Clinical, experimental, and fundamental studies have shown the interest of a foreign body-induced membrane to promote the consolidation of a conventional cancellous bone autograft for reconstruction of long bone defects. The main properties of the membrane are to prevent the resorption of the graft and to secrete growth factors. The induced membrane appears as a biological chamber, which allows the conception of numerous experimental models of bone reconstruction. This concept could probably be extended to other tissue repair.
The reconstruction of wide long bone diaphyseal defects is often the major challenge in limb salvage whatever the etiology of bone loss. The most common and widely accepted procedures are the vascularized bone free transfer and the Ilizarov bone transport method. Bone autograft is not advocated when the defect is over 4 to 5 cm. When diaphyseal defects larger than 6 cm are reconstructed with autologous bone graft, healing is incomplete because of graft resorption even in a good vascularized muscular envelope. Experimental study and clinical experience concerning osteoperiosteal flaps were encouraging, but the limited sizes of harvested flaps on the human body incited the authors to abandon this technique of bone reconstruction
Since 1986, the authors’ routinely use a technique that has permitted the authors to elaborate the concept of induced membrane and to reconstruct large defects with nonvascularized bone autograft. Induced membrane is different from the bioresorbable polylactide membranes experimentally tested to treat critical size, segmental defects in rabbits or sheep.
The aim of this article is to present the membrane as a biological model, to compare the results of two clinical series, and to discuss the implications of the model.
Principles of technique
The reconstruction needs to have two different operative stages ( Fig. 1 ). The first stage is comprised of a radical debridement, a soft-tissue repair by flaps when needed, and the insertion of a polymethyl methacrylate (PMMA) cement spacer into the bone defect. The second stage is performed 6 to 8 weeks later, when the definitive healing of soft tissue is acquired. The spacer is removed, but the membrane that is induced by the cement is left in place Fig. 1 A. The cavity is filled up by morcellized cancellous bone autograft harvested from the iliac crests Fig. 1 B. Sometimes, when the amount of autograft is not sufficient or to spare an iliac crest, bone substitute (demineralized ox bone) is added to the cancellous bone according to a ratio that is not over 1:3.
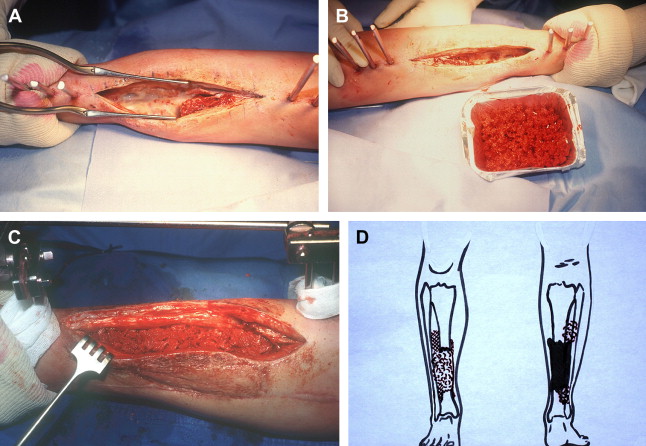
Several technical details should be emphasized. At the first stage, the cement must be wrapped around the bone extremities to allow detaching small pieces of the ends of the bone and lifting it with a bit of the induced membrane, at the second stage. When the bone graft is placed into the tube, the soft tissues, including the membrane, are sutured close to the graft resulting in a containment system Fig. 1 C. When treating a diaphyseal defect of the tibia, the cement is applied on the fibula as far as possible to obtain a very strong reconstruction. Moreover, at the second stage, the authors routinely perform an intertibiofibular graft at both extremities of the tibial defect, by a posterior approach Fig. 1 D. In reconstruction of the lower limb, the full weight bearing is usually authorized to patients at 5 to 6 months with the protection of the external fixator. Then the fixation is dynamized during 1 month and finally removed 1 month later. In 1990, the surprising results of the authors’ first cases incited them to undertake experimental and fundamental studies to elucidate the role of the membrane induced by the cement spacer. One of the main interrogations concerned the absence of resorption of the autograft.
