CHAPTER 29
Surgical Management of Spasticity in the Child With Cerebral Palsy
Kat Kolaski, John Frino, and L. Andrew Koman
Surgical interventions for the treatment of spasticity are well-accepted treatment options for children with cerebral palsy (CP). However, recommendations for any potential surgical interventions should be considered in the context of optimal medical and rehabilitative management and rational goal setting provided by a multidisciplinary team of health care providers who specialize in CP. The surgical options currently considered for the child with CP include selective dorsal rhizotomy (SDR), chronic administration of intrathecal baclofen (ITB), and orthopedic surgery. This chapter provides a general review of these surgical options; specific topics covered include scientific rationale, indications, evaluation, planning, techniques, adverse events, and outcomes based on systematic reviews and meta-analyses.
SELECTIVE DORSAL RHIZOTOMY
Rationale
Muscle tone is regulated by the output of the alpha motor neurons in the spinal cord. The alpha motor neurons normally are regulated by interneurons in the spinal cord, which, through a balance of competing excitatory and inhibitory influences, exert a net inhibitory influence (1,2). Inhibitory impulses travel in descending corticospinal projections from the cerebellum and basal ganglia. Excitatory influences from the muscle spindles travel to the spinal cord in the sensory or dorsal roots where they mediate the local spinal reflex arc. In CP, early central nervous system (CNS) injury results in a reorganization of corticospinal projections that reduces the descending inhibition on the alpha motor neurons (3). During a rhizotomy procedure, excitatory input from the dorsal roots is attenuated by sectioning of individual rootlets. Theoretically, this selective sectioning results in restoration of the balance of the excitatory and inhibitory influences on the alpha motor neurons.
Sectioning of the dorsal roots to modify spasticity was described in the early 20th century (4); however, because of concerns that this neuroablative procedure resulted in excessive sensory and motor loss, rhizotomy did not receive considerable attention until the 1970s. Gros et al (5) introduced the concept of selectivity to the procedure with the use of electromyography (EMG) monitoring to identify rootlets innervating more clinically abnormal muscle groups. Fasano et al (6) proposed criteria for rootlet sectioning primarily based on the results of intraoperative electrical stimulation. In North America, this technique was further modified and popularized for the treatment of spasticity in patients with CP by Peacock et al (7–9). Today, most centers performing SDR continue to use variations of the surgical techniques described in these earlier studies.
Indications
The current patient selection guidelines are generally based on descriptions of patients with CP who had the best outcomes in several early studies by Fasano et al (10,11) and Peacock et al (8). The best results were reported in primarily spastic, intelligent, motivated patients who possessed some degree of independent locomotion but who did not have fixed deformity or mass synergy patterns. Peacock et al (8) emphasized the importance of preoperative assessment and postoperative rehabilitation. In these and subsequent series, less favorable outcomes in terms of both spasticity reduction and function were reported in patients with quadriplegic CP (10–12). The reduction of spasticity after SDR—especially in more severely involved patients—has the potential to unmask significant coexisting movement disorders such as dystonia (8,10,12). Greater functional benefits after SDR have been shown in younger patients (13–15) and patients with spastic diplegia versus quadriplegia (16–20). Chiccoine et al (21) reported that a better initial gait score and the diagnosis of diplegia versus quadriplegia were the strongest predictors of ability to walk after SDR. Funk et al (22) found those patients with better motor function (defined as 65%–75% on a standardized scale) benefit most from SDR at 4 to 7 years after the procedure. Based on a multivariate analysis, Kim et al (12) found that the diagnosis of spastic diplegia versus quadriplegia was the only variable that predicted a good versus poor outcome after SDR.
The information accumulated about outcomes of SDR over the past few decades has led to the development of more rigorous criteria for SDR candidacy at most centers currently offering this treatment option for patients with CP (23–27). However, a recent review found such criteria vary between centers and are not based on standardized assessments (28). Screening of potential SDR candidates typically involves a comprehensive clinical evaluation as described elsewhere in this text; videotaping and/or gait analysis are also commonly used (29–31). Good potential candidates are typically ambulatory, intelligent patients 3 to 8 years of age with spastic diplegic CP and a history of prematurity. These children exhibit good proximal strength and selective motor control in the lower extremities with minimal contracture. The ability to participate in and to have access to a physical therapy program post-SDR must also be considered. Relative contraindications include predominantly nonspastic movement disorders, poor trunk control, severe weakness, and multiple, severe contractures or a history of multiple, previous orthopedic surgeries. Parks and Johnston (32) recommend SDR for ambulatory, motivated adults less than 40 years of age who have mild spastic diplegic CP and minimal orthopedic deformity. In general, ITB is recommended instead of SDR for nonambulatory patients with more severe neurologic impairment (19,31,33,34). However, a study comparing nonconcurrent patients treated with SDR or ITB matched by age and functional level found that SDR was more effective in reducing spasticity and improving function but follow-up was limited to 1 year (35).
