Neurologic rehabilitation interventions may be either therapeutic or compensatory. Included in this article are lower extremity functional electrical stimulation, body weight–supported treadmill training, and lower extremity robotic-assisted gait training. These poststroke gait training therapies are predicated on activity-dependent neuroplasticity. All three interventions have been trialed extensively in research and clinical settings to show a positive effect on various gait parameters and measures of walking performance. This article provides an overview of evidence-based research that supports the efficacy of these three interventions to improve gait, as well as providing perspective on future developments to enhance poststroke gait in neurologic rehabilitation.
Key points
- •
Poststroke gait training interventions may be either therapeutic, resulting in enhanced motor recovery, or compensatory, whereby assistance or substitution for neurologic deficits results in improved functional performance.
- •
Functional electrical stimulation, body weight–supported treadmill training, and robotic-assisted gait training are examples of poststroke gait training therapies.
- •
Poststroke gait training therapies are predicated on activity-dependent neuroplasticity, which is the concept that cortical reorganization following central nervous system injury may be induced by repetitive, skilled, and cognitively engaging active movement.
- •
Additional research is necessary to determine whether specific technology-enhanced gait training methods are superior to conventional gait training methods.
Introduction
This article provides a comprehensive review of specific rehabilitation interventions used to enhance hemiparetic gait following stroke. Neurologic rehabilitation interventions may be considered either therapeutic or compensatory. Poststroke therapeutic interventions may improve gait performance by various motor relearning mechanisms including improved motor strength or enhanced motor control. Motor relearning is defined as the recovery of previously learned motor skills that have been lost following localized damage to the central nervous system. Compensatory interventions may improve gait performance by providing assistance or by substituting for neurologic deficits to improve function. The specific spatiotemporal, kinematic, and kinetic parameters of gait that distinguish hemiparetic gait from other upper motor neuron gait patterns are described elsewhere in this issue. Included in this article are specific clinical and research applications of rehabilitation interventions that may enhance poststroke gait, including lower extremity functional electrical stimulation (FES), body weight–supported treadmill training (BWSTT), and lower extremity robotic-assisted gait training. These 3 poststroke gait training therapies are all predicated on activity-dependent neuroplasticity, which is the concept that cortical reorganization following central nervous system injury may be induced by repetitive, skilled, and cognitively engaging active movement. This article does not cover spasticity management, lower extremity orthotics, or specific physical therapy (PT) therapeutic treatments such as neurodevelopmental technique (NDT). Instead, this article provides a concise overview of evidence-based research that supports the efficacy of the 3 interventions mentioned earlier, as well as providing perspective on future developments to enhance poststroke gait in neurologic rehabilitation.
Introduction
This article provides a comprehensive review of specific rehabilitation interventions used to enhance hemiparetic gait following stroke. Neurologic rehabilitation interventions may be considered either therapeutic or compensatory. Poststroke therapeutic interventions may improve gait performance by various motor relearning mechanisms including improved motor strength or enhanced motor control. Motor relearning is defined as the recovery of previously learned motor skills that have been lost following localized damage to the central nervous system. Compensatory interventions may improve gait performance by providing assistance or by substituting for neurologic deficits to improve function. The specific spatiotemporal, kinematic, and kinetic parameters of gait that distinguish hemiparetic gait from other upper motor neuron gait patterns are described elsewhere in this issue. Included in this article are specific clinical and research applications of rehabilitation interventions that may enhance poststroke gait, including lower extremity functional electrical stimulation (FES), body weight–supported treadmill training (BWSTT), and lower extremity robotic-assisted gait training. These 3 poststroke gait training therapies are all predicated on activity-dependent neuroplasticity, which is the concept that cortical reorganization following central nervous system injury may be induced by repetitive, skilled, and cognitively engaging active movement. This article does not cover spasticity management, lower extremity orthotics, or specific physical therapy (PT) therapeutic treatments such as neurodevelopmental technique (NDT). Instead, this article provides a concise overview of evidence-based research that supports the efficacy of the 3 interventions mentioned earlier, as well as providing perspective on future developments to enhance poststroke gait in neurologic rehabilitation.