The Foreign Body-induced Membrane
Initially, the role of the cement spacer was to avoid the collapse of the soft tissue into the bone defect and to prepare the bone reconstruction. Moreover, as most of the authors’ clinical cases were posttraumatic septic nonunions, the spacer was considered an excellent witness of successful debridement in the absence of recurrent infection after 2 months. The initial reason for which the authors’ did not excise the membrane was to prevent excessive bleeding. Finally, the main role of the cement is biological, by inducing a foreign-body surrounding membrane.
The first step of the investigation was to confirm the role of the membrane. Experimental study was done at the AO Development Institute of Davos and it was asserted that the membrane avoided the resorption of the cancellous bone and had a positive effect upon the healing of the autograft. Material comprised 30 sheep on which a segmental femoral defect, 3 cm in length, was created, filled up with a PMMA cement spacer, and stabilized with a plate. One month later, four groups were constituted after removing the spacer:
- •
Group A: the membrane was maintained and filled up with cancellous bone chips.
- •
Group B: the membrane was excised and the defect was filled up with cancellous bone chips.
- •
Group C: the membrane alone was maintained without filling.
- •
Group D: the membrane was excised and the defect was not filled up.
As expected, no bone formation was noted in groups C and D. In group B, bone healing was partially obtained with an important resorption in all cases. In group A, bone healing was acquired without reduction of the volume of the initial graft.
The second step of the investigation was to precise the role of the membrane by evaluating its histologic and biochemical characteristics. Histologic and immunochemistry studies were performed and the following data have been established:
- •
The membrane is richly vascularized in all its layers.
- •
The inner part (face to the cement) is a synovial like epithelium and the outer part is made of fibroblasts, myofibroblasts, and collagen.
- •
The membrane secretes growth factors: high concentration of VEGF and TGF Beta 1 were observed as early as the second week. BMP-2 is at its highest level at the fourth week.
Finally, membrane extracts stimulated bone marrow cell proliferation and differentiation to osteoblastic lineage.
Clinical experience showed that the cancellous bone inside the membrane is not submitted to resorption. As shown by a case report, after healing, macroscopic examination of transverse section of the healed bone graft exhibits normal bone anatomy, and the junction between the normal bone and the graft was difficult to see by macroscopic examination of longitudinal sections.
Retrospective clinical experience
Between 1986 and 1999, the authors did a series of 35 reconstructions of long bone segmental defects ranging from 5 to 24 cm after debridement. Lower limb was involved in 29 cases and the majority of cases were posttraumatic septic nonunions of the leg (23 cases) ( Figs. 2 and 3 ). Upper limb was concerned in six cases ( Fig. 4 ). Soft-tissue repair by flaps was needed in 28 cases (14 free flaps and 14 pedicled flaps). Immediate complications concerned the failure of the free flaps in three patients who were treated successfully by other techniques of reconstruction (Papineau and Ilizarov procedures). In the induced membrane and grafting procedure, bone healing was regularly obtained in a time that was independent of the length of reconstruction. In all cases the authors noted an aspect of radiological healing at 4 months. At the beginning of the authors’ experience, they performed additional grafts to reinforce the extremities of the reconstructed segment; but later the systematic petaling of the extremities permitted a primitive healing. At the lower limb, full weight bearing without protection was acquired in the mean time of 8.5 months (range: 6–17 months). Infection healed in all patients involved and that was probably in relation with the very radical initial debridement. In the series, the authors noted four stress fractures (2 early and 2 late) that healed by simple immobilization.
