The introduction of cyclophosphamide for treatment and the detection of antineutrophil cytoplasmatic antibodies (ANCA) as a seromarker for ANCA-associated vasculitis (AAV) have been the most important milestones in the history of AAV. Nevertheless, there are still many issues to resolve to fully understand the pathogenesis of AAV and to improve patient outcomes. There is a need for diagnostic criteria; treatment strategies need further improvement to reduce the toxicity of conventional immunosuppressants such as cyclophosphamide. The elucidation of the genetic background in patients with AAV and the role of granulomatous lesions found in Wegener’s granulomatosis are required to fully understand the pathophysiology of AAV.
In the 1950s, the vasculitides associated with antineutrophil cytoplasmic antibodies (ANCA) (Wegener’s granulomatosis [WG], microscopic polyangiitis [MPA], and Churg-Strauss syndrome [CSS]) were characterized by fatal outcome with a mortality of 80% within 1 year. A median patient survival of 5 months was described for WG because no effective treatments for WG and other ANCA-associated vasculitis (AAV) were available. In the 1970s, the introduction of the Fauci regimen, namely treatment with cyclophosphamide (Cyc) and glucocorticoids, represented a milestone in the improvement of patients survival. Since then, several important advances have been made in the pathophysiology and treatment of AAV. The detection of ANCA as a serologic marker in the 1980s facilitated the diagnosis of AAV. Subsequently, it was shown by in vitro studies and animal models that ANCA plays an important role in the pathogenesis of small-vessel vasculitis. In the 1990s, the establishment of classification criteria and disease definitions led to major progress in categorizing disease entities. The European Vasculitis Study Group (EUVAS) defined disease stages for AAV (such as localized and generalized) and initiated the first controlled trials to provide evidence for efficacy of therapy based on disease stage and disease activity. Validated scores were introduced to assess disease activity (Birmingham Vasculitis Activity Score [BVAS]), disease extent (Disease Extent Index [DEI]), and damage caused by AAV (Vasculitis Damage Index [VDI]).
Today, we have a good understanding of the pathophysiology of ANCA-induced vasculitis, but the pathophysiologic role of granulomatous lesions in WG and CSS and the role of eosinophils in CSS remain largely unknown. The authors follow a stage- and activity-based treatment regimen that helps to further improve outcome and curtail toxic treatment regimens. Nevertheless, there is an ongoing need for less toxic treatment strategies. Furthermore, the diagnosis of vasculitis is often made using classification criteria or disease definitions, because diagnostic criteria for the primary vasculitides are still lacking.
Future milestones in the understanding of the pathophysiology of AAV will be to elucidate the role of granulomatous lesions in WG and CSS. Understanding the genetic background of AAV (eg, by genome-wide association and subsequent fine-mapping studies) will probably offer valuable information to fully understand questions surrounding the pathogenesis of AAV and its different phenotypes. A strict classification of disease entities and phenotypes is needed, and diagnostic criteria need to be established. Regarding treatment strategies, the substitution of a less toxic treatment option instead of Cyc would be a major milestone. Rituximab is a good candidate, but long-term studies are needed to evaluate its side effects and toxicity. Moreover, answers need to be found to the questions regarding the duration of maintenance therapy required in AAV and delineation of the doses of glucocorticoids needed to control the disease during remission induction and maintenance.