Functional electrical stimulation for gait training
Neuromuscular electrical stimulation (NMES) refers to the activation of paretic or paralyzed muscles via stimulation of an intact lower motor neuron (LMN). The term FES was originally coined by Moe and Post to describe the use of NMES to activate paretic muscles in a magnitude and sequence that directly facilitates a functional task such as walking. A lower extremity neuroprosthesis is an FES device or system designed to enhance functional walking, and the term neuroprosthetic effect describes the enhancement of walking performance that results when the neuroprosthesis is used. This effect may be evident by improved gait efficiency (for example, energy expenditure) or by improved spatiotemporal, kinematic, or kinetic parameters of gait (for example, walking velocity, paretic ankle dorsiflexion angle at heelstrike, or paretic plantarflexion power at push-off). Evolving basic science and clinical studies on central motor neuroplasticity support the role of active repetitive movement training of a paretic limb to enhance motor relearning, and thus lower extremity FES applications have also been proposed for therapeutic purposes in hemiparesis. The rationale for a therapeutic role of FES is that, if active repetitive movement training facilitates motor recovery, then, theoretically, FES-mediated repetitive movement training may also facilitate poststroke motor recovery. A recent review of the clinical and research applications of NMES in neurorehabilitation includes an overview of FES neurophysiology, FES componentry, and therapeutic applications of research and commercially available FES devices.
Neurophysiology of FES
Most clinical FES applications are limited to patients with upper motor neuron dysfunction, because FES depends on the LMN being intact. Either the motor point of the nerve proximal to the neuromuscular junction or the peripheral nerve itself can be directly stimulated by clinical and research-based FES systems. The threshold for eliciting a nerve fiber action potential is 100 to 1000 times less than the threshold for muscle fiber stimulation. An action potential (AP) induced by an FES system is identical to the all-or-none phenomenon of the AP produced by natural physiologic means. However, an AP produced by normal physiologic mechanisms initially recruits the smallest diameter neurons before recruitment of larger diameter fibers, such as α motor neurons. The nerve fiber recruitment pattern induced by FES differs from a physiologic AP by following the principle of reverse recruitment order. Reverse recruitment order means that the nerve stimulus threshold is inversely proportional to the diameter of the neuron. Thus, large-diameter nerve fibers, which innervate large motor units, are recruited preferentially. Adjustment of stimulus parameters such as stimulus amplitude, pulse width, and frequency thus affect both nerve fiber recruitment and resultant muscle contraction force characteristics. The minimum stimulus frequency that can generate a fused muscle response is approximately 12.5 Hz. Although stimulation frequencies higher than 12.5 Hz can generate higher muscle forces, there is a risk of muscle fiber fatigue and resultant decrease in muscle contractile force. An efficient FES system is designed to use the minimum stimulus frequency that produces a fused response, with ideal stimulation frequencies ranging from 18 to 25 Hz for lower limb applications.
Components of FES Systems
Most research and commercially available FES systems for neurorehabilitation are in 2 broad categories based on electrode type: transcutaneous (surface) electrode and implanted electrode systems. A transcutaneous electrode is applied directly to the skin and stimulates either the peripheral nerve or the motor point of the underlying muscle. A transcutaneous electrode is the simplest electrode available and uses an external lead to connect directly to the neurostimulator device. An electrical current is created when 2 electrodes are placed in either a monopolar or bipolar configuration in relation to each other. The risk of tissue injury in patients with cognitive and/or sensory deficits may be increased with a transcutaneous electrode system and thus specific neurologic deficits must be evaluated before use. Other common limitations associated with transcutaneous electrode systems in patients who have had strokes include intolerance caused by activation of cutaneous pain receptors, poor muscle selectivity, inconsistency with electrode positioning, insecure fixation on a moving limb, skin irritation associated with the electrode, and cosmetic concerns.
The percutaneous intramuscular electrode is a type of implantable electrode. The placement of a percutaneous electrode is a minimally invasive procedure; however, lead wires that exit the skin are required to connect the electrode to the neurostimulator. The advantages and disadvantages of the percutaneous electrode have been well described previously. The advantages of the percutaneous electrode are the elimination of skin resistance and cutaneous pain issues, greater muscle selectivity, and lower stimulation currents. Safety risks include lead displacement or breakage, infection, and granuloma formation associated with retained electrode fragments. The cumulative long-term failure rate of percutaneous electrodes varies between 56% and 80%, which generally limits their use to less than 3 months. As a result, the most common applications of percutaneous electrodes are for time-limited therapeutic purposes or research applications.
