joints are complex structures that form at the junction of two or more bones and possess various functions and limitations. They play a dual role in facilitating motion, allowing the human body to interact with its surroundings and providing mechanical support. Joints are usually classified according to their function or structure, with significant overlap between the two groupings.
Classification of Joints
Structural classification names and categorizes joints according to the type of connection between the bones and differentiates fibrous joints, joined by fibrous connective tissue; cartilaginous joints, joined by hyaline cartilage; and synovial joints, enclosed by joint capsules that are not joined directly. Functional classification is based on the degree of mobility allowed by the joint. Tables 5-1, 5-2, and 5-3 show a composite classification system based on the function and shape of joints.
Anatomic Type | Description |
---|---|
|
|
|
|
|
|
∗ In diarthrosis, a freely movable synovial joint allows movement in one or more planes.
Anatomic Type | Description |
---|---|
| Cartilage allows some motion; no synovial lining |
|
|
∗ In amphiarthrosis, a semimovable, nonsynovial joint has some movement at the cartilage-bone interface.
Type | Description |
---|---|
| Connects skull bones |
| Connects the roots of teeth to their sockets |
| Inferior tibiofibular joint |
∗ In synarthrosis, a nonmovable, nonsynovial joint is formed by connective tissue between bones.
Despite widespread use, functional classification fails to take into account small but vital movements in other planes (e.g., knee rotation at the end of flexion) or sliding movements. Joints may also be grouped according to anatomic regions or on the basis of their biomechanical properties. Joints can be divided into simple joints formed by two articular surfaces (e.g., metacarpophalangeal [MCP] joint, proximal interphalangeal [PIP] joint); compound joints formed by three or more articular surfaces (e.g., radiocarpal joints), depending on the number of bones involved; and complex and combination joints featuring two or more articular surfaces with additional articular structures such as articular disks or menisci (e.g., knee joint). Anatomic regions include articulations of the hand, elbow joints, wrist joints, axillary articulations, sternoclavicular joints, vertebral articulations, temporomandibular joints [TMJs], sacroiliac joints, hip joints, knee joints, and articulations of the foot.
Peripheral joints can also be grouped according to size. This classification system is useful for selecting the ideal transducer and technical parameters for sonography and for arthrocentesis and joint injections. Size-based classification distinguishes between small joints (e.g., carpometacarpal [CMC], MCP, metatarsophalangeal [MTP], PIP, distal interphalangeal [DIP],TMJ), medium-sized joint (e.g., wrist, elbow, ankle, hindfoot, midfoot), and large joints (e.g., knee, shoulder, hip).
Musculoskeletal Ultrasound
Musculoskeletal ultrasound has a history spanning more than 50 years. In 1942, Karl Theodore Dussik because the first physician to use ultrasound as a medical diagnostic tool. In his 1958 paper on this subject, he examined different articular and periarticular tissues, such as skin, adipose tissue, muscle, tendon, articular capsule, articular cartilage, and bone. He measured the acoustic attenuation constants of these tissues, described fiber anisotropy, and proposed several pathologic influences on sound attenuation. He stated that hydration decreases and dehydration increases the attenuation constants; cellular filtration in general may increase values, but fatty infiltration decreases the attenuation constants. Cirrhotic changes or collagen fiber increases the attenuation constants. Aging of tissues tends to increase attenuation values due to the gradually increased relative amount of intercellular substances and the progressive loss of fluid.
Ultrasound reflects off acoustic interfaces, and the amount of reflection depends on the difference between the acoustic impedances of the two media forming the interface (less than 1% difference is enough for reflection to occur). In medical practice, air or other gas and bone are the two extremes whose properties radically differ from those of soft tissue or fluid.
Image Acquisition
Images should be obtained in the longitudinal and transverse planes to provide the most information and enable proper localization and measurement of components. Not all parts of a joint may be visible through such an acoustic window, and not every joint can be investigated from all aspects; for example, the hip joint cannot be examined from the direction of the trunk. The term acoustic window refers to the part of the joint that allows reflection of emitted ultrasound waves from anatomic structures. If a joint or part of a joint is covered by bone, the wave will reflect from the bone (e.g., patellofemoral joint surface), preventing visualization of the underlying joint.
