FIGURE 2-1 Bone development. During embryonic development, undifferentiated cells proliferate and compact to form the limb bud. As these cells proliferate, regions within the limb bud that undergo greater condensation will form future long bones. A: Radiograph of a tibia showing the anatomic portions of the long bones. B: The ends of long bones consist of the epiphysis and metaphysis, with the growth plate between the two. C: Growth plate chondrocytes undergo a programmed differentiation pathway causing the longitudinal growth of the bones, ending in hypertrophy and programmed cell death. Indian hedgehog (IHH) and parathyroid hormone-like hormone (PTHLH) act in a negative feedback loop regulating this differentiation process. Vascular cells enter the center of the cartilaginous template of the bone, and osteoblasts replace the cartilage to form the primary center of ossification.
When bone is initially formed, it is immature woven bone, which is remodeled to more mature, and stronger, lamellar bone. During bone growth at the metaphysis, the trabeculae of bone-covered calcified cartilage (called primary spongiosa) are resorbed by osteoclasts and the cartilage template is replaced by lamellar bone, which is remodeled into more mature bone trabeculae (secondary spongiosa). The deposited secondary bone trabeculae at the metaphyseal–diaphyseal junction is further remodeled and incorporated into the diaphysis, in a process in which osteoclasts remove bone from the periphery of the metaphysis and new bone is formed at the endosteal surfaces. The cortical bone is remodeled into a complex structure of osteons that together form the cortical bone.31 Osteons are tubular structures that consist of layers of ordered lamellar bone around a central canal. The central canal contains blood vessels, lymphatics, and in some cases, nerves.45
Bone is constantly remodeled by osteoclasts and osteoblasts. Osteoblasts produce new bone, and start as bone-lining cells with slender cellular processes that make contact with the more mature osteocytes within the mineralized bone. Osteocytes are thought to arise from osteoblasts that have become entrapped during bone formation. Besides the cellular processes that connect osteocytes to one another and to the bone-lining cells, these cells also can sense their mechanical environment. When mechanical stress is higher, they will deposit more bone matrix, whereas if a lack of stress, bone resorption occurs. Osteoclasts are bone-degrading cells that are produced from hematopoietic progenitors. Upon activation, they bind to the surface of the bone and secrete enzymes into the space beneath. The space is acidic and contains proteolytic and bone-degrading enzymes.34,59,69
Osteoblast and osteoclast activities are linked during the bone remodeling. Osteoclastic activity releases growth factors, such as insulin growth factor and bone morphogenetic protein (BMP), from the matrix stimulating osteoblastic differentiation. Osteoblast lineage cells produce a protein called receptor activator of NF-κB ligand (or RANKL), which binds its receptor, RANK, on osteoclast precursors, to cause osteoclast activation. Osteoprotegerin (OPG), is a decoy receptor for RANKL, which blocks the binding of RANKL, and thus, inhibits the development of osteoclasts. Modulation of this system has been developed into a pharmacologic agent (Denosumab), which blocks RANK activation and osteoclast-mediated bone resorption50,93 (Fig. 2-2).
FIGURE 2-2 The linked activities of osteoclast bone resorption and osteoblast bone formation during bone remodeling. Bone-lining cells, osteoblasts, or some marrow stromal cells express RANKL, which activates receptor RANK on osteoclast progenitors of the monocyte–macrophage lineage and stimulates the osteoclast differentiation and activation. M-CSF is another essential factor for osteoclast differentiation. However, decoy receptor osteoprotegerin (OPG) binds RANKL and antagonizes RANK function and thus inhibits osteoclast formation. Activated osteoclasts secrete acid and proteases and erode bone on the surface. During the resorption process, sequestered growth factors, such as IGF-I, TGF-β, and FGFs, are released from the bone matrix and activated, which results in the recruitment, differentiation, and activation of the osteoprogenitors to become osteoblasts to initiate bone matrix synthesis and bone formation.