Epimysial, epineural, intraneural, and cuff electrodes are all types of surgically implanted electrodes that may be used for long-term neurostimulation applications. These electrodes are surgically placed above the muscle, above the nerve, within the nerve, or around the nerve, respectively. These types of implanted electrodes all connect to implanted lead wires and require the implantation of the neurostimulator. A radiofrequency (RF) telemetry link relays both power and command instructions to the neurostimulator from an external control unit. An implanted peroneal nerve stimulation (PNS) device is an example of a clinically viable neuroprosthesis that uses implantable electrodes.
Correlating user intent to functional performance, particularly in the application of a lower extremity neuroprosthesis, presents significant challenges in the design of FES control systems. Many upper extremity clinical FES systems use open-loop control (preset pattern of stimulation) with sensory feedback limited to residual visual and proprioceptive input. A closed-loop control system for continuous real-time modification of the stimulation pattern based on sensory feedback offers maximal advantage for a lower extremity neuroprosthesis. A transcutaneous PNS device is an example of a neuroprosthesis that uses a closed-loop control system such as a simple heel switch, tilt sensor, or acceleration sensor.
Lower Extremity FES in Hemiparesis
The initial application of a neuroprosthesis in hemiparesis was the transcutaneous PNS device for correction of ankle dorsiflexion weakness associated with upper motor neuron dysfunction. In a 1961 publication, Lieberson first described a stimulator that dorsiflexed the ankle during the swing phase of gait. Burridge and colleagues later reported that chronic stroke survivors treated with PNS and PT had a significant increase in walking velocity and decrease in energy expenditure measured by the physiologic cost index compared with a control group who received PT only. The application of surface PNS has more recently been proposed as an alternative to an ankle-foot orthosis (AFO) based on other favorable neuroprosthetic effects of PNS. Studies of hemiparetic subjects treated with neuroprosthetic applications of PNS have also reported possible therapeutic effects including enhanced walking speed, increased maximal isometric contraction of the ankle dorsiflexors and plantarflexors, increased dorsiflexion torque, increased agonist electromyogram (EMG) activity and decreased EMG cocontraction ratios, increased maximum root mean square (measure of muscle output capacity), decreased calf spasticity, and improved ankle control, outcomes that were all measured while the subject was not wearing the device. Studies that have specifically compared the neuroprosthetic effect of a PNS with the orthotic effect of an AFO in hemiplegic gait have also facilitated broader clinical prescription and usage of these devices.
The US Food and Drug Administration (FDA) has approved 3 surface PNS devices for clinical use in the United States. These devices are the Odstock Dropped Foot Stimulator (ODFS; Odstock Medical Limited, Salisbury, UK; Fig. 1 ), Ness L300 Footdrop System (Bioness Inc, Valencia, CA; Fig. 2 ), and the WalkAide System (Innovative Neurotronics, Austin, TX; Fig. 3 ).
The ODFS also carries the CE mark of approval by the European Union. The ODFS and the Ness L300 device use heel switches and the WalkAide device uses a tilt sensor as a control system to time stimulation to the swing phase of gait. The clinical use of these devices may be limited in patients who have had strokes who have skin breakdown or edema, lower extremity sensory deficits, cognitive deficits, ankle range-of-motion limitations, plantarflexion spasticity, cerebellar ataxia, and/or muscle fatigue. Common reasons for lack of efficacy of a surface PNS device include patient intolerance to surface stimulation, difficulty with electrode placement, insufficient medial-lateral control during stance phase, persistent genu recurvatum, and/or lack of rehabilitation support staff to assist with device programming.
