Articulation techniques in the treatment of pain and movement
In this chapter we will discuss the biomechanics and individual components of movement. We will present a general overview but will concentrate in particular on synovial joints, which are the joints that manual therapists engage with most.
Movement and stress
Human joints have to withstand considerable forces day to day from compression, shearing and tension. To do this they have evolved layers of tissues and mechanisms to protect against and dissipate the forces that daily activities generate.
Movement and stress always result in mechanical deformation of tissues and structures in the body because tissues undergo an elastic deformation when they are stretched. This deformation allows the tissue to rebound to its original shape and length if it is stretched within normal limits. However, if the tissue is stressed beyond its physical limitations of the elastic phase, it will fail to return to its original structure and will be lengthened. We call this the plastic phase of deformation (Threlkeld 1992).
Stressing the tissue beyond its maximum capacity will result in a tear of that tissue. It is, however, important to note that joint tissues typically work in the elastic phase of tissue deformation as this is vital for normal tissue homeostasis. Not undergoing a healthy deformation within the elastic phase because of lack of activity or immobilization will inhibit joints from regenerating optimally. The end result is tissue thickening, insufficient water in the tissue matrix, loss of tissue elasticity, collagen cross-bridging and shortening of tissue (Threlkeld 1992). There will be a loss of range of motion of joints and the patient will experience joint stiffness and difficulty in moving.
Joint articulation techniques which involve movements that exceed the natural motion barrier stress the tissues excessively and force them into the plastic phase of deformation and so should be avoided.
Movement and tissue repair
Healthy movement through the joint tissues can aid tissue repair and recovery. Khan and Scott (2009) discuss the theory of mechanotransduction and its stages of mechanocoupling, cell–cell communication and effector cell response. Mechanotransduction is described as ‘the process whereby cells convert physiological mechanical stimuli into biochemical responses’ (Khan & Scott 2009) or ‘the mechanical and structural cues that encourage cell behaviour’ (Ingber 2006). An example is the effect on bone mineral density in astronauts living in zero-gravity conditions and the reintroduction of gravity and loading to the skeletal system upon their return to Earth. This area is of interest because it can help our understanding of how the mechanical forces involved in distorting and stressing tissues can influence healing and promote tissue recovery by affecting their chemical structure. It also helps us to understand how poor mechanical forces can have the opposite effect, converting mechanical energy into biochemical energy. Manual therapy can aid mechanotherapy by enabling the patient to return to physical activity as quickly as possible and promoting healthy cellular homeostasis (Huang et al 2013).
Joint articulation is commonly used as a technique in manual therapy that has been shown to be effective at reducing musculoskeletal pain (Shum et al 2012). Articulations are often used with the objective to increase the range of motion of the joint through stretching of fibrous tissue and by affecting the stretch reflex excitability (Cook 2007). One theory is that repetitive movement and stretching may cause the tissue to elongate (Refshauge & Gass 2004). This elongation relates to the ability of tissues to change shape over time as a constant load is applied (Threlkeld 1992). It is thought that this effect on the tissues can increase the range of motion at the joint (McCollam & Cindy 1993, Arnoczky et al 2002, Powers et al 2008, Shum et al 2012). In addition, rhythmical movement causes a pumping action within the joint which influences the intra-articular pressure (Rice & McNair 2009), improving quality and quantity of motion. Saunders and Saunders (2004) demonstrated that articulation of the joint can also increase the vascular circulation to and from the joint during and after treatment. Bortnem and Zavertnik (2009) hypothesized that this may assist in the removal of metabolic waste products and help to balance pH levels within the joint, thus aiding the health, stability and integrity of the joint.
