After a concussion, a series of complex, overlapping, and disruptive events occur within the brain, leading to symptoms and behavioral dysfunction. These events include ionic shifts, damaged neuronal architecture, higher concentrations of inflammatory chemicals, increased excitatory neurotransmitter release, and cerebral blood flow disruptions, leading to a neuronal crisis. This review summarizes the translational aspects of the pathophysiologic cascade of postconcussion events, focusing on the role of excitatory neurotransmitters and ionic fluxes, and their role in neuronal disruption. We review the relationship between physiologic disruption and behavioral alterations, and proposed treatments aimed to restore the balance of disrupted processes.
Key points
- •
After a concussive event, a complex set of processes occur that disrupt neuronal functioning and result in different signs and symptoms.
- •
Pathophysiologic processes after concussion include ionic shifts, neuronal architecture damage, increased neuroinflammation, increased release of excitatory neurotransmitters, and altered cerebral blood flow control.
- •
The inability to deliver energy to the brain after a concussion, paired with a high demand for energy to restore damaged functions, results in an energy mismatch.
Introduction
Concussion, a type of mild traumatic brain injury, has been defined through expert consensus as a complex pathophysiologic process that is the result of head trauma, and produces an onset of nonspecific signs or symptoms and/or changes in mental function. For clinicians who diagnose and manage concussions, decision making can be challenging and imprecise owing to the limited clinical tools available to understand postinjury deficits, and the translation of knowledge from the underlying pathophysiologic processes responsible for signs and symptoms to clinical care. Although concussion incidence increased from the early 2000s to mid 2010s, , more recent research indicates that sport-related concussions sustained during practice have decreased, as well as recurrent concussion rates from 2013 to 2018. These results suggest that perhaps medical management of concussion has evolved, and the use of updated practice approaches and preventative strategies may be helping to decrease the incidence of concussion. Despite these advancements, the physiologic processes that underlie the signs and symptoms of concussion, accurate methods to reliably diagnose concussion, and the time course of physiologic recovery remain difficult to discern.
Currently, concussion remains a clinical diagnosis with few objective and widely used assessments that measure physiologic functions, in part owing to the lack of paradigms available to assess pathophysiologic responses to injury in a feasible clinically viable manner. Some approaches such as advanced neuroimaging, cerebral blood flow assessment, or fluid biomarker analysis allow for the detection of persistent biological disruptions after clinically observed recovery, but the usefulness of these techniques within widespread clinical practice is low. As a result, concussion diagnosis is less precise than other injuries, such as musculoskeletal sport injuries, which may alter the perception of recovery. Despite this challenge, concussion management guidelines have evolved considerably over the past 2 decades. , , , Assessment methods such as the Sport Concussion Assessment Tool, and each subsequent version are intended for sideline use, and have a high positive predictive value for concussion identification. According to the most recent guidelines from various medical associations and expert consensus groups, a critical aspect of concussion management is a multifaceted measurement approach intended to examine the various physiologic functions that are disrupted by a concussion. These metrics include, but are not limited to, assessments of symptoms, mental status, neurologic function, vision, gait, balance, and sleep–wake disturbances. , , These approaches, however, have demonstrated a less than optimal level of reliability across time. Furthermore, recovery as identified by these measures may not truly reflect the physiologic restoration of the brain, leading to further complications after clearance to return to preinjury activities, such as sports. , Studies using neuroimaging or animal models have also shown that physiologic restoration of the brain takes longer than symptoms. Therefore, a thorough understanding of the physiologic disruption that occurs after a concussion may provide a translational link between impaired cell biology and appropriate clinical methods to consider when diagnosing and developing rehabilitation strategies for individuals with a concussion.
Although many clinical studies focus on the diagnostic accuracy of different assessments for concussion or track the trajectory of recovery, the interpretation of changes across time continue to suffer from the difficulty of disentangling the effects of recovery compared with practice or learning effects. Furthermore, many assessment approaches are subjective in nature, mitigating the ability to address causality within an investigation. As such, experimental models of concussion, primarily among rodents, have been used to better understand the mechanisms that underlie clinical signs and symptoms apparent after a concussion. Among the more common models that have been used to this point, closed-skull weight decrease, , lateral fluid percussion, controlled cortical impact, , and closed-skull controlled impact models have each been developed to explore molecular alterations, ion fluxes, the role of excitatory neurotransmitters in dysfunction, and glucose metabolism. Therefore, the purpose of this review article is to discuss the molecular pathophysiology of concussion by examining each of the aforementioned aspects as they relate to concussion and their potential clinical implications. An emphasis on the translational aspects of the work done in this area is provided so that clinicians who diagnose, manage, and treat individuals with concussion can approach decision making with an understanding of mechanisms responsible for clinical observations.