Another major condition for depicting joint structures by ultrasound is the requirement of interfaces between tissues and tissue components, which are caused by inherited differences in the acoustic impedance. In privileged cases, a multiplanar view allows investigation of a single joint from different aspects to the full extent of the acoustic window. It is frequently useful to scan the contralateral side of paired structures to identify individual variations of normal joints.
Anisotropy denotes directional dependence, and isotropy refers to homogeneity regardless of direction. Some periarticular soft tissue structures may not be visualized to the same extent as others because they are not parallel to the surface or because the transducer cannot be manipulated so that the ultrasound beam is perpendicular to the selected structure (e.g., posterior cruciate ligament). Superficial structures usually can be visualized with higher resolution compared with deeper structures because of the higher frequency of the ultrasound wave. Different body builds and tissue compositions can lead to significant differences in ultrasound examination results for the healthy joints of various patients. The examiner must remember that the acquired image is a two-dimensional depiction of a three-dimensional tissue volume. A certain amount of information is always lost in the process, depending on the slice thickness. Lower frequencies denote thicker slices on most available ultrasound machines.
Image Interpretation
Interpretation of sonograms follows a distinct pattern. Starting from the identification of basic attributes, the sonographer progresses to more advanced levels of recognition, incorporating data available from other scientific fields (e.g., anatomy, physics, acoustics) ( Table 5-4 ).
|
Transducers and Presets
Presets are a group of physical characteristics or software parameters adapted to certain types of examination. They are originally part of the software system of individual ultrasound machines and may be modified manually to suit the examiner. Choosing the appropriate transducer and preset is a crucial step before the acquisition of sonographic images.
Transducer sizes correlate with various ranges of frequency. The surface area of a transducer in contact with the patient is referred to as the footprint size . Smaller footprint size and a higher frequency yield higher resolution and better-quality images, but penetration is reduced, because the available acoustic energy required for penetrating deeper structures decreases at higher frequencies. Depending on the desired area or region, different transducers may be selected ( Fig. 5-1 ). Superficial structures, such as the skin, subcutaneous tissue, certain tendons, and small joints, usually are scanned with smaller transducers at high frequencies, and large, low-frequency transducers are selected for deeper structures, such as muscles and large joints. The transducer can be moved in several ways to facilitate better visualization ( Table 5-5 ).

|
Ultrasound Examinations
Joint Components
Ultrasound examinations in rheumatology tend to focus mainly on diarthroses, also known as synovial joints. Synovial joints are composed of several tissues. Some are obligatory, such as the articular bone surface, hyaline cartilage, capsule, and synovial membrane. Joints may contain various nonobligatory components, including fat pads, fibrocartilage structures, and tendons. Because of their three-dimensional nature, structure, and reflectivity, obligatory joint components can be evaluated only partly by ultrasound, and some are not detectable under normal conditions (e.g., synovial membrane, lymphatic network) ( Table 5-6 , Figs. 5-2 through 5-4 ). Nonobligatory components are only partly detectable by ultrasound ( Table 5-7 ). Dynamic examination is especially useful for evaluating nonobligatory components (e.g., labrum, ligament), because they often are revealed to a greater extent when the joint is in motion. Fluid and tendons also are more easily detected during motion.