Given the nature of the loading, the pressure inside synovial joints and the need to achieve a low-friction environment, it is important to develop the optimum mechanical conditions after an injury to aid a joint’s recovery (Buckwalter 1998). The application of early controlled movement through a joint can aid recovery and also prevent degenerative changes to the articular surfaces (Buckwalter 1995, Buckwalter et al 1996). Buckwalter (1995) also postulated that early mobilization of the joint stimulated the recovery of the proteoglycan matrix, a theory supported by the work of Williams and colleagues (1994). Although getting patients to return to activity has been demonstrated to help articular cartilage recovery (Salter 1993, Mankin & Buckwalter 1997), loading the cartilage too soon can cause delayed recovery in the cartilage (Williams & Brandt 1984, Sun 2010). It is also suggested that the early introduction of movement helps in fluid drainage, preventing the build-up of fluid and distension forces upon the tissues and joint (Lederman 1997). In addition, prolonged distension of a joint caused by effusion could potentially decrease the blood supply to the synovial membrane, leading to damage of the chondrocytes and thus the articular cartilage (Geborek et al 1989, Lafeber et al 1992, Rice & McNair 2009). Beattie et al (2009) found that articulation of L5/S1 caused an increase in water diffusion at the associated intervertebral disc. It was postulated that an increase in diffusion to the intervertebral disc would cause an increase in intradiscal cell activity and elevated oxygen levels, thus increasing the formation of collagen and proteoglycans within the disc. Although these effects were noted to happen several hours after articulation of the joint, they would still aid in the health and structural stability of the disc.
Joint articulation has been shown to influence the sympathetic nervous activity of the body, thus affecting blood flow, blood pressure, heart rate or respiratory rate (Jowsey & Perry 2010, Zusman 2011, Moutzouri et al 2012, Kingston et al 2014). These sympathetic responses may be associated with changes in blood component levels caused by articulation reducing inflammatory proteins (Teodorczyk-Injeyan et al 2006).
Thus early articulation and management of a joint and injury are essential in the recovery and long-term management of the patient (Hockenbury & Sammarco 2001, Wolfe et al 2001). A study by Green et al (2001) found that passive articulation to the ankle joint after an acute ankle sprain greatly improved the recovery of pain-free movement compared to a control group, providing evidence for the importance of passive joint articulation at the acute stage. This was also supported by Collins et al (2004) who found that articulation of the ankle joint caused an increase in joint range of movement, although this study found no significant hypoalgesic effect from the articulation. However, Yeo & Wright (2011) did find a decrease in pain threshold as well as an increase in range of movement with passive articulation.
Structure of synovial joints
Synovial joints are formed from two opposing bones that are connected by a joint capsule which surrounds the joint cavity. The joint capsule is made up of two distinct layers: the first is an outer fibrous coating around the joint; the second is an inner layer of synovial membrane. This second layer is important in the secretion of synovial fluid into the joint (synovial) cavity. The surfaces of the opposing bones are each covered by a layer of articular cartilage, which aids shock absorbency and fluidity of movement in the joint. This synovial cavity, which is found only in synovial joints, permits movement within the joint.
Cartilage
There are three main types of cartilage: hyaline, white fibrocartilage and yellow fibrocartilage.
• Hyaline cartilage forms the fetal skeleton and can also be found in the articular cartilage of synovial joints. In articular cartilage it helps to provide some of the elasticity needed to withstand articular stresses.
• White fibrocartilage has great tensile strength. It is found in the intervertebral discs, the menisci of the knee and the articular discs of the temporomandibular and wrist joints, and it also helps form the labrum of the glenohumeral and hip joints.
• Yellow fibrocartilage is elastic in nature and, unlike hyaline cartilage, does not ossify or calcify. It is found mainly in the external ear and some cartilages of the larynx and epiglottis.
Articular cartilage is the load-bearing material of synovial joints. It is important in the distribution of contact forces and the reduction of friction and wear. It can be compressed by 5–20% of its overall thickness (Eckstein et al 2000, Kersting et al 2005). Articular cartilage is an avascular structure, i.e. lacking blood vessels, lymph channels and nerves. Nutritional supply to the cartilage is predominately from the synovial fluid, so movement through the joint, both passively and actively, is important not only for the optimal health of the articular cartilage but also in its recovery from damage (da Gracca Macoris & Bertone 2001, Viitanen et al 2003, Bertone 2008) and for the smooth lubrication of the articular surfaces. This lubrication and the surface architecture create a smooth and low-friction surface which is five times smoother than ice (O’Meara 1993).
