CHAPTER 6 Survey of mechanotransduction disorders
Introduction
Living systems rely on complex biochemical interactions within the framework of a diverse biomechanical environment. Knowledge of the physical basis of health and disease has been underdeveloped even though clinical symptoms may result from altered tissue structure or mechanics. With the exception of the study of ergonomic factors in injury, only recently has attention been given to the regulatory role of external and internal forces on cell, tissue, and system behaviour. How tissues interact and respond to variations in mechanical force is critical in maintaining homeostasis (Yang et al. 1998). Well-recognized examples include the effects of large-scale environmental force modulation of development, as with bone compression, muscle exercise, and vascular pressures. Less clear is the influence of dispersed, microscale forces on all cells. A wide range of disorders have etiology and clinical presentation resulting from abnormal cell and tissue response to mechanical stress (Ingber 2003). Expanding appreciation of these mechanisms poses both a challenge and an opportunity for future care givers.
As evidence-based medicine has come to dominate health policy, the preferred use of manual medicine procedures has shifted primarily to musculoskeletal (MSK) conditions, where the rationale and evidence are clearer. However, observations that non-musculoskeletal conditions may respond to mechanically based treatments continue to be voiced. Based on systematic review (Hawk et al. 2007), evidence from controlled studies, and usual practice potentially supports the clinical encounter in chiropractic care as beneficial to patients with asthma, cervicogenic vertigo, and infantile colic. Specific therapeutic components that may be responsible remain to be clarified. Similarly, there is evidence of potential benefit for an ensemble of osteopathic manual procedures for children with otitis media and elderly patients with pneumonia (Degenhardt & Kuchera 2006; Noll et al 2008). Manipulation of the upper cervical spine has been associated with a reduction of blood pressure in mild to moderately hypertensive patients (Bakris et al. 2007). Treatment of these disorders remains controversial, partly because of the absence of clear theoretical foundations and mechanisms to guide both the selection of appropriate candidates for care and the identification of effective procedures. Are favorable results associated with specific mechanosensitive disorders or for special populations where there is a convergence of circumstances involving genetic and environmental factors? Are treatment effects purely mechanical, reflexive, or the complex interaction of neurohumoral and mechanical factors? How may the results of care be predicted and replicated? If more is understood about mechanotransduction in disease and the treatments offered, appropriate strategies may be developed that bring the right treatment to the right patient at the right time.
Tissue infrastructure for loading
Body segments are constructed in a cross-sectional stratum of overlapping systems, organized in a functional hierarchy. Each system, whether an extremity, the torso or the head, is composed of different organs and tissues (Fig. 6.1). The organ parenchyma is divided by connective tissue partitions. The specialized cells are held together by an extracelluar matrix (ECM) that is continuous across the strata through a network of collagen, glycoprotein, and proteoglycans. Each cell is adherent to the ECM and its neighbors by specialized patches of the cellular membrane termed ‘focal adhesions’ (Katsumi et al. 2004). The membrane, an elastic proteolipid bilayer, deforms instantaneously, bending or stretching in proportion to the application and withdrawal of force. Membrane shape is reinforced by a filamentous cytoskeleton (CSK) that connects to all intracellular organelles and the nucleus (Maniotis et al. 1997). Molecules important to cell function cluster about the focal adhesions and the CSK fibers. The extracellular and intracellular environments are connected through the membrane by specialized integrin proteins. In summary, the stratified structure creates a functional hierarchy where force acting on the tissues results in a coordinated deformation at various size scales that is transmitted through the system. Experts suggest (Suki et al. 2005) that collagen, in its several isoforms, is the key load-bearing component determining cellular health and response to injury.