Techniques
The neurosurgical procedure requires general anesthesia without the use of muscle relaxants. With the patient in the prone position, a midline incision along the lower back is made, and the dura is opened. This was previously done through multiple-level laminectomies or laminotomies from L1 or L2 to L5 or S1. However, because of concerns about the potential impact of such extensive spinal surgery, a single level technique was developed and is currently more popular (27). This involves laminectomy at the L1 level in order to expose the conus, thus allowing exposure of the dorsal and anterior roots (32). The segmental levels of the exposed roots are identified with electromyographic (EMG) recording electrodes placed in 4 to 10 target muscles with L1/L2–S2 innervation (36,37). The L1–S2 dorsal roots are separated from the anterior roots and lower sacral roots and divided into rootlets. The dorsal rootlets are stimulated and their EMG responses are graded. The rootlets within each targeted root segment are selectively transected (Figure 29.1) based on their EMG responses.
FIGURE 29.1 Techniques for performing SDR. (A) The stimulation of the dorsal roots. (B) The transection of the dorsal roots.
Source: Reproduced with permission from Ref. (42). Koman LA, ed. Wake Forest University School of Medicine Orthopaedic Manual. Winston-Salem, NC: Orthopaedic Press; 2001.
The neurophysiologic methods used and the criteria applied to identify which rootlets are “abnormal”—that is, contributing most to spasticity—vary among centers performing SDR. Original criteria for an abnormal EMG response, as defined by Fasano et al (6), include a low threshold to a single stimulus, and a sustained response to tetanic stimulation in the stimulated muscles that may spread to other segmental, contralateral, and/or upper extremity and trunk muscles. Over the past few decades, the validity of these criteria has been questioned. In one series of patients with CP, none of the rootlets stimulated met the criteria for a “normal” response (6,38), whereas another study found that nonspastic patients exhibited sustained contractions that met criteria for an abnormal response (39). In a series of 92 patients with CP, Hays et al (40) found that the percentage of abnormally responding rootlets sectioned at SDR did not correspond to measures of spasticity or function performed at baseline and post-SDR evaluations. The issue is further confused by the variability that exists in the electrophysiologic techniques used among centers performing SDR (9,37,41). In addition, significant intrinsic variability in these responses exists as well (43). Accordingly, a wide range of total rootlets transected are reported in the literature. Peacock et al (8) reported that 25% to 50% of rootlets tested are sectioned in a typical patient with spastic diplegic CP. Other studies published within the past 10 years report that the average rootlet transection rate is between 40% and 70% (25,27,31,34,35), but the maximal percentage imposed varies by center.
There is also specific debate in the literature with regard to the inclusion and sectioning of certain dorsal roots. The L1 root is included when hip flexor spasticity is problematic, but typically one half or less of rootlets are sectioned nonselectively (27,32). Relatively less of the L4 root may be transected to maintain some quadriceps tone (31). Many earlier series excluded the S2 root, presumably because of the potential for injuring functionally normal S2 afferent fibers, which mediate bladder reflexes (8,10,17). However, preserving the S2 dorsal roots allows persistence of potentially abnormal reflex circuits that subserve the ankle plantar flexors; thus, exclusion at this level may result in continued ankle plantar spasticity interfering with function. The literature offers inconclusive evidence, with case series showing spasticity reduction in ankle plantar flexors with (29) and without (44) S2 root sectioning. Lang et al (45) compared retrospective results of SDR in which S2 sectioning was or was not performed. At 6 months post-SDR, they found a significant reduction in ankle plantar flexor spasticity with the addition of the S2 roots but did not evaluate any functional changes. These authors used sensory-evoked potentials to ensure the preservation of bowel and bladder function. Other authors support S2 sectioning with pudendal nerve monitoring and less total percentage of rootlets cut and/or more stringent EMG criteria applied for sectioning (29,31,34,46).
Unfortunately, the ultimate contribution of electrophysiologic monitoring cannot be determined by the available evidence. Only one report compares outcomes after selective versus nonselective rhizotomy, in which a certain percentage of rootlets were transected randomly. This study found no advantage to electrophysiologically guided SDR in 26 patients compared with a historical cohort at 12 months (47). Although most centers currently performing SDR continue to use some form of intraoperative neurophysiologic monitoring for accurate root identification, different approaches to guide rootlet selection have developed at different centers (9,20,31,34,37). These typically involve the critical integration of the unfolding results of neurophysiologic monitoring along with clinical information and goals. Although such approaches are rational and based to some extent on objective criteria, they remain nonstandardized. Finally, because the overall clinical results from centers performing SDR are not significantly different, the value of electrophysiologic techniques remains questionable.
