© Springer-Verlag London 2016
Dominique G. Poitout (ed.)Biomechanics and Biomaterials in Orthopedics10.1007/978-1-84882-664-9_1212. Striated Muscles, an Underestimated Natural Biomaterial: Their Essential Contribution to Healing and Reconstruction of Bone Defects
(1)
Department of Orthopaedic Surgery A, Rambam Medical Center, HaAliya HaShniya St 8, Haifa, 3109601, Israel
(2)
Bioengineering Division and Musculoskeletal Disorders Research Laboratory, Department of Orthopedic Surgery, University of Colorado, Denver, Health Sciences Center, 12800 E 19th Ave, RC-1N, Mail Stop 8343, 6511, Denver, CO 80045, USA
Keywords
Striated musclesMuscles, striatedBiomaterials, striated muscleEMG in biomechanicsElectromyography in biomechanicsMuscle fatigue and EMGMechanical and Biological Factors
Surgery of the Musculoskeletal System is the most vibrant, quickly developing and enlarging reconstructive surgical specialty of this Century. The current 10 years are dedicated to this subject, and entitled “The bone and joint decade” which is a tribute to this subject’s significance.
The past 35 years have been the stage for more significant developments and advances in reconstructive surgery of the Musculoskeletal System then all the previous decades from the time the name of “Ortho-Paeis” was cornered during the Industrial Revolution.
Studies into the mechanical properties of the thin wire hybrid three plane, circular external fixator, have opened and enlarged the understanding of biological processes stimulated and supported by the above mentioned mechanical environment. Thus, with time, the crucial role played by striated muscles in the physiology of bone growth and repair is attaining broader recognition and understanding.
Mechanical Properties
Orthopaedic surgeons need to be updated on mechanical terminology.
1.
Compliance is the inverse of stiffness.
2.
Higher compliance allows higher deformation
3.
Stiffness is the ratio between load and deformation. If measured in bending, it is expressed as the ration between the bending moment and the angular displacement is has caused. It is expressed in Newtonmeters by degree (Nm/degree). This modality is well accepted and used by engineers but less so by medical graduates. The latter, prefer the use of compliance.
4.
Stiffness relates inversely to the angle of deformation. In-vivo, bones are surrounded by soft tissues. The latter create ligamentotaxis, which opposes bone displacement, provided bone integrity is preserved.
5.
The ring fixation frame, introduced by Ilizarov into clinical practice, is minimally invasive. It is connected to bone by thin K-wires which create minimal damage to both muscle and bone. These wires, are the only means of osseous fixation for the frame and the varying degrees of tension applied to them, determine their frame’s stiffness.
6.
The ring configuration of the frame, resists torsion, shear and bending.
7.
The factors which determine a ring fixators stiffness are:
(a)
the K-wire tension
(b)
the ring diameter
(c)
the centric or eccentric location of the bone within the ring.
8.
In clinical practice, the ring diameter is often dependent on the circumference of the soft tissues present at the site. The larger the ring diameter, the lower the stiffness. The anatomical tissue topography, further dictates the location of the bone proper in the ring. The more eccentric the bone, the higher the stiffness. Hence, the only control over frame stiffness left to the surgeon, is the control of the tension applied to the transfixing K-wires.
The Biological Result
The mechanical properties of external fixation frames, have a direct influence on the rate of fracture healing [2–27]. In other words, the mechanical properties of external fixation frames have an unequivocal and direct influence on the biological conditions needed for successful (bone gap) fracture healing. This issue has been discussed also by Chao in his presentation to SICOT in Amsterdam [28]. Ring fixation frames harbour a “trampoline” effect which allows both axial compression and distraction during gait.
Intermittent distraction appears to be of crucial importance for physiological bone growth. The proof of this statement are tubercles, tuberosities and bone outgrowths wherever muscles are inserted into bone in the skeleton. Muscle tonus consists of intermittent contractions of muscle fibers. At the site of muscle tendon insertion, these physiological muscle contractions create intermittent distraction. The latter induce local bone growth the end result being a tubercle, a tuberosity or an outgrowth.