Biology of loading
The experience of mechanical stimulus surrounds us on a daily basis. Biological systems accommodate to steady-state inputs that allow decision-making processes to focus on signals of change. The adaptation of light touch to the presence of clothing, for example, is a common experience. The flexibility of the extracellular matrix (ECM) in the Pacinian mechanoreceptors rapidly adapts to steady pressure, contributing to a lack of conscious awareness (Ingber 2003). To appreciate this phenomenon, one has only to consider one’s own state of awareness. Are you aware of the touch and weight of your clothing? Only when force changes sufficiently is a signal generated for conscious recognition. Mechanical signals within a healthy range foster function, stimulate growth, metabolic activity, and development. Low physical stress reduces physiologic fitness and increases the risk of heart disease, obesity, and diabetes, and excess exertion risks inflammation, apoptosis (Jaalouk & Lammerding 2009), tissue failure (Chaffin & Anderson 1984) or maladaptive remodelling (Lotz et al. 2004, Tavi et al. 2001). Health is bounded, from above and below, by a range of mechanical forces.
Tissues thrive in a state of mechanical homeostasis (Turner & Pavalko 1998), with cells responding to and interpreting biochemical signals within the context of mechanical forces to provide a structurally rational system. Cells sense mechanical forces which are then translated into modified intracellular biochemistry, structure, and gene expression. For example, it is well known that musculoskeletal tissues respond to exercise and use. What is less well recognized is that mechanical stress contributes to the development and clinical expression of a number of important diseases (Table 6.1) which are among the major sources of health costs internationally (Ingber 2003).
Table 6.1 Diseases that involve mechanotransduction error in their etiology or expression of symptoms
Musculoskeletal | Non-musculoskeletal |
---|---|
Ankylosing spondylitis | Asthma |
Low back pain | Atherosclerosis |
Carpal tunnel syndrome | Diabetes |
Discopathy | Heart failure |
Osteoporosis | Irritable bowel syndrome |
Osteoarthritis | Migraine |
Stroke |
Mechanisms of mechanotransduction
Mechanical regulation distinguishes function across nested structural systems within body regions (see Fig. 6.1). Force is transmitted in cross-section through the layered tissues in a fashion that is defined by the local geometry and tissue and subcellular structural properties to co-regulate behavior. Loads and deformations are dispersed in an organized but non-uniform fashion (Langevin et al. 2005) that results in local concentration and dampening of stress within different strata from the same extrinsic source. Figure 6.2, for example, demonstrates the distribution of tissue strain in the low back strata from a simple passive flexion of the torso.
A relatively large range of forces and moments, whether environmental or therapeutic, act on the human system at any one time. Effective stimuli include indentation, vibration, osmotic pressure gradients, and fluid dynamic pressures (Hamill & Martinac 2001). All cell types are able to respond with differing degrees of potency. Two types of mechanical sensitivity have been identified within the cell membrane bilayer: mechanically gated (MG) channels (Hamill & Martinac 2001, Huang et al. 2003) and transmembrane protein receptors (Jalali et al. 2001, Kamm & Kaazempur-Mofrad 2004). Shear tends to initiate stronger reaction than does compression, with threshold levels that range from 30 to 200 piconewtons (pN).
MG channels sense membrane tension directly, being either ion selectively permeable nonspecific, exchanging fluid volumes (Hamill & Martinac 2001) or passing tension directly to the CSK. Calcium flux across the membrane, for example, is involved in the control of a number of important functions, including growth, differentiation, motility, synaptic transmission, apoptosis, and necrosis. Local membrane stretch opens (stretch activated) or closes (stretch inactivated) channels. Albeit similar to active membrane pump systems in that they transfer ions, MG channels are separate mechanisms that do not generate global electrical responses as seen with neurons.