Issues to resolve in the pathophysiology of AAV
Genetics
The limited knowledge to date on the genetic background of AAV has been gained from candidate-gene approaches. The most remarkable genetic associations in WG, which have been replicated by several studies, are those with the deficiency allele PIZ and PIS of the protease-inhibitor 1 (also referred to as alpha1-antitrypsin) gene (odds ratio [OR] for ZZ/SS/SZ compared with MM, 14.62) ; for the HLA-DPB10401 allele (OR 3.38–3.91) and the 620 W allele of PTPN22 (OR 1.76). Deficiency of alpha1-antitrypsin may lead to an increased presence of the autoantigen PR3 in the circulation, which may be one factor for the induction of autoimmunity against PR3. However, not all homozygous PIZ carriers develop WG, so additional factors must be required for the development of WG. HLA genes contribute to the risk of almost all autoimmune diseases, although the exact mechanism is not well understood. Certain HLA alleles induce a specific structure of the major histocompatibility complex peptide and/or its binding cleft, which facilitates the presentation of self-antigens to autoreactive lymphocytes. Outside the HLA region, PTPN22 is a susceptibility gene for many autoimmune diseases. PTPN22 encodes lymphoid tyrosine kinase (LYP), a protein tyrosine phosphatase that decreases IL-2 production and inhibits T-cell receptor signaling. As a result of a single nucleotide polymorphism inducing a change in the encoded protein from arginine to tryptophan at position 620, the T cell receptor signaling is reduced. The exact pathogenic mechanism of reduced T-cell receptor signaling predisposing to autoimmunity is not fully understood, but may be related to impaired negative selection of T cells in the thymus or a reduction of regulatory T cells. HLA-DPB10401 and the PTPN22 R620 W allele have been linked to ANCA-positive WG with the latter also being associated with vasculitic manifestations such as glomerulonephritis. Moreover, PTPN22 R620 W is a genetic risk factor for MPA, including association with positive ANCA status. In contrast, among AAV, HLA-DPB0401 seems to be a specific risk factor for WG, as no associations with this allele have been found in MPA (unpublished observation) or CSS. Other HLA genes have been implicated as risk factors for CSS (such as HLA-DRB1, -DRB3, -DRB4). Recently, a protective haplotype of IRF5 has been identified for WG (OR 0.73) in a large study population (n>600), implicating that the interferon (IFN) type I system (IFNα and IFNβ) is involved in the pathogenesis of WG. Compared with controls, the protective effect was stronger in patients with systemic disease than in those with localized disease (OR 0.68, P = .0000641 vs OR 0.8, P = not significant). In another recent study, a certain haplotype of the IL-10 gene (IL-10.2 haplotype) has been associated with CSS, in particular with ANCA-negative CSS, but not with WG ; this finding may be linked to the increased serum levels of IL-10 observed in CSS. Although the results of the latter two studies need replication, they are important because they have been performed in a large study population and point to the differences in genetic background of the different diseases, and to different phenotypes within one disease.
In summary, the evidence from genetic studies in AAV suggests that the genetic background consists of risk factors that are common to several autoimmune diseases, including AAV (such as PTPN22 R620 W ). There are also unique genetic risk factors characteristic of one AAV (such as HLA- DPB10401 for WG) or even for a phenotype within one AAV (such as the IL10.2 haplotype being associated mainly with ANCA-negative CSS). Therefore, disease groups and phenotypes need to be considered carefully for genetic association studies to dissect the underlying pathogenetic disease mechanisms.
In the future, the genome-wide association studies will be a powerful tool to identify further genetic risk factors. It will then be important to link identified genetic risk factors to functional studies to fully understand the genetic basis for AAV and their subgroups or phenotypes.
Induction of an adaptive immune response in WG
Target Antigens in AAV
Whereas there is at least some evidence from in vitro studies and from animal models for the pathophysiologic role of circulating ANCA, far less is known about the induction or generation of ANCA. Proteinase 3 (PR3) and myeloperoxidase (MPO) are currently recognized as the main target antigens in AAV. This view has recently been challenged, however, by the detection of human lysosomal membrane protein-2 (LAMP-2) antibodies in the serum of patients with pauci-immune glomerulonephritis, 50% of whom also display antibodies against PR3 or MPO. It is not certain therefore whether all relevant target antigens have been identified and whether the possible disease mechanisms elucidated in vitro or in animal models based on the assumption that PR3 and MPO are indeed the main target antigens reflect the true basis for the pathogenesis of AAV. Furthermore, it is not known why immunity against PR3 is more likely to induce the phenotype of WG characterized by formation of granulomatous lesions and vasculitic manifestations, whereas immunity against MPO seems to convey the phenotype of more or less purely vasculitic MPA.