Ultrasound Evaluation | Obligatory Components |
---|---|
Completely detectable by ultrasound | None |
Partly detectable by ultrasound |
|
Not detectable by ultrasound |
|



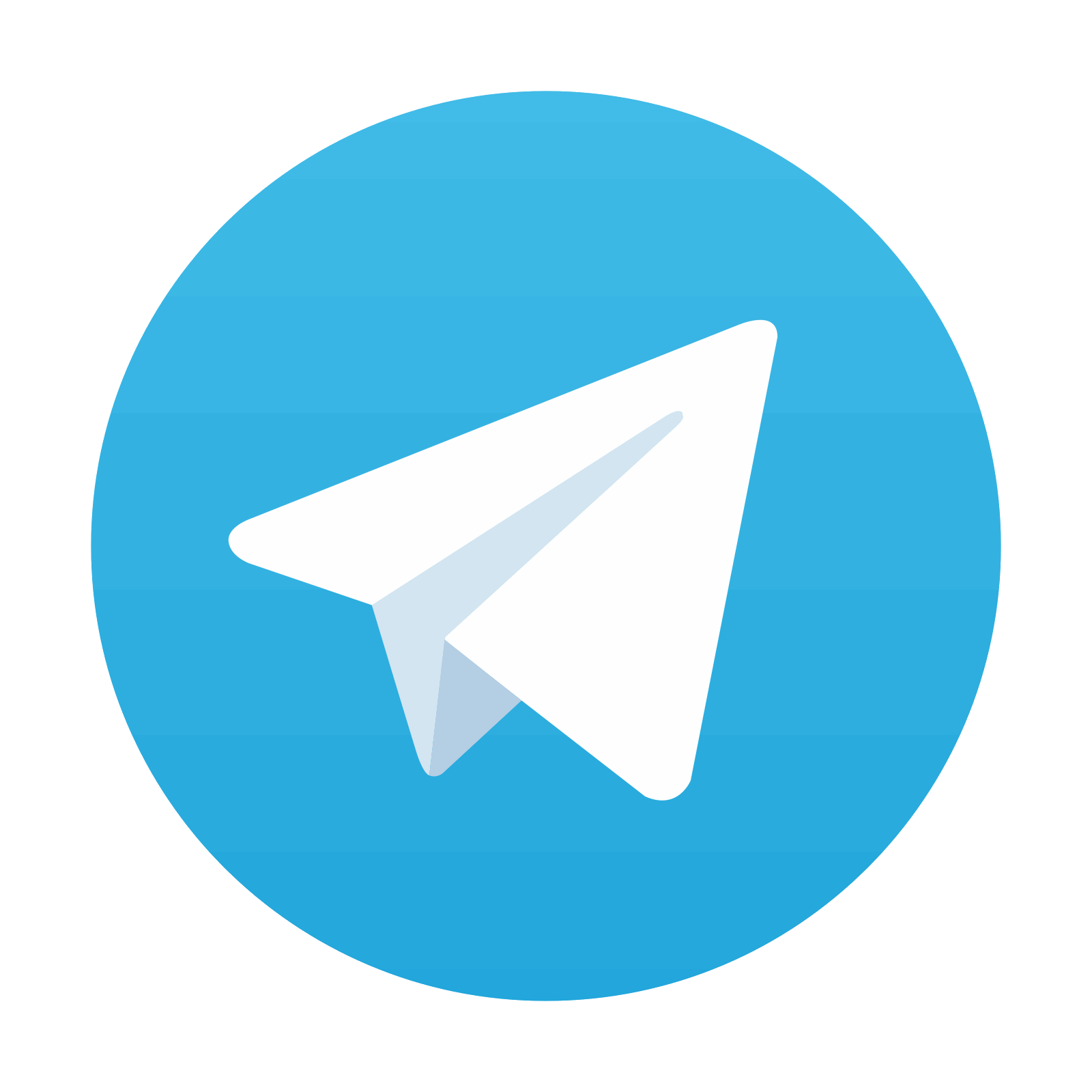
Stay updated, free articles. Join our Telegram channel

Full access? Get Clinical Tree
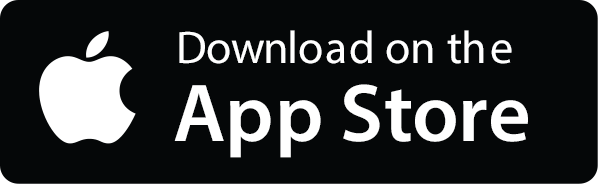
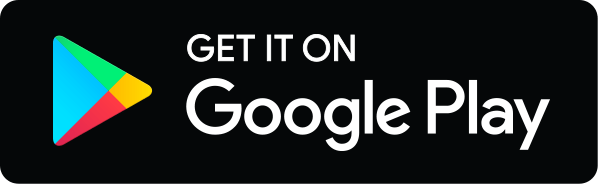