Adverse Events
In general, studies of SDR report a low incidence of adverse events and rare occurrence of any serious adverse events resulting in long-term morbidity (48,49). Intraoperative and perioperative adverse events involving respiratory problems were more common (1.3%–6.9%), as reported in earlier studies (50,51). Improvements in these rates reported in later series are attributed to refinements in surgical and anesthetic care (49,50).
In a review by Steinbok (31), perioperative transient urinary retention is a common adverse event, with an incidence of between 1.25% and 24%. One center reports that transient urinary retention was associated with the use of postoperative epidural morphine analgesia, leading to their recommendation that the epidural catheter be removed at postoperative day 3 with maintenance of the Foley catheter an additional 24 hours (34). Transient dysesthesias are also common (2.5%–40%), but permanent symptoms occur uncommonly (0%–6%; [31]). Persistent sensory changes were associated with more rootlet sectioning in one study (51), but this finding was not statistically significant. The lower rate of post-SDR is also attributed to the decrease in the amount (<70%) of sectioning (25,34).
Late-onset bowel and bladder dysfunction was reported in 5.1% of patients in one series (51), and was associated with the lack of pudendal nerve monitoring (51). As described previously, the current use of pudendal nerve monitoring techniques and more stringent criteria for sectioning are recommended when the S2 root is included. According to several authors, use of these techniques also has greatly reduced the occurrence of bladder dysfunction (34,50,52).
Another important late adverse event is the development of musculoskeletal problems. Development of foot deformities after SDR has been anecdotally reported (53), but not well documented in any retrospective or prospective series. Hip subluxation, another common problem in patients with CP, has been studied more extensively, but many studies are retrospective and do not use consistent definitions of hip pathology. More systematic, prospective studies suggest that the effect of SDR on hip subluxation is likely to be neutral or positive (54–56). One such study found that worsening of hip subluxation after SDR may be more likely to occur in more severely affected patients (56). It has also been suggested that foot deformities and hip subluxation may occur in patients with CP after SDR or other antispasticity interventions more as the result of lever arm dysfunction and growth rather than from spasticity (57,58).
The musculoskeletal area of greatest concern after SDR is the spine. This is because of the association of spinal deformity in patients with CP and risk of spinal deformity associated with multiple lumbar laminectomies (59–61). The risk of spinal deformity after SDR has been evaluated in numerous studies; unfortunately, a lack of consistent surgical and radiographic techniques does not allow for accurate comparison. SDR may increase the incidence of scoliosis, kyphosis, hyperlordosis, and lumbosacral spondylolisthesis (62–70). The increased risk of scoliosis after SDR has been found to be higher for nonambulatory patients with spastic quadriplegia (34,65,66,70), whereas the risk of spondylolisthesis is higher in ambulatory children (63,65,66,70). It has been suggested that the use of a limited laminectomy may reduce the risk of these complications (32,44). However, this finding has not been demonstrated in any controlled studies.
Overall, lower rates of both early operative and late adverse events in the past 20 years are attributed to a variety of technical improvements and refinements, and currently, SDR is considered to be a very safe procedure. More rigorous studies are needed to determine the long-term influence of SDR on spinal and hip deformities; however, based on the available information, development of hip and/or spinal deformities requiring surgical intervention in ambulatory patients who undergo SDR is probably not significantly increased. Ongoing orthopedic monitoring of the spine and hips is recommended for all patients who undergo SDR (33,54,70).
Outcomes
Outcomes of SDR in children with CP have been the subject of two systematic reviews and one meta-analysis. In 2001, the systematic review by Steinbok (71) found good evidence to support SDR efficacy for short- and long-term decreased spasticity (Ashworth score) and increased lower extremity joint range of motion (ROM) for up to 5 years. Good evidence of SDR efficacy for short-term improvement in motor function was also found in the Steinbok review (71) and in a meta-analysis of three randomized trials comparing SDR combined with physical therapy to physical therapy alone (72). This is in contrast to the conclusion of the most recent systematic review published in 2011. This review included only those studies with more than 5-year follow-up and found poor to moderate evidence for long-term positive effects on tone and no evidence of positive effects on function (62). Since this last review was published, several retrospective studies based on prospective cohorts report durable improvements in tone 10 to 20 years after SDR, but their conclusions regarding long-term improvements of function are inconsistent (73–76).