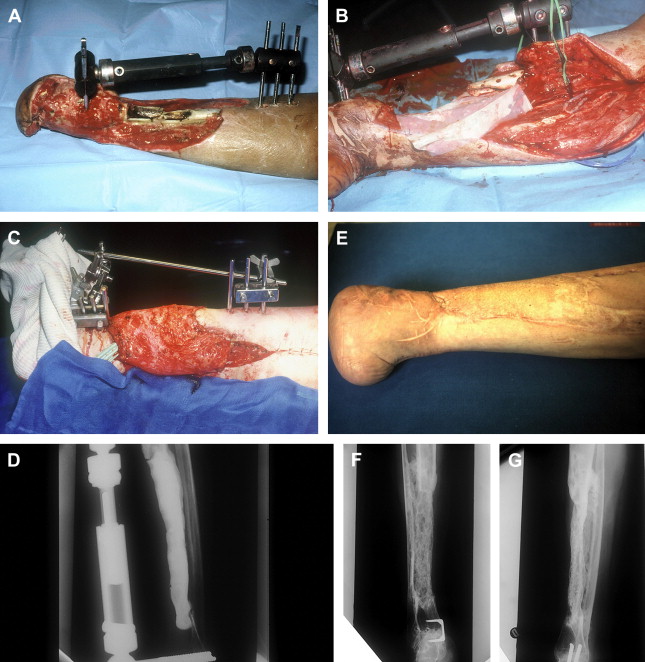
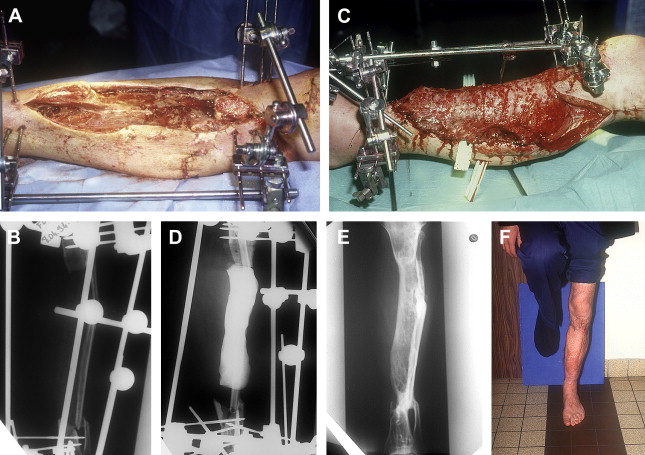
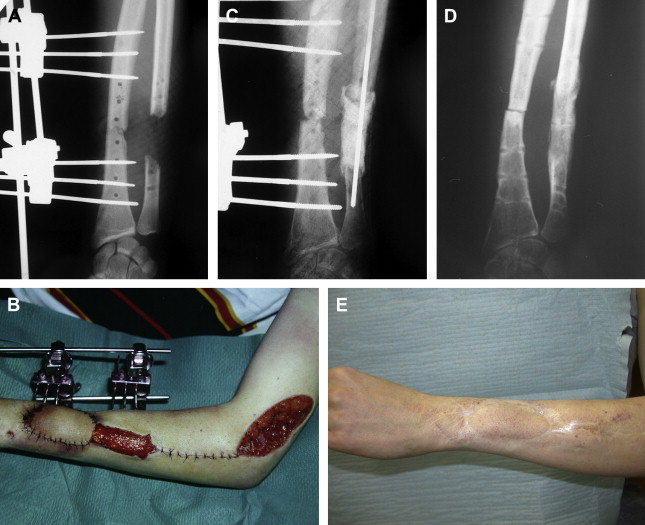
Retrospective clinical experience
Between 1986 and 1999, the authors did a series of 35 reconstructions of long bone segmental defects ranging from 5 to 24 cm after debridement. Lower limb was involved in 29 cases and the majority of cases were posttraumatic septic nonunions of the leg (23 cases) ( Figs. 2 and 3 ). Upper limb was concerned in six cases ( Fig. 4 ). Soft-tissue repair by flaps was needed in 28 cases (14 free flaps and 14 pedicled flaps). Immediate complications concerned the failure of the free flaps in three patients who were treated successfully by other techniques of reconstruction (Papineau and Ilizarov procedures). In the induced membrane and grafting procedure, bone healing was regularly obtained in a time that was independent of the length of reconstruction. In all cases the authors noted an aspect of radiological healing at 4 months. At the beginning of the authors’ experience, they performed additional grafts to reinforce the extremities of the reconstructed segment; but later the systematic petaling of the extremities permitted a primitive healing. At the lower limb, full weight bearing without protection was acquired in the mean time of 8.5 months (range: 6–17 months). Infection healed in all patients involved and that was probably in relation with the very radical initial debridement. In the series, the authors noted four stress fractures (2 early and 2 late) that healed by simple immobilization.