ANATOMIC REGIONS OF THE CHILD’S BONE
Long bones can be divided into epiphysis, physis, metaphysis, and diaphysis.
Epiphysis
The epiphysis is the region of a long bone between the end of the bone and the growth plate (or physis). At birth, the end of the bones is completely cartilaginous (except for the distal femur), and termed a chondroepiphysis. A secondary center of ossification forms at a specific time for each chondroepiphysis, which gradually enlarges until the cartilaginous area has been almost completely replaced by bone at skeletal maturity. The appearance of the ossification centers differs between different bones, and this needs to be taken into account when diagnosing fractures of these regions.10,57 As the ossification center matures, there is increased rigidity at the end of the bone, and this increase in rigidity is responsible for changes in the fracture pattern with age. Indeed, injuries that might not result in a fracture in this region in the very young may do so as the skeleton becomes more rigid.
The external surface of the epiphysis is composed of articular cartilage or perichondrium. Muscle fibers, tendons, and ligaments attach to the perichondrium, which also contributes to the centrifugal enlargement of the epiphysis. The perichondrium blends into the periosteum. This perichondrial/periosteal tissue continuity contributes to the biomechanical strength of the epiphyseal/metaphyseal junction at a region that is called the zone of Ranvier.14,88 Hyaline cartilage below the articular cartilage contributes to the growth of the epiphysis. As skeletal maturity is reached, a tidemark develops at the demarcation between the articular and calcified epiphyseal hyaline cartilage. Articular and hyaline chondrocytes cannot perform each other’s functions, and this was demonstrated by studies61 showing that when a contiguous core of articular and hyaline cartilage is removed, turned 180°, and reinserted, the transposed hyaline cartilage eventually will form bone at the joint surface, whereas the transposed articular cartilage remains cartilaginous and becomes surrounded by the enlarging secondary ossification center. Such a situation in a child will lead to a nonunion.
Physis
The physis, or growth plate, is located between the cartilaginous epiphysis and the newly generated bone in the metaphysis, is responsible for longitudinal bone growth. The perichondrium is a layer of dense connective tissue which surrounds the growth plate. Within the growth plate, chondrocytes undergo differentiation progressing through the resting, proliferation, prehypertrophic, and hypertrophic stages, eventually undergoing programmed cell death. The hypertrophic chondrocytes form columns parallel to the long axis of the bone, and produce type X collagen, which may act as a template for the new bone formation. Adjacent to the region where chondrocytes undergo apoptosis, blood vessels invade the cartilage matrix, presumably bringing in osteoblasts that produce endochondral bone.14,88,94 Because the epiphyseal cartilage remains radiolucent throughout the early stages of growth, its location is inferred from the metaphyseal contour, which follows the physeal contour.
The blood supply to the growth plate comes from the epiphyseal side. If a segment of the epiphyseal vasculature is compromised, the cartilaginous growth associated with the particular vessels slow. Unaffected regions of the physis continue normal growth; however, growth rates of the cells directly adjacent to the affected area are more compromised than cellular areas farther away. The differential growth results in an angular or longitudinal growth deformity, or both. In contrast, interruption of the metaphyseal circulation has no effect on chondrogenesis, but the transformation of cartilage to bone is blocked, causing widening of the growth plate. Once the disrupted metaphyseal circulation is reestablished, this widened region of the physis is ossified, returning the physis to its normal width (Fig. 2-3).
FIGURE 2-3 Patterns of response to ischemia of the growth plate. Response to ischemia of the epiphyseal (A, B) versus metaphyseal (C, D) circulatory systems. Metaphyseal ischemia is usually transient; epiphyseal ischemia is usually severe and permanent.