The association between SDR and the requirement for orthopedic surgery is another outcome of interest. However, because of the interdependence of spasticity, deformity evolution, and growth, this is a difficult outcome to evaluate, especially without a control group. In addition, it is important to separate potential effects of SDR on ambulatory versus nonambulatory patients because they differ in terms of risks for type and extent of musculoskeletal deformities. Accordingly, there is weak evidence to support a decreased need for orthopedic surgery after SDR based on a small number of short-term studies (30,71,77–80).
INTRATHECAL BACLOFEN
Rationale
Direct ITB delivery and continuous infusion via a device delivery system evolved to compensate for the problems associated with the orally administered drug. Administration of continuous ITB (CITB) is a nonsurgical, pharmacological treatment that is typically managed by nonsurgical specialists. However, it requires surgical intervention for implantation of the drug delivery system and for correction of later, potential delivery system complications.
Indications
The general indications for CITB in patients with CP and the associated screening process for patient selection are reviewed elsewhere in this book. From a surgical perspective, candidates for the CITB must be medically and neurologically stable and free of infection; in addition, candidates must have adequate body mass and abdominal girth to accommodate the pump (81).
Instrumentation
The Medtronic Synchromed Infusion® system is used to treat spasticity with CITB in children with CP. The system components are a surgically implanted, battery-powered pump connected to a flexible, radiopaque intrathecal silicone catheter. The drug dosage and mode of delivery (eg, bolus or continuous) are adjusted to individual needs with an external programmer using radiofrequency telemetry (Figure 29.2). The pumps have a longevity of 4 to 7 years before needing replacement due to end of battery life. Two pump sizes are available that are approximately the diameter of a hockey puck; however, the pumps differ in the volume of the drug reservoir (20 or 40 mL; Figure 29.3). The 20-mL reservoir pump is thinner and has less volume displacement compared with the 40-mL pump, making it more suitable for smaller and thinner patients (82). Two types of catheters are available, the 8731 model and the newer Ascenda™ models (8780, 8781). Both are two-piece but have different connections between the pump and spinal segments and different anchoring devices (Figure 29.4).
FIGURE 29.2 The 8840 N’Vision programmer uses a touch screen display for data entry, and telemetry for programming drug doses and infusion rates.
Source: Photo courtesy of Medtronic, Inc.
FIGURE 29.3 A lateral view of the two SynchroMed II pumps demonstrating the difference in their width to accommodate either 20 or 40 mL of drug.
Source: Photo courtesy of Medtronic, Inc.
FIGURE 29.4 A SynchroMed II pump showing the catheter access port and catheter attached with sutureless connector. To refill a pump, the central drug reservoir port is accessed percutaneously in the office setting.
Source: Photo courtesy of Medtronic, Inc.
Techniques
Surgical implantation of the ITB delivery system is performed under general anesthesia, typically by a pediatric neurosurgeon. Details of the procedure are described elsewhere (83,84). The procedure involves three basic steps: placement of the catheter in the spine, connection of the catheter to the pump, and implantation of the pump in the abdomen. All steps are performed with the patient in the lateral decubitus position. First, the spinal needle is placed into the intrathecal space at the L2–3 or L3–4 level. The catheter tubing is introduced through the needle and is advanced through the intrathecal space (Figure 29.5). The catheter tip is positioned under fluoroscopic guidance at a spinal cord level predetermined by clinical information. A longitudinal incision is made at the needle site, and the subcutaneous tissues are dissected to secure the catheter with the appropriate anchoring devices.
The next step involves creation of the abdominal pump implant site. The site and depth of pump placement in the abdomen are determined before surgery. The site and side of abdominal placement depend on the presence of feeding tubes, ventriculoperitoneal shunts, and abdominal scars from previous surgery as well as consideration of the patient’s daily positioning and activity level. The pump is placed in either the subcutaneous or subfascial plane. Subfascial placement is recommended in children with CP because it maximizes soft-tissue coverage and decreases the risk of skin breakdown and infection (83,85–88). A transverse skin incision is made, and the subcutaneous or subfascial tissues are dissected to form a pocket for pump implantation (Figure 29.6). The catheter is tunneled subcutaneously from the spinal incision to the abdominal pump implant site where it is connected to a pump that is prefilled with baclofen. The catheter is then secured to the pump with a sutureless connector. In the final step, the pump is anchored using suture loops or a mesh pouch. A postoperative radiograph is used to confirm the continuity and location of the catheter (Figure 29.7).
FIGURE 29.5 During the ITB surgical procedure, the catheter is introduced into the intrathecal space and is advanced to the appropriate spinal level.