The transmembrane receptors are composed of integrin proteins (Katsumi et al. 2004) that affect a physical coupling between fibronectin or vitronectin of the ECM and the CSK lattice (actin microtubules and intermediate fibers). Integrin attachments are concentrated at focal adhesions of the membrane, where they provide mechanical stiffening and support to the tissue. The lattice extends deep into the cell, connecting to the nucleus (Maniotis et al. 1997) and other organelles. Shear force transmitted by the ECM tugs on integrin and, through it, is shared with the intracellular structural proteins (Fig. 6.3) and their associated molecular messengers (Burkholder 2007, Ingber 2003, Jalali et al. 2001, Kamm & Kaazempur-Mofrad 2004). The CSK is pre-stressed within the cell as a function of the cell type and the mechanical properties conferred by genetic regulation (Huang & Ingber 2005). The baseline pre-stress, focusing at the intermediate fiber attachments, provides shape control and intracellular tone. The evidence suggests that it is the change in shape of the integrin molecule and the intracellular microtubules that bring key enzymes and proteins together, promoting up- or downregulation of biochemical and nuclear activity. The specific responses to mechanical sensitivity are based on cell type, stimulus type, and the time frame of operation.
There are no available data that explore the relationship between the dosage of exposure (time or amplitude) of tissue layers to extrinsic load and mechanotransduction outcomes. However, the evidence on mechanochemical transduction itself shows effects from ligand triggering that can extend across multiple time periods (Table 6.2). Local mediation appears to require the activation of chemical substrates and enzymes that are already present and available within the cytosol. The interactions occur within seconds to minutes after stimulation. Genetic regulation with protein transcription and translation can begin within minutes to hours of stimulus onset, whereas structural modification is seen within days. These longer-term effects were demonstrated recently when local motion limitation was surgically induced to the rat lumbar spine (Henderson et al. 2007a,b). Lost motion produced local joint adhesions within the articulations and was followed within 8 weeks by osteophytic growth formation off the articular process (Personal communication, Henderson et al. 2007b).