Articular cartilage can be difficult to analyze from a technical bio-movement model due to its composition of a permeable solid medium distended with fluid (Mow & Lai 1980). The major components of the cartilage matrix are collagen (type II), proteoglycans (60–87%) and other minor proteins and lipids (Armstrong & Mow 1982, Poole et al 2001). Histologically, the cartilage is formed of three layers with different directional fibers which deliver a structure that is resistant to load bearing (Mow et al 1989). These are classified into the superficial zone (10– 20% of the cartilage depth), the transitional zone (40–60%) and the deep zone (30%). This layering allows the movement of fluid within the cartilage matrix, making the cartilage a rapid viscoelastic responsive tissue that is able to deform in response to stress without trauma (Mow et al 1980). The hydration of the cartilage causes it to swell moderately and provides extra shock absorbency (Lai et al 1991, Khalsa & Eisenberg 1997, Bursac et al 2000). The change in orientation of the fibers between the layers allows the cartilage to respond to tensile and compressive forces without structural damage, but the layering of the proteoglycans within these tissues is still dictated by the direction of forces through them, even down to the chondrocytes (Guilak 1995, Quinn et al 2001). Therefore it is essential for the nutritional health and potential healing of the cartilage for there to be movement through the joint, whether it is active or passive.
Synovial membrane and fluid
On the inner side of the capsule of the joint is an important membrane called the synovial membrane, which secretes synovial fluid into the joint cavity. An articular joint’s resistance to wear and tear is due in part to the lubrication of the joint with this synovial fluid. One of the purposes of the fluid is to create a layer between the articular cartilages to prevent direct contact. This is particularly effective when the joint is under stress: when the cartilage is compressed and deforms, it expresses fluid to create this layer (Mansour & Mow 1976). Due to its macromolecular contents with polyanionic hyaluronic acid and the fact that it is a dialysate of blood plasma, synovial fluid can be difficult to characterize quantitatively (Mow & Lai 1980, Forster & Fisher 1996).
There have been several theories regarding lubrication of the joints such as fluid film lubrication (Macconaill 1932, Dowson et al 1969) and boundary lubrication (Charnley 1960, Hills 1989, Jay et al 2001, Schmidt et al 2007). In their study, Caligaris and colleagues (2009) suggested that the effectiveness of the synovial fluid is from boundary lubrication rather than from fluid film lubrication and lubrication via fluid cartilage pressurization (Forster & Fisher 1996, Krishnan et al 2004).
The three main theories as to how synovial fluid lubricates joints are:
• interstitial (weeping) lubrication
• fluid film lubrication
• boundary lubrication.
Interstitial (weeping) lubrication
In early studies, McCutchen (1959) pressed a large, flat glass against cartilage and noted what he proposed was interstitial (weeping) lubrication, which caused a decrease in friction and thus allowed ease of movement. This was later supported by his studies on the hypothetical effects of a ‘weeping bearing’ joint in animals (McCutchen 1962). In 2004, Krishnan and colleagues hypothesized that articular cartilage lubrication is dependent on the pressurization of the joint and the ‘cartilage interstitial water’ (Krishnan et al 2004), thus this loading of the joint shifts to a low-friction articular matrix (Forster & Fisher 1996, Ateshian et al 1998, Krishnan et al 2004, Caligaris et al 2009). McCutchen (1962) and Forster & Fisher (1996) termed this ‘self-pressurized hydrostatic lubrication’ and ‘biphasic lubrication’, respectively.
Fluid film lubrication
Fluid film lubrication takes place when opposing articular surfaces are entirely separated by a lubricant film (Hamrock et al 2004). In this theory the layer of synovial fluid over the cartilage is pressurized and disperses mechanical forces across the articular surfaces of the joint. An example of this is motor oil lubricating pistons within a car engine, resulting in low friction while under high stresses. However, this theory has not been proven within synovial joints, possibly due to the porous nature of articular cartilage (Ateshian 2009), and there is currently more evidence to support the boundary lubrication theory (Jahn et al 2016).