Source: Reproduced with permission from Ref. (42). Koman LA, ed. Wake Forest University School of Medicine Orthopaedic Manual. Winston-Salem, NC: Orthopaedic Press; 2001.
Adverse Events
Adverse events may be related to the implantation surgery, infusion system components, human error, or the drug itself. Surgical adverse events are estimated to occur with a frequency of 25% to 40%, with infections having the greatest morbidity (88–90). System-related adverse events usually involve issues with the catheter (eg, kinking, migration, occlusion, disconnection, and fracture). These occur at rates of 15% to 25% and typically require corrective surgeries (89, 91–93). Primary problems with the pump itself occur at rates of less than 10% (91). Both human error and system failures have the potential to cause abnormally high or low drug levels resulting in overdosage or withdrawal, respectively. When either occurs, serious consequences resulting in long-term morbidity can be prevented if symptoms are recognized and treated promptly (83,86,88,89). The acute ITB withdrawal syndrome can occur after sudden cessation of CITB infusion and is potentially fatal (94). However, all cases of pediatric patients with CP who experienced CITB withdrawal described in the literature recovered without long-term morbidity (88). In fact, in studies of children with CP, cases of serious overdosage of ITB are described more frequently than cases of withdrawal (88).
CNS side effects are also reported to occur even at presumably appropriate ITB dosages. These include problems such as nausea, constipation, and headaches, reported at rates of 11% to 36% (88). In addition, specific concerns have been raised about an association between CITB and increased frequency and/or severity of two problems commonly associated with CP—seizures and scoliosis. One retrospective controlled study concluded that seizure activity was not aggravated or induced by CITB (95). Two retrospective studies with control groups matched for a number of clinical characteristics did not find an increased progression of scoliosis in patients with CP treated with CITB (96,97). Special techniques must be used in patients who undergo pump implantation before or after spinal fusion or who undergo the procedures concurrently (98,99). ITB pump placement before spinal fusion is technically less demanding than after spinal fusion (100). One retrospective study that used a matched control group found that patients treated with CITB, who subsequently underwent spinal fusion, experienced higher rates of adverse events but had more preoperative comorbidities (101). Complication rates were found to be similar in a comparison study of patients who underwent ITB implant before, during, and after spinal fusion (98).
In general, CITB is acknowledged to be associated with a relatively high rate of adverse events in children with CP (88). In a multicenter study, Albright et al report that approximately 50% of patients experience some adverse event within 2 months after implantation, and 50% of patients experience an adverse event during chronic therapy (102). In a large series with long-term follow-up at a single center, 25% of patients developed a complication requiring surgical intervention (89). While younger patients and those with more neurologic impairment appear to be at higher risk for adverse events (87,88), one study has shown that treatment with CITB in individuals with CP does not increase mortality (103).
FIGURE 29.6 The pump can be placed (A) under the skin or (B) under the fascia.
Source: Reproduced with permission from Ref. (42). Koman LA, ed. Wake Forest University School of Medicine Orthopaedic Manual. Winston-Salem, NC: Orthopaedic Press; 2001.
FIGURE 29.7 Postsurgical radiographs after pump placement: (A) anterior–posterior and (B) lateral view.
Source: Wake Forest University Press, with permission from Ref. (42). Koman LA, ed. Wake Forest University School of Medicine Orthopaedic Manual. Winston-Salem, NC: Orthopaedic Press; 2001.
Changes in surgical techniques and technologic improvements in the pump and catheter are purported to have decreased rates of adverse events, but to date, such claims have not been substantiated by specific investigations (88). However, there is consensus in the literature that adverse events can be minimized when patients are treated with CITB in a coordinated system of care by a dedicated and experienced team (88,102,104,105).
Outcomes
Since ITB was approved for use in patients with spasticity of cerebral origin in 1996, two systematic reviews considered all published reports of outcomes in patients with CP (106,107). Another review considered only those studies that evaluated the effects of CITB on ambulatory children and adolescents with spasticity of cerebral origin (108). All three of these systematic reviews use an evidence-based approach (109) and classify outcomes using the International Classification of Functioning, Disability and Health (ICF) model (110). Of the total of 57 articles reporting treatment outcomes reviewed in the three reviews, there were three reports providing strong evidence for the efficacy of ITB to reduce spasticity (as measured by the Ashworth Scale [AS] or Modified Ashworth Scale [MAS]) or to decrease neurophysiologic reflexes after a single bolus injection of ITB (111–113). Multiple noncontrolled, prospective or retrospective case series studies report on the effectiveness of chronic ITB for long-term maintenance of decreased muscle tone after pump implantation and for improvements in other aspects of the ICF (eg, decreased need for orthopedic surgery, increased function, ease of care, and health-related quality of life). Although there are a large number of these studies, they lack methodological quality and thus cannot provide high levels of evidence to establish the efficacy of CITB for long-term decreased muscle tone or other improvements. Similarly, a multidisciplinary panel conducted another systematic review of the literature in 2010 and concluded that there were insufficient data to support or refute the use of CITB in children with CP (114).