Factors that May Contribute to the Induction of an Adaptive Immune Response
Assuming that PR3 is the main target antigen in WG, there is some evidence of how an adaptive immune response in WG is induced. Factors that may contribute to the induction of an adaptive immune response include increased presence of the autoantigen itself. In addition, cofactors may help to induce the activation of autoreactive immune cells. These cofactors may include bacterial stimuli that provide a proinflammatory environment or function as molecular mimics; or drugs that may modify the autoantigen to render it immunogenic. In WG there is an increased presence of the autoantigen PR3 in the circulation: Polymorphonuclear cells of patients with WG patients have increased percentages of membrane PR3 expression and a higher percentage of mPR3 expression has been detected in relapsing patients. Furthermore, there is good evidence to assume that infectious agents function as triggers of autoimmunity. First, localized or systemic infections have been shown to increase the presence of PR3 or MPO. Second, nasal carriage of Staphyloccous aureus is associated with increased relapse. Third, the occurrence of ANCA (mainly PR3-ANCA) and of vasculitic manifestations during infections such as endocarditis, parvovirus B 19 infection–associated arthritis, and chronic hepatitis C infection have been described. ANCA and clinical vasculitic manifestations typically disappear after adequate antibiotic therapy in endocarditis and other infections. Recently, the breaking of tolerance to another potential ANCA target antigen (LAMP-2) by the bacterial antigen FimH was described for the first time in a rat model, suggesting that bacterial agents may indeed function as molecular mimics for ANCA antigens and may therefore play an important role in the generation of an adaptive immune response in AAV.
Drugs are other candidates that have been associated with induction of ANCA and vasculitis. Most frequently, propylthiouracil (PTU) has been implicated in the induction of MPO-ANCA, and hydralazine, sulfasalazine, and cocaine have been associated with the induction of ANCA. Several cross-sectional and prospective studies showed a prevalence of 20% to 64% of PTU-induced ANCA, however few patients suffered from PTU-induced vasculitic manifestations. The mechanism of ANCA induction by drugs remains unclear. It has been suggested that modification of ANCA target antigen by the drug and/or its metabolites may render the target antigen immunogenic, as suggested for PTU. Antibodies to citrullinated peptides in rheumatoid arthritis are a good example of peptide modification that induces an autoimmune response. The concept of a target antigen requiring modification to become immunogenic is intriguing and may be a prerequisite for AAV. Nevertheless, it is not understood why the exposure to certain drugs leads to the induction of ANCA in only some of the exposed individuals; moreover, it remains to be elucidated why some individuals develop drug-induced ANCA only and some develop drug-induced ANCA with vasculitis.
In Vitro and in Vivo Models for the Induction of an Adaptive Immune Response
So far, the most convincing evidence of how an immune response is raised against ANCA target antigens comes from 2 studies. An in vitro study showed that PR3 induces maturation of GM-CSF/IL-4 dendritic cells (DC) derived from monocytes in vitro via protease receptor-2 (PAR-2) ; these DC can activate PR3-specific CD4 + T cells. Furthermore, Kain and colleagues showed that immunization of rats with the bacterial protein FimH induced antibodies cross-reactive to LAMP-2 that are expressed on the surface of neutrophils; these antibodies to LAMP-2 cause pauci-immune focal necrotizing glomerulonephritis when injected into rats. As mentioned earlier, antibodies to human LAMP-2 were detected with a high prevalence (93%) in a cohort of patients with pauci-immune glomerulonephritis who were positive for PR3- or MPO-ANCA in only 50% of cases. Another study suggests a link between infection and AAV by showing that ANCA production could be induced solely by CpG stimulation of B cells isolated from the circulation of AAV patients ; further studies are needed to clarify the mechanism involved in this finding.
The focus of most other studies of animal models was to prove pathogenicity of ANCA rather than to elucidate the mechanisms of ANCA induction (see later discussion).
In the future, it will be essential to identify the exact conditions that are required to induce autoimmunity against ANCA antigens. The search for other potential target antigens and the revelation of how an autoantigen relates to a certain disease or disease phenotype will be central issues to elucidate the pathophysiology of AAV. The understanding of ANCA induction will offer new targets for therapeutic interventions that interfere with the inception of the disease, even before ANCA is induced.