Boundary lubrication
Boundary lubrication occurs where there is surface-to-surface contact and it is dependent on the lubricating qualities of the surrounding fluid (Schmidt et al 2007). In a study by Caligaris et al (2009) the authors postulated that, although boundary lubrication would seem more effective than weeping lubrication, both are needed to effectively lubricate the synovial joint. This is confirmed by several studies (Jay et al 1992, Hills & Monds 1998a, Forsey et al 2006, Zappone et al 2007) that show that the boundary lubricants in synovial fluid are more effective than saline lubrication at lubricating articular cartilage. This is evidence for the importance of articulating joints through injury and recovery to ensure effective lubrication of the joint, as stated above and supported by da Gracca Macoris & Bertone (2001), Viitanen et al (2003) and Bertone (2008).
On the other hand, it has been suggested that disuse and degeneration are interconnected (Rapperport et al 1985, Salter 1989, Responte et al 2012). This is due to reduced nutritional input caused by a lack of lubrication during immobility, so movement and articulation of a joint are important for joints, especially if a patient has limited mobility. The potential reduction in interstitial lubrication could explain the increase in friction of a joint that is undergoing osteoarthritic changes (Basalo et al 2004, 2005). In addition, where osteoarthritis affects the production of components of synovial fluid, it can also affect the production of boundary lubricants (Hills & Monds 1998b, Elsaid et al 2007, 2008, Teeple et al 2008, Caligaris et al 2009). This effect again supports the idea that traction and articulation of the joint in a controlled therapeutic manner aid the lubrication of the joint, by what is sometimes called the trans-synovial pump (Fernandez et al 2010). This trans-synovial pump action is made up of three mechanisms that are enhanced with movement:
1. Fluctuation of the intra-articular pressure increases the lubrication of the joint
2. Upsurge in blood flow to the joint
3. Facilitation of drainage of the area via the lymphatic system.
The combined effect of these mechanisms aids in the optimization of synovial elements and their passage in and out of the joint. Therefore, when we undertake articulation of synovial joints as part of manual therapy, we can assume we are encouraging this trans-synovial pump, and potentially the healing within the joint and its surrounding tissues, by aiding the fluid circulation to and from the joint.
Tendons and ligaments
Ligaments (the word ‘ligament’ comes from the Latin word ‘ligare’ which means to bind) and tendons, as well as the joint capsule, surround and encase the joints of the skeletal system. The purpose of the ligaments and joint capsule is to connect and stabilize the bones of the joint and also help in the guiding of movement through the joint (Frank & Shrive 1999). They give the joint stability, and rigidity when needed, and they restrict the range of movement of the joint. Most ligaments are found around the outside of joints and are referred to as extra-articular. Less commonly they are found within the joint and are referred to as intra-articular (e.g. the cruciate ligaments of the knee).
Connective tissues such as tendons and ligaments are formed of approximately parallel collagen fibers which run in the direction of the tensile stress with some elastic fibers mixed in with them. What is interesting is that the ligamentum flavum between the vertebrae has higher elastin content than other ligaments (Nachemson & Evans 1968, Williams et al 1989). Although the fibers are laid parallel, they lie in a wave-like crumpled configuration, without loss of structural integrity. As a joint is put through its range of movement, the connective tissue straightens (see Figure 1.1). Some manual therapists consider this to be ‘taking up the slack’ in the tissues (Maitland 1986, Kaltenborn 1989).