Since these systematic reviews were published, there appears to be an effort to address the relative dearth of evidence for CITB with the publication of more methodologically rigorous studies (115–118). These have not yet been subject to systematic review; nevertheless, CITB continues to be widely accepted as an effective treatment for improving comfort and ease of caregiving in children with severe spastic and dystonic CP (119).
ORTHOPEDIC SURGERY
Rationale
Orthopedic procedures have traditionally been—and continue to be—the mainstay of surgical intervention for children with spastic CP. Of the five most frequent surgical procedures performed in children with CP in the United States, three are orthopedic (120). However, unlike SDR and CITB, orthopedic surgical procedures do not treat spasticity directly; rather, orthopedic surgery is used to address two major musculoskeletal consequences of spasticity: (a) soft-tissue contractures of muscle, tendon, and/or joint and (b) bony deformities. In the extremities, bony deformities are often referred to as “lever arm dysfunction” and include hip subluxation, torsional deformities of long bones, and foot deformities (121).
Orthopedic surgery for correction of these problems in children with CP must proceed with an understanding of the complex, interdependent processes of deformity evolution, and growth (57,58). Contractures are initiated by the primary problems of increased and/or imbalanced muscle tone resulting in dynamic abnormalities; these abnormal movements and postures can eventually become fixed with muscles in a shortened position. This contributes to the secondary problem of impaired longitudinal muscle growth relative to bone growth (122,123). In the development of bony deformity, the abnormal forces around a joint caused by spastic and imbalanced muscles result in malalignment, which then interferes with normal bone and joint structural development (58,124,125). The processes of both soft-tissue and bony deformity formation are exacerbated in children with impaired mobility due to the lack of normal developmental stimuli from muscle stretch and weight-bearing forces (58,126).
Evaluation
In patients with CP, the musculoskeletal complications of spasticity present with considerable clinical heterogeneity. This heterogeneity reflects the variation that exists in the type and extent of the primary CNS lesion and also depends on the level of skeletal maturity. The orthopedic assessment typically involves both clinical and radiological evaluations. Clinical examination is necessary to document motor function, sensibility, balance, joint ROM, the extent and severity of spasticity, and the presence of other movement disorders. Because day-to-day variation in spasticity and function is observed in children with CP, a potential surgical candidate may need to be examined several times before a final decision for surgical intervention is made (127,128). It is important to observe the patient during activities of daily living and mobility. Details on specific orthopedic physical examination techniques are available in several well-known references (123,129,130).
Radiographic assessment is usually performed with plain radiographs. Serial radiographs are recommended to monitor common problems such as progressive hip subluxation and scoliosis (131,132). Useful radiographic indices commonly used for the measurement of these problems are, respectively, the Reimer migration percentage (Figure 29.8) and the Cobb angle (Figure 29.9). Standing and weight-bearing studies are often helpful. Computed tomography, three-dimensional computed tomography, or magnetic resonance imaging may be indicated to evaluate joint congruency and bony architecture (128,134). The EMG and diagnostic muscle blocks assist in the identification of muscles with clinically relevant spasticity and weakness.
For ambulatory patients, the walking ability of the child is evaluated. This may be performed by observation using a standardized scale such as the Physician’s Rating Scale or—when available—the three-dimensional motion analysis (129,135,136). For children with ambulatory diplegic and hemiplegic CP, instrumented gait analysis has identified common patterns of muscle overactivity and deformity, which are used to guide surgical decision making with regard to the types of the procedures planned and their “dose” (138–141).
FIGURE 29.8 Reimer migration index for measurement of hip subluxation.
Source: Reproduced with permission from Ref. (137). Koman LA, ed. Wake Forest University School of Medicine Orthopaedic Manual. Winston-Salem, NC: Orthopaedic Press; 2009.
FIGURE 29.9 Cobb angle technique for measurement of scoliosis.