The technical limitations of transcutaneous devices may ultimately be solved with the further refinement of implantable neurostimulation systems. Early studies first reported improvements in spatiotemporal gait parameters following implantation of a single-channel PNS device. Although an implantable PNS device is not presently FDA approved in the United States, multichannel implantable PNS devices have recently undergone clinical investigation in Europe. A dual-channel device developed at the University of Twente and Roessingh Research and Development (The Netherlands) stimulates the deep and superficial peroneal nerves for better control and balance of ankle dorsiflexion, eversion, and inversion. A 4-channel device, developed at Aalborg University (Denmark) uses a nerve cuff electrode with 4 tripolar electrodes, oriented to activate different nerve fibers within the common peroneal nerve. Both devices have received the CE mark approval in the European Union and clinical experience with these devices is now being reported. Recent clinical trials that have evaluated the efficacy of an implantable PNS device report a neuroprosthetic effect on walking speed, paretic double support and nonparetic single support, and ankle dorsiflexion angle during swing. No PNS therapeutic effect was found in a study that compared 6 months of ambulation using an implantable, 2-channel peroneal nerve stimulator with an AFO ; however, increased voluntary muscle output of the tibialis anterior and gastrocnemius/soleus muscles in the PNS group were hypothesized to be evidence of neuroplasticity.
Several multichannel transcutaneous stimulation systems have been trialed that incorporate knee and hip flexion and extension as well as ankle dorsiflexion. The rationale for these more complex stimulation systems is that poststroke gait deviation associated with hemiplegia is not limited to ankle dysfunction or remedied by correction of footdrop alone. However, lower limb transcutaneous systems are particularly limited by reduced muscle selectivity, poor consistency of stimulation, and discomfort associated with sensory stimulation of larger, weight-bearing lower extremity muscles. From a clinical standpoint, as the number of electrodes increases, transcutaneous systems are increasingly difficult to clinically implement. However, commercially available PNS devices including the Ness L300 Plus System and the Odstock 2 Channel Stimulator device have recent modifications that allow activation of additional muscles groups such as the quadriceps and/or hamstring muscles. Multichannel percutaneous lower limb systems remain limited to research applications or time-limited therapeutic interventions intended to facilitate motor relearning as opposed to providing a longer-term neuroprosthetic solution.
Summary
A transcutaneous PNS device may be an appropriate clinical alternative to an AFO in select patients who have had hemiparetic strokes. Neurologic and medical issues that affect patient safety need to be considered and a device trial under the guidance of a trained physical therapist or other rehabilitation professional is recommended before device prescription. Additional practical issues, including access to long-term technical support and comparative device (PNS vs AFO) costs should also be considered. Additional research is necessary to determine the clinical efficacy of a 2-channel transcutaneous PNS device that incorporates quadriceps and/or hamstring stimulation. Clinical experience outside the United States suggests that an implantable PNS device may be a viable neuroprosthesis for select patients. However, potential benefits must be tempered with the risks and costs associated with an invasive procedure. At present, multichannel percutaneous lower limb FES systems remain limited to research and/or time-limited therapeutic applications. In addition, research is increasingly focusing on new technologies, such as harnessing cortical control signals, that may provide an enhanced means of interfacing with a neuroprosthesis, and developing multichannel networked implantable neuroprostheses systems that may someday address both upper and lower limb paralysis associated with central nervous system injury.
Body weight-supported treadmill training for gait training
BWSTT is an adaptive therapeutic training modality that allows otherwise nonambulatory or limited ambulatory patients who have had hemiparetic strokes to participate in task-specific gait training. Patients are fitted with harnesses that provide partial support of their full body weight as they are suspended over the treadmill surface. With the assistance of 1 or several physical therapists to facilitate paretic lower limb placement, balance, and limb sequencing, the patient is able to participate in repetitive gait training exercises while upright and partially weight bearing. The primary advantage of any BWSTT method is that the patient is able to safely be upright with limited physical assistance, which allows the treating physical therapist to observe and correct the gait pattern to improve functional performance. Overground walking with partial body weight support (BWS), without the use of a treadmill, has been shown to improve walking in patients who have had hemiparetic strokes. In a recent study, self-selected walking speeds over a 15-m walkway increased 17% on average when hemiparetic subjects walked with some level of BWS compared with the no-BWS condition. Oxygen consumption (as measured by maximal oxygen uptake [V o 2 ]) and heart rate were improved at both self-selected and maximum walking speeds during walking with 30% BWS on a treadmill compared with unsupported treadmill walking. Hesse and colleagues evaluated the gait patterns of hemiparetic subjects walking on a treadmill with 0%, 15%, and 30% BWS compared with overground walking. BWSTT was associated with a more prolonged single-stance period of the paretic limb, greater gait symmetry, less plantar flexor spasticity, and a more regular activation pattern of the gastrocnemius/soleus and anterior tibialis muscles compared with floor walking. Compared with overground walking or overground training using partial BWS, BWSTT may allow the therapist to achieve a more symmetric, efficient hemiparetic gait pattern within a more practical physical space.