Neurometabolic cascade of concussion
An understanding of the acute pathophysiology of concussion has been laid out by many different researchers, led primarily by the work of Giza and Hovda. , In response to a concussive event, a cascade of pathophysiologic events occur simultaneously, leading to potential changes in mental status, symptoms, cognitive function, or motor control. Although a complex set of processes are responsible for each of these dysfunctional outcomes, researchers hypothesize that these changes are primarily the result of an abrupt neuronal depolarization, glucose metabolism changes, excitatory neurotransmitter release, altered cerebral blood flow, and disrupted axonal function, each occurring concurrently at different stages after the concussive event. At the cellular level, potassium flows out of the neuron, and sodium and calcium flow in. These altered ionic flux processes then trigger voltage- or ligand-gated ion channels throughout the brain, and the result is a “widespread neuronal depression” state, priming the cell for barrier dysfunction as well as the inability to clear debris, resolve inflammation, and release trophic factors to repair neuronal connections.
Owing to the ionic shifts after injury, the cell attempts to restore homeostasis via membrane ionic pumps. These pumps require energy, which is quickly exhausted. The ability to deliver energy, via ATP, to the cell is also impaired after a concussion, resulting in an energy crisis. In essence, there is a high demand for energy to restore homeostasis (ie, ionic pumps) paired with the simultaneous decreased ability to deliver energy (ie, altered cerebral blood flow). Furthermore, mitochondrial dysfunction may also occur owing to the increased calcium present with the cell, worsening the mismatch between demand for energy and the ability to produce or deliver energy. This state of mismatched energy demands may last for up to 10 days in adult animal models, and relates to behavioral impairments, , but the duration of recovery for these processes are not known in humans. This time period likely varies owing to cellular, individual, and environmental differences between people, although further investigations in humans are needed to determine which factors affect postconcussion cellular restoration.
The damage that occurs after a concussion can also affect the neuronal architecture. The integrity of microstructural features such as axons and microtubules collapse as a result of the calcium influx and subsequent phosphorylation, or axonal stretch. , Owing to the shear and/or tensile forces present during the traumatic insult, cellular transportation disruptions and axonal swelling have been observed. , In the pediatric population specifically, axonal myelination may affect the degree of disruption. Given the ongoing myelination that occurs during brain development, the axonal fibers and associated microstructures may be more vulnerable to traumatic injury in childhood relative to adulthood. Advanced neuroimaging approaches may yield insights into disrupted axonal structure: diffusion tensor imaging has been used to measure the diffusion properties of water, thus providing a detailed view of how microstructural tissue functions. However, the current widespread use of advanced neuroimaging approaches for clinical purposes does not occur. Recent research is encouraging related to its potential in the future. ,
In addition to the energy crisis and neuromechanical deformation of cells leading to cellular disruption, neuroinflammation may occur and lead to functional changes. In rodent models, inflammatory genes have been reported to be upregulated, and acute neuroinflammatory responses were seen such as greater microglia and macrophage presence, as well as reactive astrogliosis. Thus, inflammation may be a source of neuronal disruption after a concussive event. Greater concentrations of inflammatory markers in the brain may reduce behavioral responses, and metabolites such as myo-Inositol (an osmolyte) has been used as a marker of neuroinflammation. Myo-Inositol is a marker of astrocytes, which become hypertrophic during inflammatory responses to injury, and increases after a concussion owing to membrane damage. , Given that traditional neuroimaging cannot adequately identify postconcussion alterations, magnetic resonance spectroscopy allows for the detection of subtle abnormalities by measuring brain metabolites. A quantitative, noninvasive measurement apparatus, magnetic resonance spectroscopy has been used for diagnosis and prognosis in severe traumatic brain injury, mild traumatic brain injury, , and among former collision sport athletes. , These studies indicate that magnetic resonance spectroscopy can identify otherwise unnoticed deficits after concussion or repetitive head trauma and point to pathophysiologic changes such as neuroinflammation.