In the past decade there has been a substantial increase in research into the control of growth plate chondrocyte function. Such work is the first step in developing approaches to modulate growth plate function, such as using a drug, a cell, or a biologic approach. Such treatments could someday be used to treat a partial growth arrest following an injury. Research using genetically modified mice is one approach that has been particularly useful in improving our knowledge about growth plate regulation. This is because mice can be genetically engineered to have particular genes deleted from just chondrocytes, thus providing a robust method to determine the function of a particular gene. Chondrocytes develop from an undifferentiated mesenchymal precursor cell, sometimes called MSCs, which differentiate into a common osteochondroprogenitor cell. The Wnt/β-catenin signaling pathway plays a key role in determining if these cells become osteoblasts or chondrocytes, as in the absence of β-catenin they develop into chondrocytes. Several drugs are available that modulate β-catenin, and these could someday be used to modulate growth plate activity.37,39,51 Once cells become committed to become growth plate chondrocytes, they undergo a coordinated process of differentiation, with expression of various genes (SOX9, IHH, PTHrP, RUNX2, and then type X Collagen)1,40,96 marking the states of differentiation. Resting cells proliferate, then hypertrophy, before undergoing terminal differentiation, where they express type X Collagen and become replaced by bone. The resting cells maintain the growth plate cells, and as such these cells located nearest to the epiphysis are critical to normal bone growth, and damage to these cells will permanently disrupt growth.
The hedgehog (Hh) signaling pathway is crucial in the regulation of chondrocyte fate in the growth plate. Prehypertrophic chondrocytes in the growth plate express the Hh ligand Indian Hh (IHH).11,56,73 IHH serves as a key regulator of endochondral ossification, acting in a negative feedback look with parathyroid hormone-like hormone (PTHLH), also called parathyroid hormone-related protein (PTHrP). IHH regulates the onset of hypertrophic differentiation by signaling the periarticular chondrocytes to upregulate PTHLH which inhibits the differentiation of proliferating chondrocytes. IHH also regulates chondrocyte differentiation and induces ossification of the perichondrium in a PTHLH-independent manner.52 The regulation of PTHLH by IHH involves mediators such as BMPs which also play a role initiating the chondrocyte differentiation cascade.62 In this way IHH and PTHrP act in a feedback loop controlling the pace of growth plate chondrocyte differentiation (Fig. 2-1).
Metaphysis
The metaphysis is the flared portion of the bone at each end of the diaphysis. It has a decreased cortical thickness and increased volume of trabecular bone in the secondary spongiosa. During growth, endochondral modeling centrally and peripherally initially forms the primary spongiosa, which then is remodeled into the more mature secondary spongiosa by osteoclasts and osteoblasts. For this reason, there is considerable bone turnover in the metaphysis compared to other regions of the bone. The metaphyseal cortex is thinner and is more porous than the diaphysis, and there are cortical fenestrations, which contain fibrovascular soft tissue elements that connect the metaphyseal marrow spaces with the subperiosteal region. The metaphyseal region does not develop extensive secondary and tertiary Haversian systems until the late stages of skeletal maturation. These microscopic and anatomic changes correlate with changing fracture patterns, and the ability of bone to deform without breaking in this region is why buckle (or torus) fractures are more likely to occur than complete metaphyseal or epiphyseal/physeal fractures.46,75,95
Although the periosteum is attached relatively loosely to the diaphysis, it is firmly fixed to the metaphysis because of the increasingly complex continuity of fibrous tissue through the metaphyseal fenestrations. The periosteum subsequently attaches densely into the peripheral physis, blending into the zone of Ranvier as well as the epiphyseal perichondrium. The zone of Ranvier is a specialized region between bone and cartilage formation, and cells in this zone contribute to growth plate remodeling over time.14,88 The fenestrated metaphyseal cortex extends to the physis as the thin osseous ring of Lacroix. There are no significant direct muscle attachments to the metaphyseal bone; instead, muscle fibers primarily blend into the periosteum. The medial distal femoral attachment of the adductor muscles is a significant exception. Because of extensive remodeling and insertion of muscle and tendon in this area, the bone often appears irregular and may be misinterpreted as showing chronic trauma.