Clinical Application of BWSTT Gait Training
Commercially available body weight–supported devices may have both overground and treadmill capability for use in a therapy gym or home setting. Examples of such devices include the body-weight support mechanism of the Lokomat System (Levi BWS, Hocoma, Zurich, Switzerland), the LiteGait device (LiteGait, Tempe, AZ), and the Gait Trainer 3Biodex Gait Training System (Biodex Medical Systems, Inc, Shirley, NY). The prescription of specific parameters including percent body-weight support, speed of the treadmill, support stiffness, and handrail hold can affect treatment outcomes in hemiparetic patients. Thus, there may be significant variability in the application of the BWS gait training system and training protocols between clinical settings. In a study of the effect of treadmill speed used during BWS gait training, the greatest improvement in self-selected walking velocity occurred with fast training speeds, thus training at speeds comparable with normal walking velocity may be more effective than training at lower speeds. Specific objective clinical targets, such as heart rate and perceived exertion, can be achieved and/or monitored during BWSTT locomotor training. Patient ease of use has also been reported, as reflected in a recent study that showed that stable walking using BWSTT, as measured by spatiotemporal gait parameters including cadence, step length, and trunk symmetry, was achieved within a 5-minute walking trial.
BWSTT Gait Training and Poststroke Neuroplasticity
Recent research has focused on determining whether BWSTT has an effect on poststroke neuroplasticity. A therapeutic benefit by neuroplastic mechanisms is largely predicated on research in spinal cord injury that has shown the malleability of the CNS after injury in response to locomotor training. Central pattern generators (CPG), neuronal circuitry located within the spinal cord, produce the coordinated activation of flexor and extensor motoneurons during locomotion and segmental reflex pathways control the basic operation of the CPG circuitry responsible for locomotion. However, supraspinal input to the spinal circuitry is essential for both human and animal walking. Locomotor training has been shown to change the excitability of simple reflex pathways as well as more complex circuitry, and adaptation occurs at both spinal and supraspinal levels in animals following spinal lesions. Beneficial therapeutic effects of early sustained locomotor training after both central or peripheral lesions, based on evidence of plasticity of spinal and supraspinal locomotor control mechanisms, has thus been advocated.
Researchers have used various neuroimaging modalities to test the hypothesis that BWSTT may influence poststroke central neuroplasticity. Enzinger and colleagues applied an ankle dorsiflexion functional magnetic resonance imaging paradigm to relate brain activity changes in subjects with chronic stroke to performance gains after 4 weeks of BWSTT gait training. At the end of treatment, walking endurance, as measured by the 2-minute walking distance, improved and clinical performance correlated to an increase in brain activity in the bilateral primary sensorimotor cortices, the cingulate motor areas, and the caudate nuclei bilaterally, and in the thalamus of the affected hemisphere. The investigators concluded that, despite subcortical contributions to gait control, walking improvements secondary to BWSTT gait training were associated with cortical activation changes. Another study used an optical imaging system to evaluate the effect of BWSTT on cortical activation during gait in hemiparetic subjects compared with controls. Treatment with BWSTT in the hemiparetic subjects was associated with changes in activation in the sensorimotor cortex (SMC) that correlated with cadence ( P <.05). Improvement of asymmetry in SMC activation also correlated with improvement of asymmetric gait ( P <.05). The investigators concluded that BWSTT might improve the efficacy of SMC function in patients with stroke. Further research is necessary to determine whether poststroke BWSTT therapy has central effects and whether those effects translate into clinically significant change in motor impairment and/or functional mobility outcome measures.