Indications and Surgical Planning
Orthopedic surgery is designed to improve function, prevent progressive deformity, relieve or prevent pain, and/or facilitate caregiving. Surgery may impact dynamic deformity and/or prevent the development of fixed deformities. Before orthopedic surgery is considered, spasticity management must be optimized using nonsurgical interventions as described elsewhere in this text and, in selected cases, with the use of SDR or ITB. These interventions may prevent the occurrence of fixed deformities or slow their progression (142–144). When orthopedic surgery is indicated, potential candidates may need referral for a nutritional assessment and evaluation of general medical and neurologic stability (145). Nonsurgical options such as medications, bracing, and adaptive devices may be better long-term strategies for patients with significant medical comorbidities.
Specific issues addressed in preoperative planning include the following: (a) timing of surgery; (b) choice, number, and staging of procedures; and (c) postoperative and rehabilitation care. The decision-making process involved can be complex and is often more challenging than the techniques used to perform the surgery (127,130). Ideally, both the recommendation for orthopedic intervention for a child with CP—and the associated preoperative decision-making process—are informed by the input of a multidisciplinary team (57,146,147).
In general, orthopedic surgery before 4 years of age is not recommended because of the higher risk of deformity recurrence secondary to growth (148–151). In addition, results in younger children may be less predictable because the full spectrum of neurologic impairments and movement disorders may not be apparent, and the child’s gait pattern is not fully mature (31,152,153). If possible, procedures should be delayed until the patient is close to skeletal maturity, but typically, soft-tissue procedures are performed around 5 to 7 years of age and bony procedures after 8 to 10 years (129,154). This schedule allows for more longitudinal bone growth to occur and improves the available bone stock, thus potentially decreasing the chance of recurrence of soft-tissue contractures and improving the stability of bony procedures. However, consideration must also be given to the natural history of specific musculoskeletal deformities and the type and severity of CP. For example, a major exception to this schedule is the treatment of hip subluxation (155). Soft-tissue procedures in young children with early hip subluxation can help maintain or improve ROM and in ambulatory children possibly prevent or delay progress (124,132,156,157). In addition, reconstructive hip surgery in younger, nonambulatory children may be necessary to prevent progressive deformity (158–161).
The performance of multiple procedures to correct soft-tissue and bony deformities in a single session as opposed to intermittent surgical procedures throughout childhood is a general principle guiding the current practice of orthopedic surgery for patients with CP. This approach, which has been termed single-event, multilevel surgery (SEMLS), relies on serial clinical examinations and the anticipation of potential structural skeletal problems (149,162). Because a deformity at one joint can affect the function of others, use of SEMLS potentially decreases the occurrence of complications from interventions performed at a single level (123,163,164). In addition, SEMLS potentially decreases the amount of time needed for hospitalization and rehabilitation. Postoperative management and rehabilitation are vital components and crucial to a successful outcome. Postoperative pain is controlled with epidural, caudal, or local nerve blocks (165). Postoperative spasticity may be addressed with intraoperative chemodenervation with botulinum A toxin intramuscular injection and/or short-term oral medications such as diazepam (127,130,166). Patients with CP often have significant underlying weakness and osteopenia and may be more susceptible to the deleterious effects of immobilization. To prevent loss of muscle strength and cardiovascular conditioning, patients should be given clearance to return to their preoperative level of activity as quickly as possible (167). To avoid complete joint immobilization, removable splints or short-term casting is used. These strategies may also decrease the risk of postoperative pathologic fracture (168). For patients with functional goals, intensive outpatient physical and/or occupational therapy emphasizing strengthening for several months postoperatively is recommended. All postoperative patients and their families should receive instructions for a maintenance home exercise program involving positioning, stretching, splinting, and/or strengthening.
Surgical Techniques
The choice of orthopedic procedures performed to correct musculoskeletal deformities in children with CP is individualized based on patient age, level of neurologic impairment, motor disorder, ambulatory status, and goals. Different types of procedures are used for correction of soft-tissue contracture versus bony deformity, and a variety of orthopedic surgical technique options are available for both.
Motor disabilities in CP are produced directly from hypertonicity (spasticity and dystonia) and weakness. The resultant motor abnormalities interfere with function and, over time, may cause muscle fiber shortening or muscle fiber elongation, joint contracture, and osseous deformity. Spastic muscles are inherently weak but may still overpower antagonists that become elongated. If motor fibers are stretched excessively, sarcomere internal architecture is compromised and further weakened. Conversely, lack of normal muscle excursion produces shortening of external collagen and internal sarcomere architecture with deformity and functional compromise (169). Muscle architecture is also impacted by relative amounts of collagen, premorbid muscle fiber anatomy (eg, unipennate vs bipennate) and fiber type (eg, fast vs slow twitch; [170]).
Soft-Tissue Contracture.