Growth Lines of Park and Harris
Many bones exhibit transversely oriented, dense trabecular linear bone patterns within the metaphysis. These lines duplicate the contiguous physeal contour, and appear after processes which transiently slow growth or increase mineralization. As such, they are seen after generalized illnesses, treatment with bisphosphonate drugs (which inhibit osteoclasts, and therefore increase mineralization), or after localized processes within the bone, such as infection or growth plate trauma. The lines are called Harris or Park growth slowdown or arrest lines. Once the normal longitudinal growth rate resumes, longitudinal trabecular2 orientation is restored. The thickened, transversely oriented osseous plate is left behind, and will be gradually remodeled, and with time will disappear89 (Fig. 2-4).
FIGURE 2-4 Growth lines. Histologic section (A) and x-ray study (B) of a distal femur showing a typical Harris line (arrows). This is formed during an acute illness and chemotherapy for leukemia. The child then resumed a more normal pattern of growth until her death from leukemia about 14 months later.
In a systemic problem slowing bone growth, the lines are distributed relatively symmetrically throughout the skeleton, and are thickest in metaphyses from bones that grow most rapidly. In response to administration of bisphosphonate in children (e.g., in the treatment of osteogenesis imperfecta) there are distinct metaphyseal bands in the growing skeleton, whose spacing is determined by the frequency of treatment, age of the patient, and rate of growth. These are sometimes called zebra lines.2 The lines are important in analyzing the effects of a fracture on growth. They can be measured and the sides compared to corroborate femoral overgrowth after diaphyseal fracture and eccentric overgrowth medially after proximal tibial metaphyseal fracture. A line that converges toward a physis suggests localized growth damage that may result in an osseous bridge and the risk of angular deformity (Fig. 2-5). This radiographic finding is best evaluated roughly 6 months after the injury, and requires an x-ray centered over the physis.55,72 If a line parallel to, but separate from the growth plate is seen at this time point, the risk of future growth disturbance is very low.
FIGURE 2-5 Example of a growth arrest line converging toward the area of physeal arrest at the medial proximal tibial physis. (Used with permission from the Children’s Orthopedic Center, Los Angeles.)
Diaphysis
The diaphysis constitutes the major portion of each long bone, and is formed from bone remodeled from the metaphysis. Mature, lamellar bone is the dominant feature of the diaphyseal bone, and the developing diaphyseal bone is extremely vascular. When analyzed in cross section, the center is much less dense than the maturing bone of older children, adolescents, and adults. Subsequent growth leads to increased complexity of the Haversian (osteonal) systems and the formation of increasing amounts of extracellular matrix, causing a relative decrease in cross-sectional porosity and an increase in hardness. Some bones, such as the tibia, exhibit a decrease in vascularity as the bone matures; this factor affects the rate of healing and risk of nonunion.13,74 The vascularity of bone is important not only because it bring nutrients to the bone, but also because pericyte cells surrounding blood vessels contribute to new osteoblasts.7 In addition, hematopoietic progenitor cells exist in the vascular centre of the bone, where bone cells are required to maintain the stem cell population maintaining blood cells.79
PERIOSTEUM
A child’s periosteum is thicker, is more readily elevated from the bone than in adults. It also has a much greater osteogenic potential than that of an adult. Indeed, in young children, one can remove the entire diaphysis of a bone, but leave the periosteum, and the bone will regrow. The thicker and stronger periosteum affects fracture displacement, ease of closed reduction, and the capacity to form new bone. The periosteum is an important internal restraint in closed reductions, where it helps to both obtain and maintain acceptable fracture alignment. The periosteum usually remains intact on the concave (compression) side of an injury. This intact periosteal hinge or sleeve may lessen the extent of displacement of the fracture fragments, and it also can be used to assist in the reduction, because the intact portion contributes to the intrinsic stability. Thus, accentuating the deformity, unlocks the periosteum, helping with the reduction. Because the periosteum allows tissue continuity across the fracture, the subperiosteal new bone that forms quickly bridges the fracture gap and leads to more rapid long-term stability.3,8