Clinical Efficacy of Poststroke BWSTT Gait Training
Multiple studies have suggested beneficial effects of BWSTT gait training in hemiparetic patients on outcomes including walking speed, balance, walking capacity, motor recovery, and patient perception of walking ability. Visitin and colleagues compared the effects of BWSTT gait training (40% weight support) with treadmill gait training without BWS. After 6 weeks of training, the BWSTT group scored significantly higher on functional balance, motor recovery, overground walking speed, and overground walking endurance outcome measures. At 3 months after treatment, the BWSTT group continued to score significantly higher than the control group on walking speed and motor recovery measures. In a study that stratified subjects according to initial overground walking speed, endurance, balance, and motor recovery, Barbeau and Visintin found that 6 weeks of BWSTT training resulted in greater walking speed, endurance, functional balance, and motor recovery change than did gait training without BWS. BWSTT was more beneficial to subjects who were older or had a greater baseline level of gait impairment. A recent study explored which kinematic and kinetic gait parameters were most associated with improved walking speed in hemiparetic subjects who were responsive to a 6-week BWSTT program. Subjects who improved their self-selected walking speeds by greater than 0.08 m/s showed greater increases in terminal stance hip extension, hip flexion power, and intensity of soleus muscle EMG activity during walking.
Although beneficial effects have been reported, a Cochrane Review and several recent randomized controlled trials show that the superiority of BWSTT gait training compared with standard-of-care gait training interventions has not been established. In a study that compared BWSTT with conventional overground gait training in nonambulatory subacute (<6 weeks after stroke) subjects who had had strokes, Franceschini and colleagues reported that walking speed and capacity, functional ambulation performance, and balance were significantly improved in both groups at the end of 4 weeks of treatment and at the 6-month follow-up. Nilsson and colleagues similarly found no statistically significant difference in Functional Independence Measure score, walking speed, Fugl-Meyer score, and the Berg Balance score gains at end of treatment and at the 10-month follow-up in acute/subacute subjects who hah had strokes randomized to receive either BWSTT or overground walking training. More recently, Duncan and colleagues reported on the Locomotor Experience Applied Poststroke (LEAPS) trial, which is the largest study to date to evaluate the efficacy of BWSTT for poststroke gait training. The LEAPS trial enrolled 408 subjects who had had subacute hemiparetic strokes (<2 months after cerebrovascular accident) and stratified the subjects by level of walking impairment. The subjects were then randomized into 1 of 3 gait training groups: (1) BWSTT starting at 2 months after stroke (early locomotor training); (2) BWSTT starting at 6 months after stroke (late locomotor training); and (3) home exercise program (home exercise) starting at 2 months after stroke. The primary outcome measure was the proportion of subjects who had improved functional walking ability at 1 year after stroke. At 1 year, all 3 groups had similar improvements in walking speed, motor recovery, balance, functional status, and quality of life. The investigators concluded that gait training that included the use of BWSTT was not superior to progressive exercise at home managed by a physical therapist. Based on current evidence, Dobkin and Duncan recently concluded that BWSTT does not lead to better outcomes than a comparable dose of progressive overground training after a stroke and should not be routinely provided to stroke survivors in place of conventional therapy.
Summary
BWSTT is a gait training modality that allows otherwise nonambulatory or limited ambulatory patients who have had hemiparetic strokes to participate in task-specific gait training. BWSTT gait training allows a hemiparetic patient to safely weight bear with limited physical assistance while the treating physical therapist focuses on optimizing the gait pattern. BWSTT systems are available for clinical use and are routinely incorporated into poststroke clinical gait training programs. Research supports a beneficial effect of BWSTT on hemiparetic walking speed and capacity, functional balance, and poststroke functional ambulation. Current research is focused on both identifying a neuroplastic mechanism that may explain a therapeutic effect of BWSTT gait training and combining BWS methods with other rehabilitation modalities, such as FES, to maximize poststroke gait rehabilitation outcomes. However, recent randomized controlled clinical trials have not established the superiority of BWSTT compared with other conventional poststroke gait training interventions.
