This article’s purpose is to explain the anatomicophysiologic basis for observations and measurements made by the electromyographer. An electromyographer may be trained in the techniques of electromyography without understanding the anatomicophysiology, and valuable interpretive subtleties may be missed. This article is an attempt to provide that background (ie, the link among anatomy, physiology, and electrodiagnostic findings). It is hoped that with a fuller understanding of the anatomicophysiologic basis of clinical electromyography, subtle observations can be made which will allow a more sophisticated level of interpretation of electromyography results. Such interpretations should improve the electromyographer’s capacity to provide useful information to a referring physician and hence, improve the management of a patient.
Electromyography (EMG) is the most valuable part of the electrodiagnostic medicine consultation. In training, electromyographers learn the characteristics of motor unit action potentials (MUAPs) in health and disease. They also learn the importance of identifying spontaneous potentials, such as positive sharp waves and fibrillation potentials, although in some training programs, electromyographers are taught that these potentials are one and the same. However, it may be that measuring MUAP morphology and firing rate and spontaneous activity of various types does not uncover all of the information that the EMG study might potentially yield. Often, an electromyographer memorizes facts about these potentials, but does not fully comprehend the underlying physiology. This article proposes that a thorough understanding of the underlying pathophysiology of the motor unit will enable an electromyographer to gather additional useful clinical information from an EMG study.
Spatial arrangement of the motor unit
A motor unit consists of a single anterior horn cell, ventral root, peripheral axon, terminal branches, myoneural junctions, and multiple muscle fibers. Fig. 1 shows a simplified drawing of the distal portion of a typical motor unit: the distal portion of a motor axon, its distal branches, and the individual muscle fibers innervated. In this drawing, the nerve, muscle fibers, and EMG needle are not drawn to scale. Throughout this article, this representation is used and modified to demonstrate graphically the changes occurring in the motor unit (and reflected by the MUAP) under various neuropathic conditions.
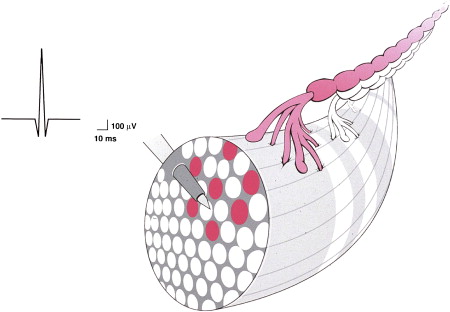
Components of the motor unit that are of interest are shaded gray. The muscle fibers innervated by the axon of interest are not contiguous. For centuries, ever since the motor unit was discovered and studied microscopically, it was assumed that all of the muscle fibers supplied by an anterior horn were contiguous. This assumption, which arose from pathologic observations of “fiber type grouping” , was reached by studying muscle tissue from patients who had neuropathic disorders. Histologic study of this muscle demonstrated groups of contiguous hypertrophic muscle fibers adjacent to other areas of small atrophic muscle fibers. Based on this, it was inferred that clumps of contiguous muscle fibers, each innervated by a single anterior horn cell, represented the natural state of motor unit organization.
However, one of the significant contributions of EMG to neurophysiology research occurred after WWII in the laboratory of Dr. Edward Lambert at the Mayo Clinic. One of his fellows, Dr. Murray Brandstater, a physical medicine and rehabilitation resident at that institution, had as his research project the determination of the spatial arrangement of muscle fibers in healthy muscle. These investigators teased apart motor root axons, severing all but one. They then applied a rapid electric stimulation proximal to the severed roots, consequently stimulating only one axon, and depleting the glycogen in the muscle fibers innervated by that one axon. Following this, they stained the muscle for glycogen, looking for the spatial distribution of glycogen depletion. What they found was that rarely were two muscle fibers of a motor unit in contact; they were spread out and interdigitated with muscle fibers of other motor units over a field that ranged from 5 to 10 mm . These muscle fibers are spatially disseminated, as in Fig. 1 .