The periosteum comprises two tissue layers. An outer fibroblast layer provides fibrous attachment to subcutaneous connective tissue, muscles, tendons, and ligaments, whereas the inner cambium layer contains a pool of cells that support bone formation and repair. The periosteum, rather than the bone itself, serves as the origin for muscle fibers along the metaphysis and diaphysis. This mechanism allows coordinated growth of bone and muscle units; something that would be impossible if all the muscle tissues attached directly to the developing bone or cartilage. Exceptions include the attachment of muscle fibers near the linea aspera and into the medial distal femoral metaphysis. The latter pattern of direct metaphyseal osseous attachment may be associated with significant irregularity of cortical and trabecular bones. Radiographs of this area often are misinterpreted as showing a neoplastic, osteomyelitic, or traumatic response, even though this is actually a variation of skeletal development. The periosteum in the growing child also plays a critical role in remodeling, as the tissues in tension over the concave side of a deformity will produce new bone. The bone on the tension, or convex, side of a deformity will be resorbed over time, ultimately resulting in a straight diaphysis.3,8,42
APOPHYSIS
An apophysis is composed of fibrocartilage instead of columnar cartilage and grows primarily in response to tensile forces. They are generally attached to muscular structures. With growth secondary ossification centers can form in the apophysis. Because of the differing histologic composition of these structures, they fail differently than other parts of the bone, and excessive tensile stress may avulse the apophysis, especially during the late stages of closure. Such injuries can generate large amounts of new bone, and may be mistaken for tumors, especially around the pelvis. Healing of a displaced fragment to the underlying undisplaced secondary center creates the symptomatic reactive overgrowth, and in the tibial tuberosity apophysis, this is known as an Osgood–Schlatter lesion.25,60,81
COMPOSITION OF CARTILAGE AND BONE
The cartilage matrix is synthesized by chondrocytes, and the main constituents of the cartilaginous matrix are collagens (mainly type II) and proteoglycans. Although collagen provides structural strength, the proteoglycans contribute the ability to respond to resist deformation, and also has regulatory effects on cells. Except for a small percentage of molecules from the circulation and pre-existent matrices that may become entrapped, the bone matrix is almost entirely synthesized by osteoblasts. Bone matrix is a composite material composed of an inorganic (mineral) portion and an organic portion. The composition of living bone is 60% to 70% inorganic components, 5% to 8% water, and the remainder 22% to 35% is organic. The inorganic portion is mainly hydroxyapatite, with some carbonate and acid phosphate groups. It has also been suggested that bone crystals do not contain hydroxyl groups and should be termed apatite rather than hydroxyapatite. The organic portion is composed of collagen type I (90%) and noncollagenous proteins. The noncollagenous protein portion includes a number of proteins and proteoglycans that perform structural and regulatory functions. The composite structure provides physical strength and resilience to fracture. Bone with deficient inorganic mineral content is pliable, and bone with deficient organic content is brittle.5,43,63
Proteoglycans are critical components of cartilage and bone, and are present in large amounts within all connective tissues. They have either one or a number of polysaccharide chains linked to a protein core. The polysaccharide’s glycosaminoglycan side chains are heparin, heparin sulfate, chondroitin sulfate, dermatan sulfate, or keratan sulfate. The glycosaminoglycans differ in the composition of their constituent disaccharide structures. They can combine with other molecules within the matrix to form macromolecular structures. The proteoglycans present in the physis include large proteoglycans like aggrecan as well as smaller proteoglycans, such as decorin and biglycan. Decorin and biglycan have side chains of dermatan sulfate, and betaglycan has chondroitin and heparin sulfate chains. Fibromodulin has side chains of keratan sulfate. Proteoglycans have not only a structural role but also interaction with growth factors.82
FRACTURE REPAIR MECHANISMS
Fracture healing is a complex regenerative process initiated in response to injury, in which bone can heal by primary or secondary mechanisms. In primary healing, new bone is laid down without any intermediate. This type of healing is rare in a complete bone fracture, except when the fracture is rigidly fixed through certain types of surgery. In the more common secondary healing, immature and disorganized bone forms between the fragments, which is termed the callus.15,26,61,77 During the fracture repair process, cells progress through stages of differentiation reminiscent of those that cells progress through during normal fetal bone development. In normal development of long bone, undifferentiated mesenchymal cells initially form a template of the bone, which differentiate to chondrocytes. This cartilaginous template is termed the bone’s anlage. Following this phase, blood vessels enter the cartilaginous template, and osteoblasts, which differentiate from perivascular and other cells surrounding the bone form bone.