Excitatory neurotransmitters and ion flux
Within the neurometabolic cascade of events that occurs after a concussion, an acute increase in excitatory neurotransmitters release likely causes alterations to glutamate, ion channels and N-methyl-D-aspartate(NMDA) receptor function. After acute trauma, damage-associated molecular patterns (DAMPs), glutamate, glutamine, sodium, potassium, and calcium reach elevated levels for prolonged periods, altering cell function. DAMPs are proteins, nucleic acids, and other molecules that are present in cells before an injury that remain dormant until an injury occurs and an immune response is elicited. Although investigated within the context of cancer and rheumatoid arthritis, researchers are now investigating a possible connection between DAMPs and neuroinflammation after a concussion in and around the extracellular space. Adenosine 5ʹ-triphosphoate (ATP) acts as an important type of DAMP with both intracellular and extracellular roles after a concussive event. ATP is mediated by purinergic receptors, but the mechanisms of these receptors after a traumatic neuronal insult are not well-established. ATP and high mobility group box 1 (HMGB1) are secreted passively from dead cells and actively secreted by stressed cells to recruit immune cells, stem cells, and neighboring cells to clear debris and generate new tissue growth. HMGB1, another type of DAMP, has been identified as the proangiogenic factor that stimulates endothelial cells and macrophages, allowing for blood vessel repair and growth in damaged tissue. The release of ATP and HMGB1 precedes microglia activation and other immune signals. Microglia are the immune cells of the central nervous system, and are among the first responders after damage. , Microglia appear as early as 6 hours after a concussion, with noticeable morphologic changes within 72 hours after injury. The release of DAMPs and microglia activation occur rapidly in extracellular space after injury, and are associated with poor outcomes after trauma. , , Related to behavioral changes, outcomes among rodent models include decreased memory, , , altered motor coordination, and cognitive impairments. ,
In addition to damaged cells releasing DAMPs after a concussion or traumatic brain injury, cells also release an increased concentration of glutamine and glutamate. Glutamate receptors are ligand-gated ion channels that allow the passage of sodium and potassium, and in some cases small amounts of calcium. Glutamate receptors produce postsynaptic excitatory responses, perpetuating the release of glutamate and allowing for an increased ionic flow rate. This process leads to the synapses becoming toxic and increasing the risk of neuronal death. A toxic environment then activates protective mechanisms, where astrocytes mediate extracellular toxicity. Glutamate transporter 1 and glutamate aspartate transporter are high-affinity sodium-dependent glial transporters that mediate the bulk of glutamate transport. In astrocytes, glutamate is converted to glutamine by glutamine synthase and shuttled back to the presynaptic neuron to be used. However, a glutamate overload affects shuttling capabilities, leading to further damage owing to altered sodium concentrations. Rao and colleagues reported that excitotoxic neuronal death may occur as a result of traumatic brain injury generating a transient downregulation of transporter proteins (glutamate transporter-1 and glutamate aspartate transporter) within the injured side of the cortex. Without these transporters working efficiently, the toxicity of the cell cannot be mediated, resulting in cell death. Greater glutamate concentrations may also cause overstimulation of NMDA receptors, resulting in increased potassium and calcium concentration and flux through the neuronal membrane. , Accumulations have been identified during a concussive event in animal models, but return to homeostatic levels may occur as soon as 3 hours in mild to moderately injured cells. ,
Although existing research primarily focuses on increased intracellular and extracellular concentrations of calcium perpetuating cell death, Zander and colleagues suggest that sodium should also receive consideration. Calcium influx increases into the cell after potassium efflux and sodium influx, which creates dysregulation that overwhelms glutamate and NMDA receptors and results in cellular damage. Therefore, it is possible that sodium is one source of ion dysregulation, although further experimental evidence is required. In a study by Paiva and colleagues, sodium serum disorders were investigated in stable patients in the intensive care unit with a moderate to severe traumatic brain injury. Sodium serum disorders are not well-understood in the realm of neurologic disorders and even less well-understood in connection with neurologic trauma. The results suggest that sodium disorder incidence is higher among patients with diffuse traumatic brain injury relative to focal lesions. Thus, sodium regulation may play a central role in cellular dysregulation. Furthermore, sodium concentrations have been shown to become altered and persist for years after concussion symptom recovery, increasing the risk of negative outcomes, such as impaired memory.