Figure 1.1
The configuration of collagen fibers in a tendon changes from wave-like (left) to straight (right) during joint movement
Following trauma to ligaments and tendons, it is important to encourage movement through them, either passively or actively, along the lines of mechanical stress, in order to aid their recovery (Sawhney & Howard 2002). Physical strain can affect the kinetic chain of protein strands and protein ligands in cells. Applying stress through connective and muscular tissue prolongs the strength and resistance of the tissues (Veigel et al 2003). This structural positioning to mechanical stressors can be found in the majority of tissues in the human body (Schmidt & Wrisberg 2008). Tendons are an excellent example of this structural arrangement of tissue, but it is not only the tendon that responds in this manner: blood vessels, nerve tracts and muscle bundles are all shaped and arranged to withstand and support stress through the tissues and joints, even down to the cellular arrangement of the basement membranes of the cells (Ralphs et al 2002, Huijing & Jaspers 2005).
Evidence would suggest that placing stress on connective tissue is important for its nutritional and vascular health (Noel et al 2000, Schild & Trueb 2002, Heinemeier et al 2007). In addition, movement of the joint has been shown to aid in its lubrication. This, in turn, increases the metabolic actions caused during inflammation and recovery (Hargens & Akeson 1986, Hawley 2010). Some studies have shown that early articulation of a joint after injury can help to develop higher tensile strength of the ligaments, as long as the movement is not too great (Takai et al 1991, Thomopoulos et al 2009).
Joint articulation is often employed to alleviate pain and increase range of motion of the joint by stretching fibrous tissue (Refshauge & Gass 2004). This causes the tissue to change its shape over time as persistent load is applied to it. Prompt articulation of a joint has similarly been shown to reduce the formation of fibrous tissue and adhesions between the tendon and its sheath that could interfere with the normal gliding of the tissues (Thomopoulos et al 2009).
To cause a physical change and lengthen connective tissue, joints need to be articulated at the end range of movement (Threlkeld 1992). When they are placed under stress, tendons and ligaments remodel to the pressures placed through them within their anatomical limits (Herzog & Gal 1999). They strengthen and become stiffer when placed under strain and weaken with less strain (Wang et al 2012). As the body ages, so do the ligaments and tendons, by forming more cross-links in the collagen fibers (Couppe et al 2009). As the tissues age, the collagen content of the fibers decreases, thus decreasing the flexibility of the tissues, decreasing the strength and increasing the diameter of the fibers (Dressler et al 2002, Karamanidis & Arampatzis 2006, Couppe et al 2009). Tendon-related injuries therefore increase as you get older (Dressler et al 2002). When you are articulating a joint, you must reflect on what you are trying to achieve with the individual patient.
Synovial folds (meniscoids)
Intercapsular synovial folds (meniscoids) can be formed either in the embryo or as a result of trauma to the joint. Meniscoids of the spinal facet joints were originally thought to be present mainly in the facet joints of the cervical and lumbar spine, but subsequent studies have shown that they can be found in most intervertebral joints (Kos et al 2001, Webb et al 2009). Farrell et al (2015) discovered that 86% of the cervical facet joints they examined had meniscoids inside them. Meniscoids vary in size and shape (Kos et al 2001), and are not specific to size, gender or joint type (Farrell et al 2015). Meniscoids of the facet joints are of embryological origin, but in other synovial joints they can also be a result of trauma to the joint. The ankle joint is a site where meniscoids regularly occur after trauma to the joint (Lahm et al 1998, Baums et al 2006, Glazebrook et al 2009), and this will be discussed in more detail in Chapter 14, The ankle and foot.