There are, however, several important differences between bone repair, and development. One is that repair does not need to progress through a cartilaginous template. Another is that the liberation of growth factors in the extracellular environment and inflammatory mediators initiates fracture repair, and the activation of these factors does not occur during development. Indeed, this inflammatory initiation of repair processes may be the fundamental difference between development and regeneration. This is one reason that agents that modulate inflammation can affect bone formation. Although some inflammatory pathways can have both positive and negative effects on bone repair, an inhibition of prostaglandin activity inhibits bone formation, and indeed this has been used clinically to prevent bone formation.12,65,80
Osseous repair progresses through closely integrated phases. In the initial phase of fracture repair, bleeding from the damaged tissues causes a hematoma at the fracture site, stopping blood loss and liberating growth factors and cytokines. Endothelial cells respond by increasing their vascular permeability, allowing leukocytes, monocytes, macrophages, and multipotential mesenchymal cells to reach the fracture site.70 The blood supply is temporarily disrupted for a few millimeters on either side of the fracture site, producing local necrosis and hypoxia. It is likely that necrosis also results in the release of sequestered growth factors (e.g., BMPs), which promotes differentiation of the surrounding mesenchymal cells into bone-forming cells.16,20,49,68,87 In the proliferative phase, undifferentiated mesenchymal cells aggregate at the site of injury, proliferate, and differentiate presumably in response to growth factors produced by the injured tissues.15 This process involves both intramembranous and endochondral ossification. Intramembranous ossification involves the formation of bone directly from committed osteoprogenitor cells and undifferentiated mesenchymal cells that reside in the periosteum, resulting in hard callus formation.84 During endochondral ossification, mesenchymal cells differentiate into chondrocytes, producing cartilaginous matrix, which then undergoes calcification and eventually is replaced by bone. The formation of primary bone is followed by extensive remodeling until the damaged skeletal element regains its original shape and size26,61,84 (Fig. 2-6).
FIGURE 2-6 Phases of fracture repair. The figure demonstrates the three phases of fracture repair (A) inflammatory phase, (B) reparative phase, and (C) remodeling phase. The inflammatory cells remove the debris from the fracture site and, together with the fibroblastic cells, develop the site into a matrix that will support the cells that enable new bone to be formed. The mesenchymal cells are recruited by the release of growth factors in the fracture site. The mesenchymal cells may differentiate into osteoblasts that produce bone in a membranous fashion. Alternately the mesenchymal cell may become chondrogenic and produce bone by the endochondral pathway. Remodeling begins with resorption of mechanically unnecessary, inefficient portions of the callus and the subsequent orientation of trabecular bone along the lines of stress.