The MUAP is made up of the summated action potentials of each muscle fiber innervated by a firing anterior horn cell. The muscle fibers closest to the needle tip (see Fig. 1 ; Fig. 2 , which show a variable distance between the innervated muscle fibers and the recording site) generate the largest component to the MUAP and the fibers further from the recording site generate a much smaller amplitude potential . Figs. 1 and 2 also show that some of the terminal branches are smaller in diameter and hence, conduct more slowly than others, thus producing an asynchronous firing of the muscle fibers. This asynchrony accounts for the duration of the MUAP (the muscle fibers firing soonest contribute to the beginning of the MUAP; those firing last contribute to the final portion).
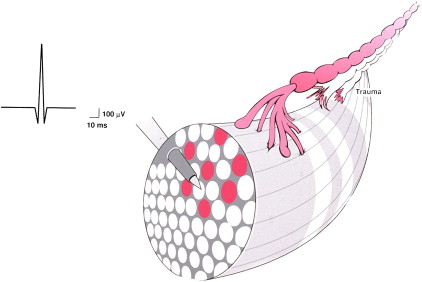
Acute partial denervation
When a peripheral nerve containing motor fibers is partially sectioned, the intact fibers will conduct impulses, producing activation of the still innervated muscle fibers. This situation is represented schematically in Fig. 2 , where the white axons are severed, allowing only the gray axon to function. As every electromyographer knows, in the normally innervated muscle, full contraction yields a full “interference pattern,” where MUAP activity is so great that the screen is filled with potentials and one cannot detect single MUAPs. However, in the example diagrammed in Fig. 2 , only one axon is functioning. Immediately after this nerve was partially severed, the remaining MUAP shows a normal configuration because the innervated muscle fibers still remain their normal size and distribution. (Compare the MUAP in Fig. 2 with that in Fig. 1 ).
In a normally innervated muscle, the lowest threshold motor units start firing at about 5 Hz. As the contraction becomes stronger, other units are recruited, starting with the lowest and moving to the highest threshold units, with the firing rates of all units increasing. But, because many of the axons are not functioning and the partially denervated muscle fibers are unable to contract, the electromyographer sees the existing MUAPs firing at a faster and faster rate. No additional MUAPs will be recruited. At full effort, if a sufficient number of axons have been severed, only a few MUAPs will be present and they will be firing at a very fast rate (up to 40 Hz). The MUAPs will have the characteristic appearance of a “picket fence,” as demonstrated in a tracing recorded under these conditions ( Fig. 3 ). In an acutely partially denervated muscle, MUAPs have normal amplitude, duration, and phasity because the anatomic distribution of the muscle fibers that are still innervated has not had enough time to be altered by the changes that inevitably occur following a partial nerve injury.
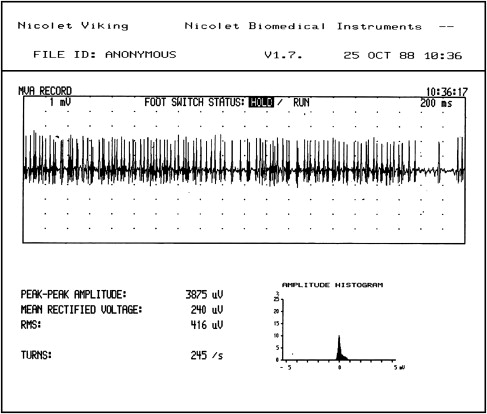
Acute partial denervation
When a peripheral nerve containing motor fibers is partially sectioned, the intact fibers will conduct impulses, producing activation of the still innervated muscle fibers. This situation is represented schematically in Fig. 2 , where the white axons are severed, allowing only the gray axon to function. As every electromyographer knows, in the normally innervated muscle, full contraction yields a full “interference pattern,” where MUAP activity is so great that the screen is filled with potentials and one cannot detect single MUAPs. However, in the example diagrammed in Fig. 2 , only one axon is functioning. Immediately after this nerve was partially severed, the remaining MUAP shows a normal configuration because the innervated muscle fibers still remain their normal size and distribution. (Compare the MUAP in Fig. 2 with that in Fig. 1 ).