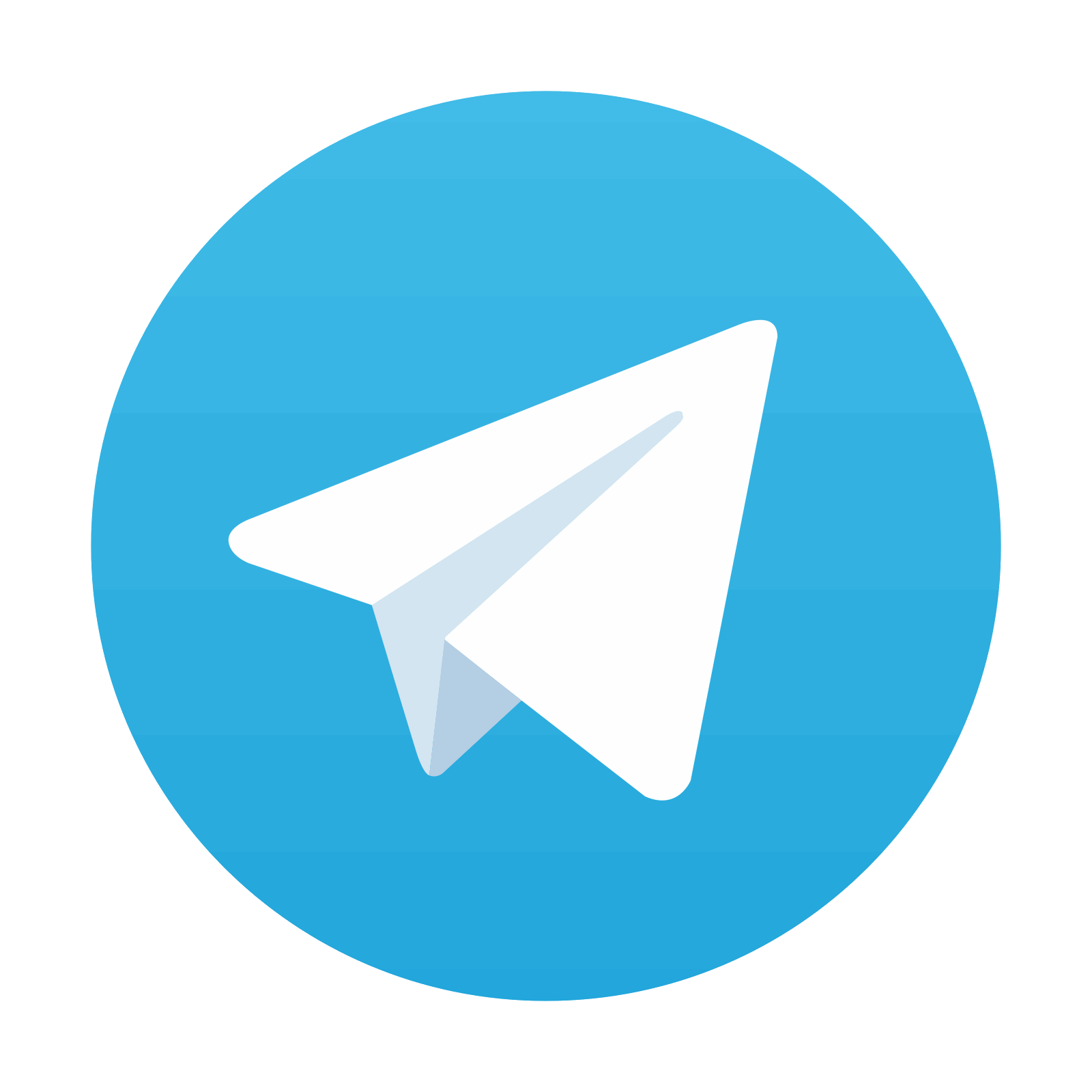
Stay updated, free articles. Join our Telegram channel

Full access? Get Clinical Tree
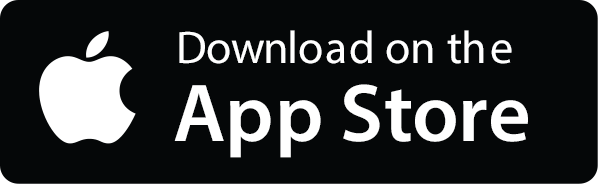
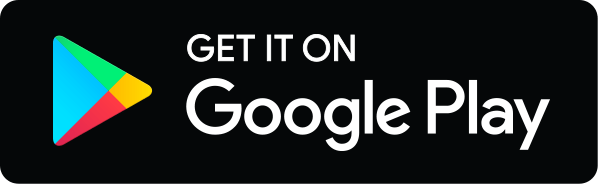