Altered behavioral changes
In addition to the neurometabolic alterations that occur after a concussion, researchers have investigated altered brain function and behavioral changes following concussion in humans. Although most patients recover quickly, recent work suggests that 43% of athletes may experience symptoms for more than 7 days after the injury. However, concussion symptoms may arise owing to many different factors and, as such, they contain a low specificity for postconcussion problems, making it difficult to discern the mechanism by which they occur. Headache is one of the most common postconcussion symptoms reported, occurring in about 85% of patients. , External factors such as sleep dysregulation, emotional stress, and dehydration can increase the severity of headaches in patients with a concussion. , Approximately 30% to 85% of patients complain of sleep disturbances, with most resolving 3 months after injury. Other cognitive alterations can occur after a concussion, including complications with verbal and visual memory, processing speed, impulse control, orientation, attention, and executive function. , With a variety of symptoms and the lack of effective and quick diagnosis techniques, multidisciplinary investigation into treatment options is needed to help combat patient discomfort and decrease the amount of time out of work, school, and exercise. , Although these behavioral changes are apparent upon clinical presentation, determining their causes and subsequently identifying appropriate therapeutic targets for interventions among individuals with concussion remains challenging. To prevent long-term problems and initiate appropriate treatments, determining the extent and the factors and mechanisms of the problem must first occur.
Treatment
Many treatments have been proposed over the past decade. Although pharmacologic, nutritional, and therapy-based interventions have been tested, the most widely studied form of concussion treatment is subthreshold aerobic exercise. Traditionally, complete physical and cognitive rest has been prescribed until symptom resolution. However, researchers demonstrated that this strategy may actually have a negative effect on symptom recovery. More recent work has observed that, relative to a nonaerobic form of exercise (ie, stretching), a tailored exercise program below the level of symptom exacerbation can actually help to shorten symptom recovery times among adolescents. The physiologic mechanisms underlying this positive result have yet to be delineated. It is logical that a long period of rest, particularly among athletes, may lead to deconditioning that results in cerebrovascular control changes, and that these changes contribute to development of additional symptoms independent of the initial injury. The addition of regular exercise after an injury may, therefore, require the integration of multiple mechanism that relate to cerebrovascular function. After a brief rest period after injury, physical and cognitive rest seems to be an ineffective strategy to facilitate healing. Additional work examining the mechanisms by which symptom improvement occurs as a result of exercise after concussion are needed.
Other non–exercise-based treatments have been proposed to affect brain healing after a concussion. However, evidence is sparse and mixed to this point. Currently, we are unaware of any human-based studies that have investigated the role of supplement or vitamin-based intervention for concussion recovery. Furthermore, there is limited evidence to support the use of pharmacologic approaches to concussion treatment. Thus, multisite and prospective studies of concussion treatments and their mechanistic effects are required.
Summary
Concussion results in a cascade of complex, overlapping, and disruptive processes to the brain. These disruptions occur owing to many different pathophysiologic processes, including ionic shifts, neuronal architecture damage, increased neuroinflammation, increased release of excitatory neurotransmitters, and altered cerebral blood flow control. Collectively, a subsequent energy crisis ensues, given the mismatch between the need for energy to restore these disruptions in the brain, and a reduced ability to deliver energy to, or produce energy within, the brain. Although human-based studies have recently shed light on the restoration of these processes, recovery of each may occur within a distinct timeline and is likely affected by many individual factors. Treatments have focused on the use of aerobic exercise that does not worsen symptoms, and this approach shows promise to alleviate many of the pathophysiologic disruptions present after injury. Currently, adequate time for recovery should occur (ie, not returning to unrestricted activities) so as to not worsen ongoing disruptions, but early integration into regular physical activity that does not worsen outcomes after a concussion should be considered to facilitate a proper healing environment.
Clinics Care Points
- •
Clinical evaluation of pathophysiological disruption is currently difficult within typical clinical concussion evaluations.
- •
Emerging techniques, such as advanced neuroimaging may eventually provide useful clinical information about neuropathology after concussion.
- •
To understand how treatment affects pathophysiological functioning after concussion, further clinical studies are needed.
Disclosure
Dr D.R. Howell receives research support not related to this study from the Eunice Kennedy Shriver National Institute of Child Health & Human Development ( R03HD094560 ), the National Institute of Neurological Disorders and Stroke ( R01NS100952 , R41NS103698 , R43NS108823 ), and MINDSOURCE Colorado Brain Injury Network . The remaining author has nothing to disclose.