Induction of an adaptive immune response in WG
Target Antigens in AAV
Whereas there is at least some evidence from in vitro studies and from animal models for the pathophysiologic role of circulating ANCA, far less is known about the induction or generation of ANCA. Proteinase 3 (PR3) and myeloperoxidase (MPO) are currently recognized as the main target antigens in AAV. This view has recently been challenged, however, by the detection of human lysosomal membrane protein-2 (LAMP-2) antibodies in the serum of patients with pauci-immune glomerulonephritis, 50% of whom also display antibodies against PR3 or MPO. It is not certain therefore whether all relevant target antigens have been identified and whether the possible disease mechanisms elucidated in vitro or in animal models based on the assumption that PR3 and MPO are indeed the main target antigens reflect the true basis for the pathogenesis of AAV. Furthermore, it is not known why immunity against PR3 is more likely to induce the phenotype of WG characterized by formation of granulomatous lesions and vasculitic manifestations, whereas immunity against MPO seems to convey the phenotype of more or less purely vasculitic MPA.
Factors that May Contribute to the Induction of an Adaptive Immune Response
Assuming that PR3 is the main target antigen in WG, there is some evidence of how an adaptive immune response in WG is induced. Factors that may contribute to the induction of an adaptive immune response include increased presence of the autoantigen itself. In addition, cofactors may help to induce the activation of autoreactive immune cells. These cofactors may include bacterial stimuli that provide a proinflammatory environment or function as molecular mimics; or drugs that may modify the autoantigen to render it immunogenic. In WG there is an increased presence of the autoantigen PR3 in the circulation: Polymorphonuclear cells of patients with WG patients have increased percentages of membrane PR3 expression and a higher percentage of mPR3 expression has been detected in relapsing patients. Furthermore, there is good evidence to assume that infectious agents function as triggers of autoimmunity. First, localized or systemic infections have been shown to increase the presence of PR3 or MPO. Second, nasal carriage of Staphyloccous aureus is associated with increased relapse. Third, the occurrence of ANCA (mainly PR3-ANCA) and of vasculitic manifestations during infections such as endocarditis, parvovirus B 19 infection–associated arthritis, and chronic hepatitis C infection have been described. ANCA and clinical vasculitic manifestations typically disappear after adequate antibiotic therapy in endocarditis and other infections. Recently, the breaking of tolerance to another potential ANCA target antigen (LAMP-2) by the bacterial antigen FimH was described for the first time in a rat model, suggesting that bacterial agents may indeed function as molecular mimics for ANCA antigens and may therefore play an important role in the generation of an adaptive immune response in AAV.
Drugs are other candidates that have been associated with induction of ANCA and vasculitis. Most frequently, propylthiouracil (PTU) has been implicated in the induction of MPO-ANCA, and hydralazine, sulfasalazine, and cocaine have been associated with the induction of ANCA. Several cross-sectional and prospective studies showed a prevalence of 20% to 64% of PTU-induced ANCA, however few patients suffered from PTU-induced vasculitic manifestations. The mechanism of ANCA induction by drugs remains unclear. It has been suggested that modification of ANCA target antigen by the drug and/or its metabolites may render the target antigen immunogenic, as suggested for PTU. Antibodies to citrullinated peptides in rheumatoid arthritis are a good example of peptide modification that induces an autoimmune response. The concept of a target antigen requiring modification to become immunogenic is intriguing and may be a prerequisite for AAV. Nevertheless, it is not understood why the exposure to certain drugs leads to the induction of ANCA in only some of the exposed individuals; moreover, it remains to be elucidated why some individuals develop drug-induced ANCA only and some develop drug-induced ANCA with vasculitis.