Table 6.2 Timeframe of mechanochemical response from cells triggered by stretch-sensitive channels or ligand transmembrane proteins. Local mediation relies on a cache of enzymes, messengers, and ions available at the membrane. Genetic regulation and structural modifications require the transcription and protein synthesis
![]() | ||
---|---|---|
Local mediation→ | Genetic regulation→ | Structural modification |
Mechanobiology
Load sharing is most studied in the area of the myofascial networks. Various clinical approaches to managing posture and musculoskeletal disorders have been based on the assumption of a kinetic chain or ‘lines of pull’ (Mayers 2009) within the soft tissues, based on anatomical observations, interpreted through tensegrity theory, which states that force applied locally is transmitted throughout the whole structure (Ingber 1997). Clearly, within the cell and based on the cytoskeletal matrix, tensegrity models appear to provide some explanation for pre-stress of the cell structure, making it responsive as a whole to loads that reach it. Within the organ and tissue layers, however, the evidence suggests that the transmission pathways may be more complex. At the very least, the load distributions that occur within the tissues do not appear to be linear (Triano et al. 2009a, 2009b) and are not evenly distributed (Langevin et al. 2005). Loads acting between and within organ structures, at least in the case of muscle, are highly influenced by the geometry of the dense connective tissue attachments. Epimuscular connective tissue separates muscles and muscle compartments, and encases the neurovascular bundles entering them. The percentage of total load transferred by these mechanisms is as high as 50%, depending on joint angle and direction (Yucesoy et al. 2008). Load sharing across and within tissues has four important observations, particularly for the softer myofascial systems (Huijing 2007):
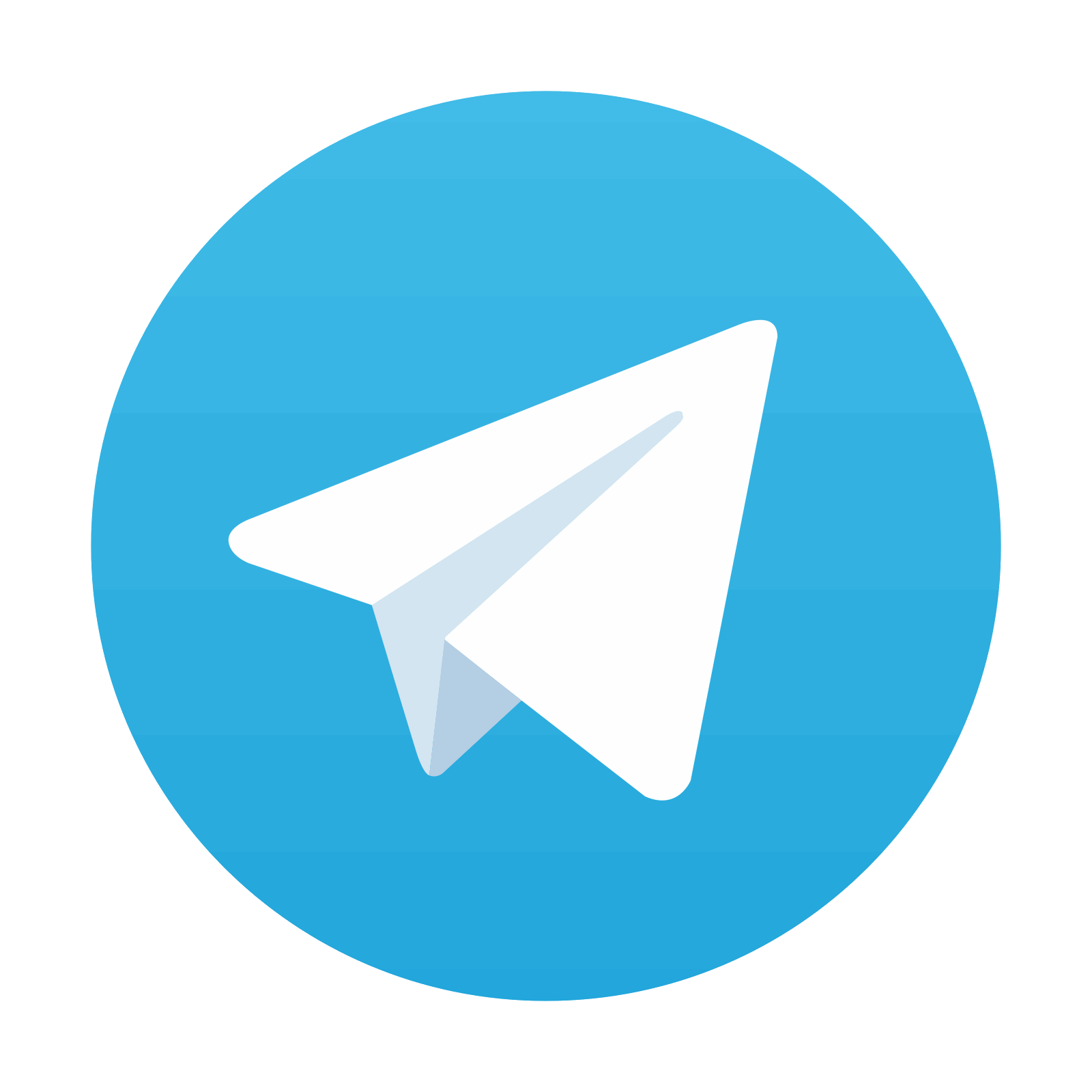
Stay updated, free articles. Join our Telegram channel

Full access? Get Clinical Tree
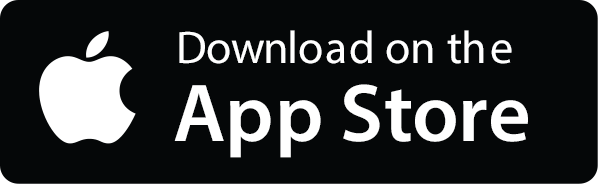
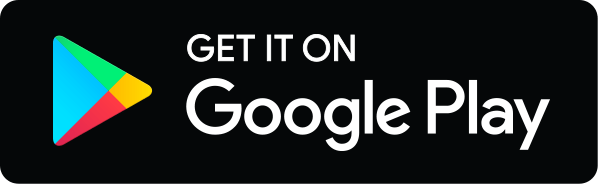