In a normally innervated muscle, the lowest threshold motor units start firing at about 5 Hz. As the contraction becomes stronger, other units are recruited, starting with the lowest and moving to the highest threshold units, with the firing rates of all units increasing. But, because many of the axons are not functioning and the partially denervated muscle fibers are unable to contract, the electromyographer sees the existing MUAPs firing at a faster and faster rate. No additional MUAPs will be recruited. At full effort, if a sufficient number of axons have been severed, only a few MUAPs will be present and they will be firing at a very fast rate (up to 40 Hz). The MUAPs will have the characteristic appearance of a “picket fence,” as demonstrated in a tracing recorded under these conditions ( Fig. 3 ). In an acutely partially denervated muscle, MUAPs have normal amplitude, duration, and phasity because the anatomic distribution of the muscle fibers that are still innervated has not had enough time to be altered by the changes that inevitably occur following a partial nerve injury.
Motor unit action potential weeks after partial denervation
One can anticipate the next stage of this story. Following denervation, muscle fibers previously innervated by the severed axon undergo progressive atrophy, causing shrinkage of the volume and surface area . Simultaneously, the remaining innervated muscle fibers undergo hypertrophy as normal demands on the muscle now produce substantial resistive exercise on the few remaining muscle fibers. This situation is demonstrated in Fig. 4 , showing that the gray muscle fibers are now hypertrophic and that the white muscle fibers, representing denervated muscle, are now atrophic. So far, no terminal sprouting has occurred. Consequently, the motor unit still consists only of the originally innervated muscle fibers.
Because the amplitude of the individual muscle fiber action potential increases as the surface area of the muscle fiber enlarges , it would be expected that the summation of these hypertrophic muscle fibers would combine to produce a larger amplitude MUAP. In addition, with progressive atrophy of the intervening denervated muscle fibers, the innervated muscle fibers come closer to the recording needle, also contributing to greater MUAP amplitude . Indeed, such an occurrence is seen.
Motor unit action potential several months after partial denervation
A healthy motor unit attempts regeneration. The process is one of terminal sprouting, in which fine-diameter, poorly myelinated sprouts attempt to reinnervate adjacent denervated muscle fibers ( Fig. 5 ). Fig. 5 shows not only the large hypertrophic gray muscle fibers representing the original components of the motor unit of interest but also some gray (reinnervated) atrophic muscle fibers representing those previously denervated fibers that have now been captured by the distal sprouts.
Physiologically, these young sprouts have several characteristics. First, because they are very small in diameter, they conduct slowly. Consequently, electric activity transmitted by them to muscle fibers now captured occurs much later in time, which explains the long latency seen to satellite potentials in regenerating motor units. Second, the newly formed myoneural junctions are immature and do not fire every time an impulse is transmitted down the nerve, which can be seen on a delay line as intermittent blocking with variation in the amplitude and position of the polyphasic satellite potentials.
When the motor unit, now in a stage of early sprouting, fires, it appears as in Fig. 5 . The large main component of the MUAP (which may be the only one that can be identified on a conventional EMG without a delay line) is time linked to these “satellites,” typically accruing around 20 milliseconds after the primary potential.
In the months after this stage of terminal sprouting, through activity, the newly captured muscle fibers become larger and contribute a larger electric potential to the MUAP. Thus, the main MUAP becomes polyphasic as some of the previous satellite potentials are now firing within the time frame of the primary potential. As a result of these two factors, the MUAP becomes larger and more polyphasic, and the satellites are no longer present. This scenario is depicted in Fig. 6 .