In Vitro and in Vivo Models for the Induction of an Adaptive Immune Response
So far, the most convincing evidence of how an immune response is raised against ANCA target antigens comes from 2 studies. An in vitro study showed that PR3 induces maturation of GM-CSF/IL-4 dendritic cells (DC) derived from monocytes in vitro via protease receptor-2 (PAR-2) ; these DC can activate PR3-specific CD4 + T cells. Furthermore, Kain and colleagues showed that immunization of rats with the bacterial protein FimH induced antibodies cross-reactive to LAMP-2 that are expressed on the surface of neutrophils; these antibodies to LAMP-2 cause pauci-immune focal necrotizing glomerulonephritis when injected into rats. As mentioned earlier, antibodies to human LAMP-2 were detected with a high prevalence (93%) in a cohort of patients with pauci-immune glomerulonephritis who were positive for PR3- or MPO-ANCA in only 50% of cases. Another study suggests a link between infection and AAV by showing that ANCA production could be induced solely by CpG stimulation of B cells isolated from the circulation of AAV patients ; further studies are needed to clarify the mechanism involved in this finding.
The focus of most other studies of animal models was to prove pathogenicity of ANCA rather than to elucidate the mechanisms of ANCA induction (see later discussion).
In the future, it will be essential to identify the exact conditions that are required to induce autoimmunity against ANCA antigens. The search for other potential target antigens and the revelation of how an autoantigen relates to a certain disease or disease phenotype will be central issues to elucidate the pathophysiology of AAV. The understanding of ANCA induction will offer new targets for therapeutic interventions that interfere with the inception of the disease, even before ANCA is induced.
Animal models to prove the concept of small-vessel vasculitis induced by ANCA
To date, an animal model supporting the pathogenicity of ANCA has been successfully developed for MPO-ANCA, but not for PR3-ANCA. Xiao and colleagues provided the first convincing model of immunity against MPO in which necrotizing vasculitis could be induced. Immunization of MPO-deficient mice with mouse MPO led to the induction of MPO-ANCA. Injection of MPO-ANCA into immunodeficient or wild-type mice generated pauci-immune focal necrotizing glomerulonephritis. A relatively small proportion of glomeruli were affected, which may be a consequence of the passive transfer of antibodies. When splenocytes from MPO-injected MPO-deficient mice were transferred into Rag2 −/− mice, they developed necrotizing crescentic glomerulonephritis that was more severe than that induced by the passive transfer of the antibody. In a second model, the passive transfer of MPO-ANCA was circumvented by immunizing MPO −/− mice with MPO followed by irradiation and transplantation of bone marrow from MPO +/+ mice. Engraftment resulted in pauci-immune necrotizing glomerulonephritis in all mice and pulmonary capillaritis in some mice. Furthermore, it was shown that neutrophil localization to the glomeruli is dependent on the C5a receptor. Murine models show that immunity to MPO can cause small-vessel vasculitis; it remains to be elucidated whether this occurs with or without cofactors.
PR3-ANCA–associated small-vessel vasculitis has yet to be induced in rodents, for unclear reasons (see Ref. for a review). Establishing models of immunity against PR3 are further complicated because human and murine PR3 are different in structure and their antibodies do not cross-react. Immunization of rats and mice with chimeric human/mouse proteinase 3 has been shown to induce autoantibodies to mouse PR3 and rat granulocytes, but did not cause vasculitis. In another model, the injection of PR3 into PR3-deficient mice induced antibodies to mouse PR3, but passive transfer of anti-mouse PR3 antibodies did not induce vasculitis. After injection of tumor necrosis factor (TNF) into the skin of PR3-immunized mice, a local infiltrate of inflammatory cells could be detected.
Additional studies need to dissect the pathophysiologic pathways of vasculitis induced by MPO/MPO-ANCA. Whether a model for PR3-mediated immunity will be successfully established remains unknown.