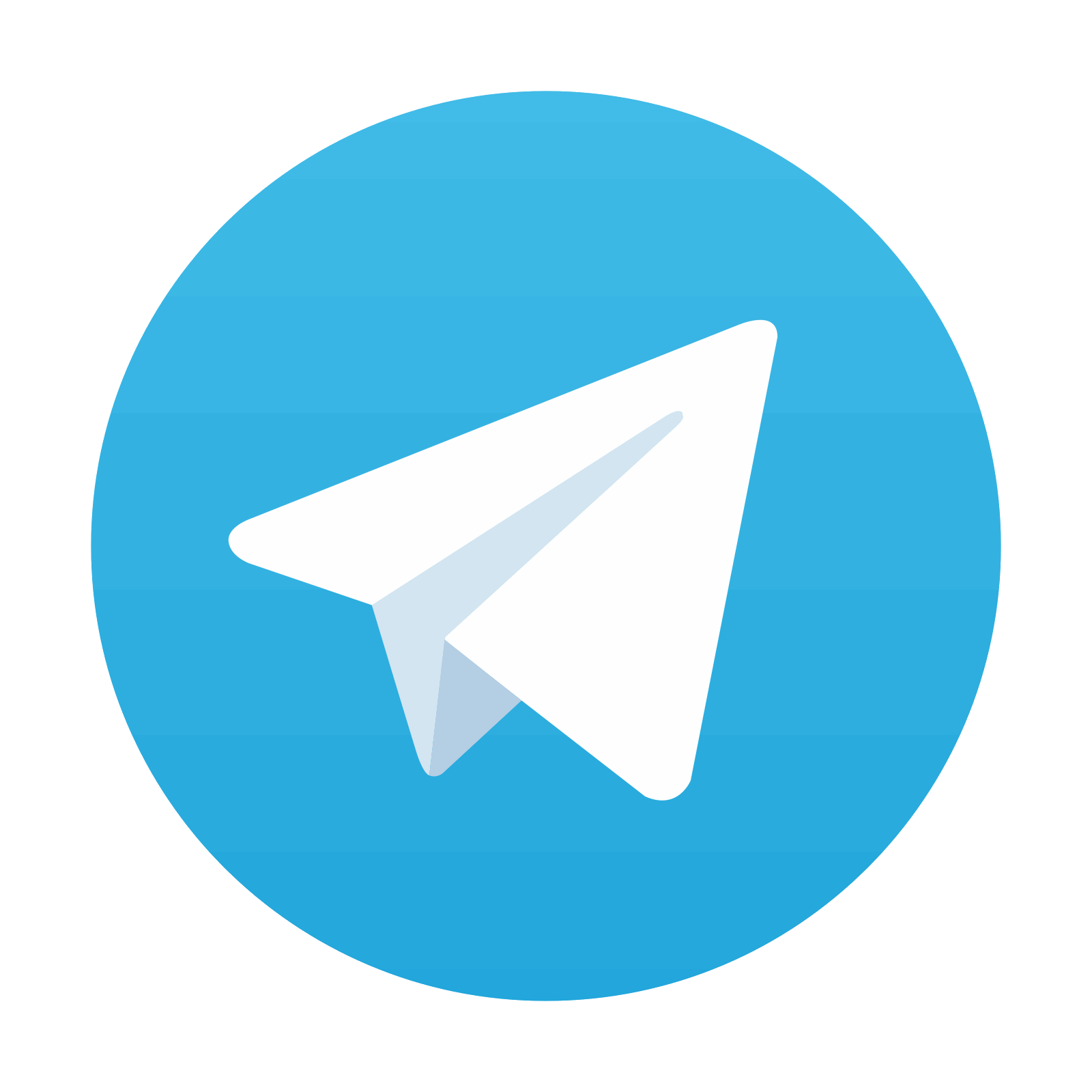
Stay updated, free articles. Join our Telegram channel

Full access? Get Clinical Tree
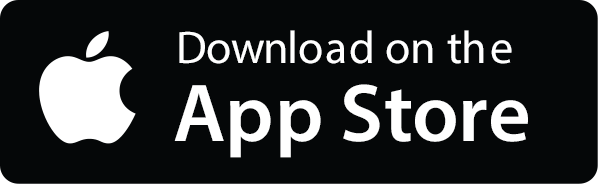
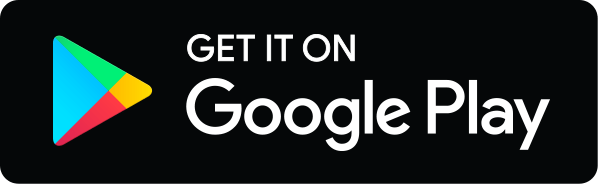