References
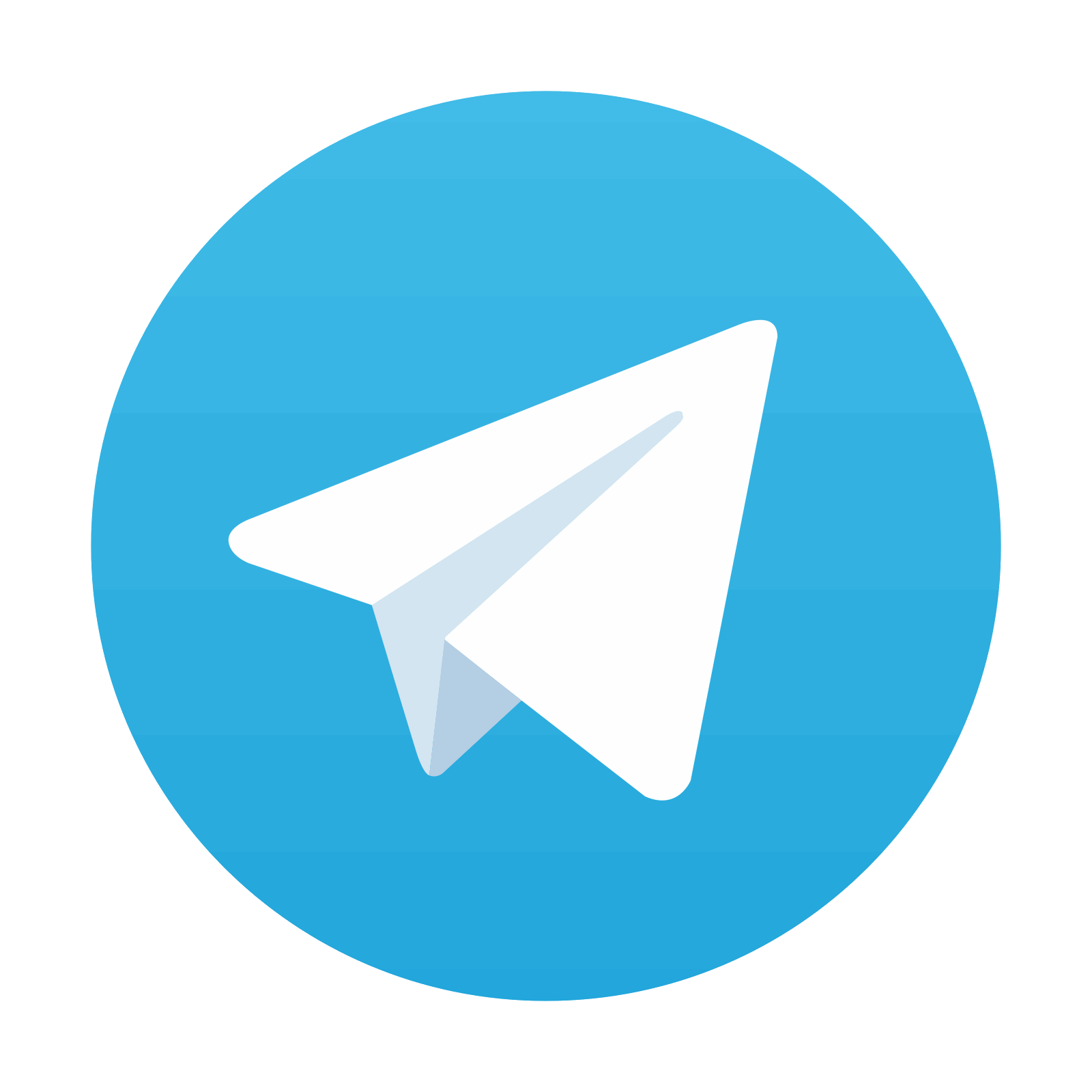
Stay updated, free articles. Join our Telegram channel

Full access? Get Clinical Tree
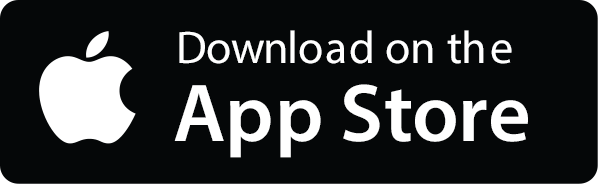
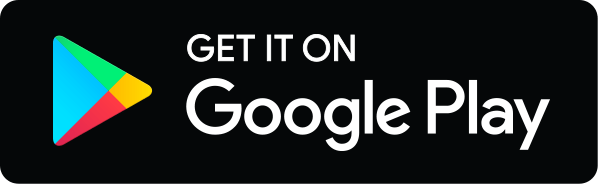