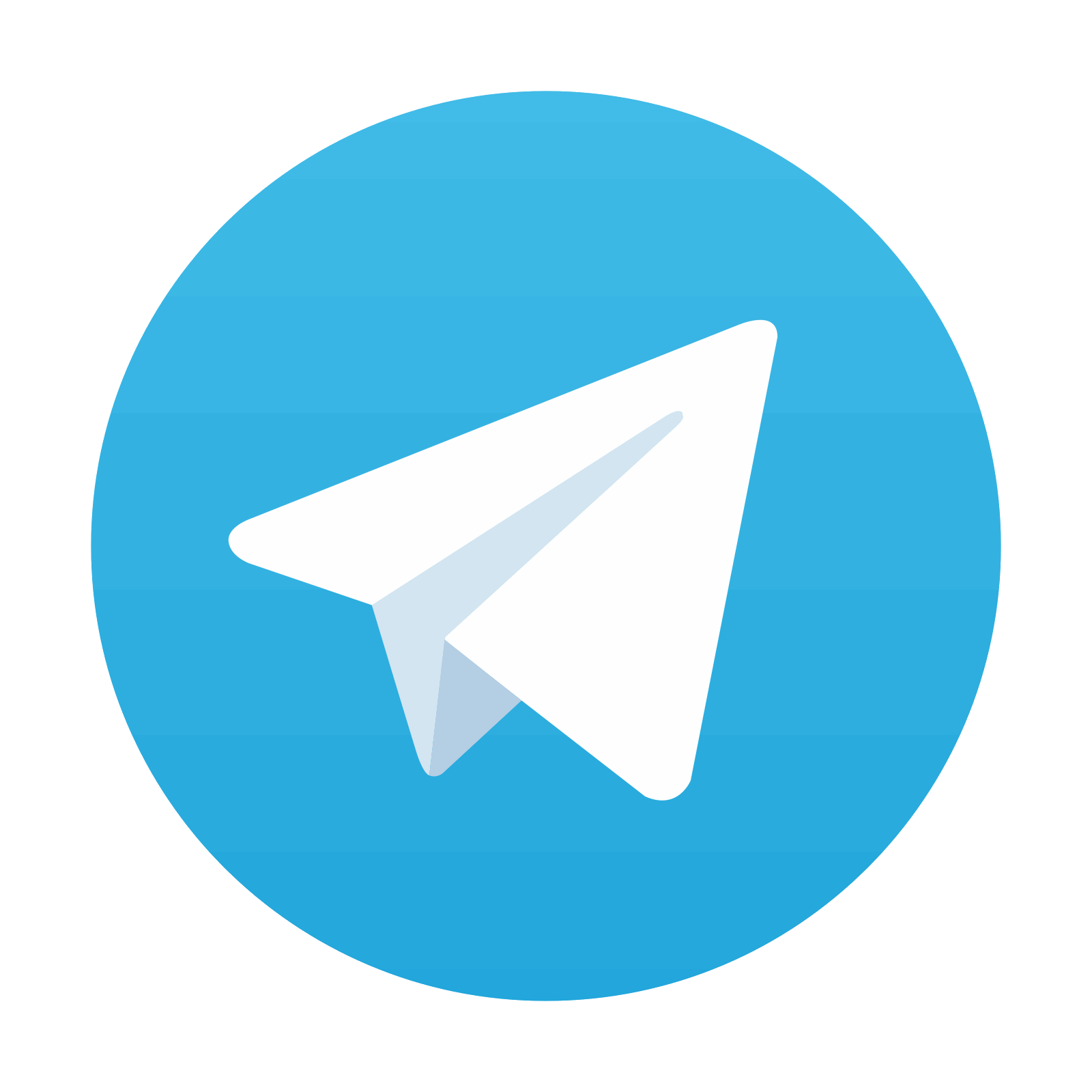
Stay updated, free articles. Join our Telegram channel

Full access? Get Clinical Tree
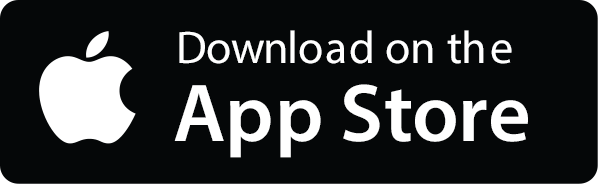
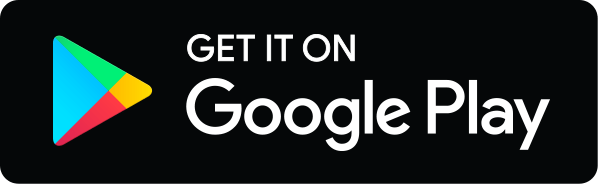