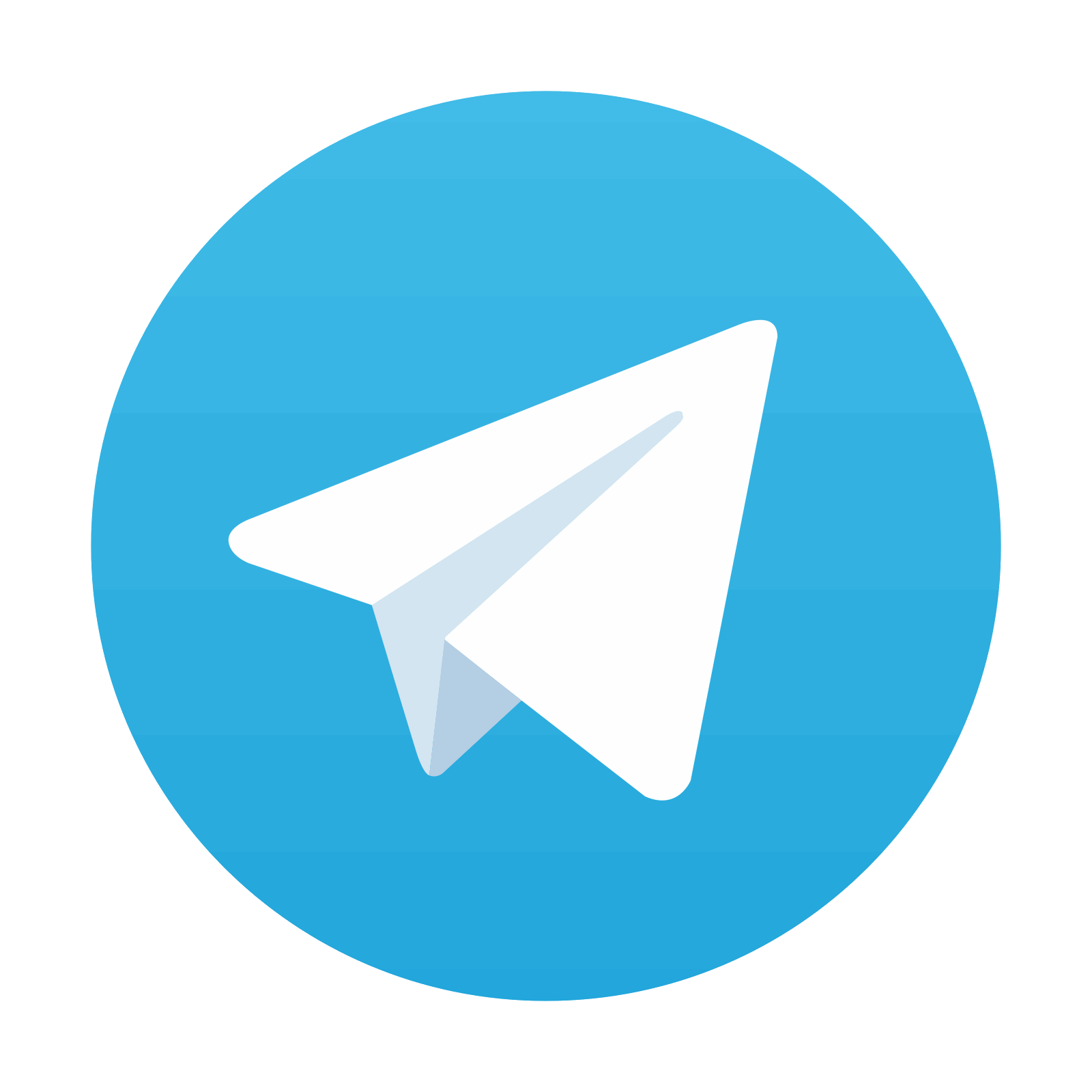
Stay updated, free articles. Join our Telegram channel

Full access? Get Clinical Tree
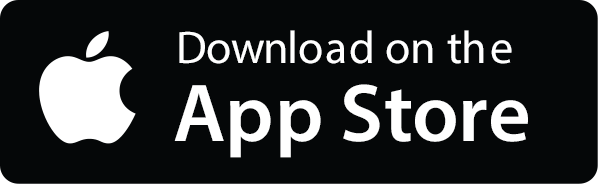
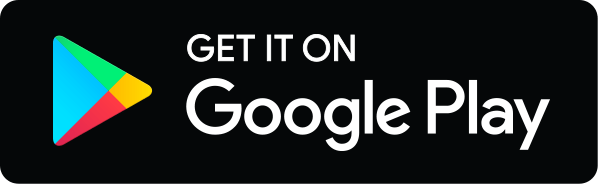