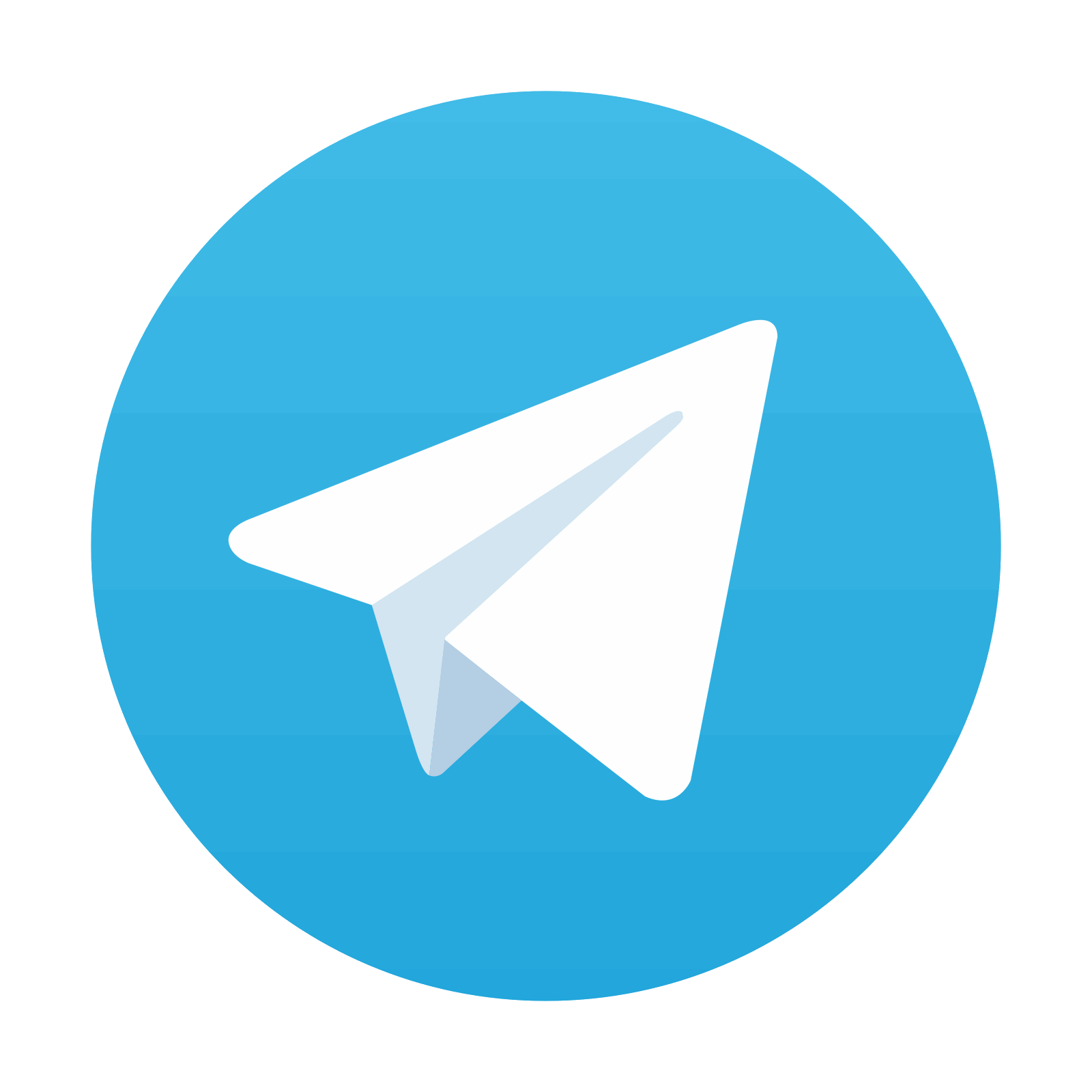
Stay updated, free articles. Join our Telegram channel

Full access? Get Clinical Tree
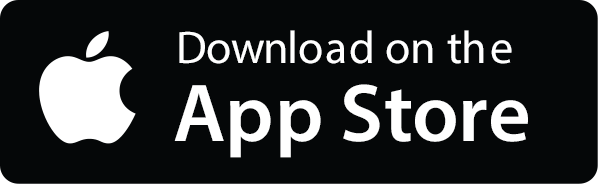
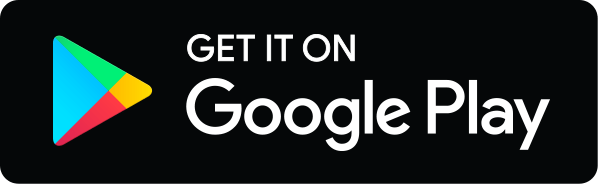