Frame stiffness of any ring fixation frame, is one of its more important mechanical properties. This stiffness depends upon the frame’s K-wire tension. For how long does this tension persist in-vivo? Under continuous axial loading, it has been shown that the loading causes a decline in the tension of transverse positioned K-wires [29]. Continuous – in vivo – measurements of the residual tension present in such K-wires in experimental animals, has shown a rapid decline within 2 weeks of mounting the frame, the measured tension values disappearing altogether within 5 weeks of tensioning them in the rings.
Nevertheless, the first signs of intramembranous ossification in the fracture gap, were radiographically detectable 14 days after fracture stabilization by the ring frame, and the whole fracture gap, were radiographically detectable 14 days after fracture stabilization by the ring frame, and the whole fracture gap was filled by woven bone on day 35.
The bridging callus starts, and proliferates from the muscle bed of the fracture. In the tibia, where the significant muscle bed is always postero-medial, this is easy to observe and follow. In both clinical cases and in experimental fractures in the laboratory, the callus has been shown to start always in the posterior plane, next to the muscle bed, and progress from there anteriorly [30–32]. In these publications, the intimate relationship between the developing callus and the muscle bed, has been described.
Most of the load on weight bearing, is transmitted through the fixator [33]. In clinical practice, the choice of the fixator frame means choosing the mechanical configuration which will induce the optimal enhancement in callus formation and fracture healing [33].
A decline in frame stiffness, as initiated by a spontaneous decline in K-wire tension, appears to be an important stimulus for bone formation and healing [7]. Thus, it is very likely that bone mass is regulated by mechanical strain [5].
In fractures, or in the presence of bone gaps, this mechanical strain needs to be induced and maintained in the muscle tissue present in the fracture bed. Micromovements in the axial plane of the fracture stabilized by a ring fixator, induce efficient medullary and periosted callus formation. This callus, undergoes early metaplasia into membranous bone, and thus stabilizes the fracture gap [34]. Similar observations have been reported by Wu [27] and by Younger [35].
The mechanical efficiency of the stabilizing fixation frame, can be evaluated by the amount of fracture callus formation, the stiffness of the latter being capable of early replacing the spontaneously declining stiffness of the ring fixation frame.
In conclusion, therefore, the preservation of the physiological function of striated muscles in the fracture bed appears to be the key biological factor for the efficient repair of a fracture with or without bone loss.
Muscle Assessments
The tools to assess the physiological function of striated muscles are either muscle charting or EMG. Muscle charting is a time honored manual semi-quantitative measurement, practiced by physiotherapists. This is a time consuming, not strictly s scientific method, the human factor being responsible for all variations in it. During the poliomyelitis epidemics, there was a whole generation of highly experienced physical therapists. Who by repeated muscle charting of the same patient, could provide reliable proof to the progress of the disease, or improvement of the patients due to active aggressive physiotherapy.
At the present, reliable, quantitative computerized muscle charting has become available with the aid of ARCON (http://www.arcon-rehab.com/dynamic.asp). This computerized assessment is particularly reliable in measuring the motor power of various muscle groups.
Electrophysiology and Biomechanics
The EMG is an important tool in the assessment of muscle activities in various occupational tasks, only if used properly. The recordings, processing and interpretation of the EMG should be done while considering the proper electrodes size, interelectrodes distance, muscle architecture, contraction rate, motor units recruitment pattern, muscle length, cross-talk from nearby muscles, sampling rate, filter bandwidths, and smoothing time constants. Each of the above factors, if not considered, can introduce substantial error and result in false or misleading interpretation.
The EMG is a by-product of muscle activity and therefore a dependent signal, influenced by various physiological and anatomical factors as well as the technical aspects of the recording/processing protocol.
The anatomical factors that have profound impact on the EMG are muscle fiber penation, and predominant fiber composition (fast or slow twitch types). Physiological factors that strongly impact the EMG are motor units firing rate and recruitment pattern, force generation rate (isometric), and muscle length changes (shortening or lengthening). Secondary anatomical/physiological issues are the size of the muscle, its depth (below the skin and other muscles), the presence of adipose tissue, nearby muscles and proximity to large blood vessels and bones.
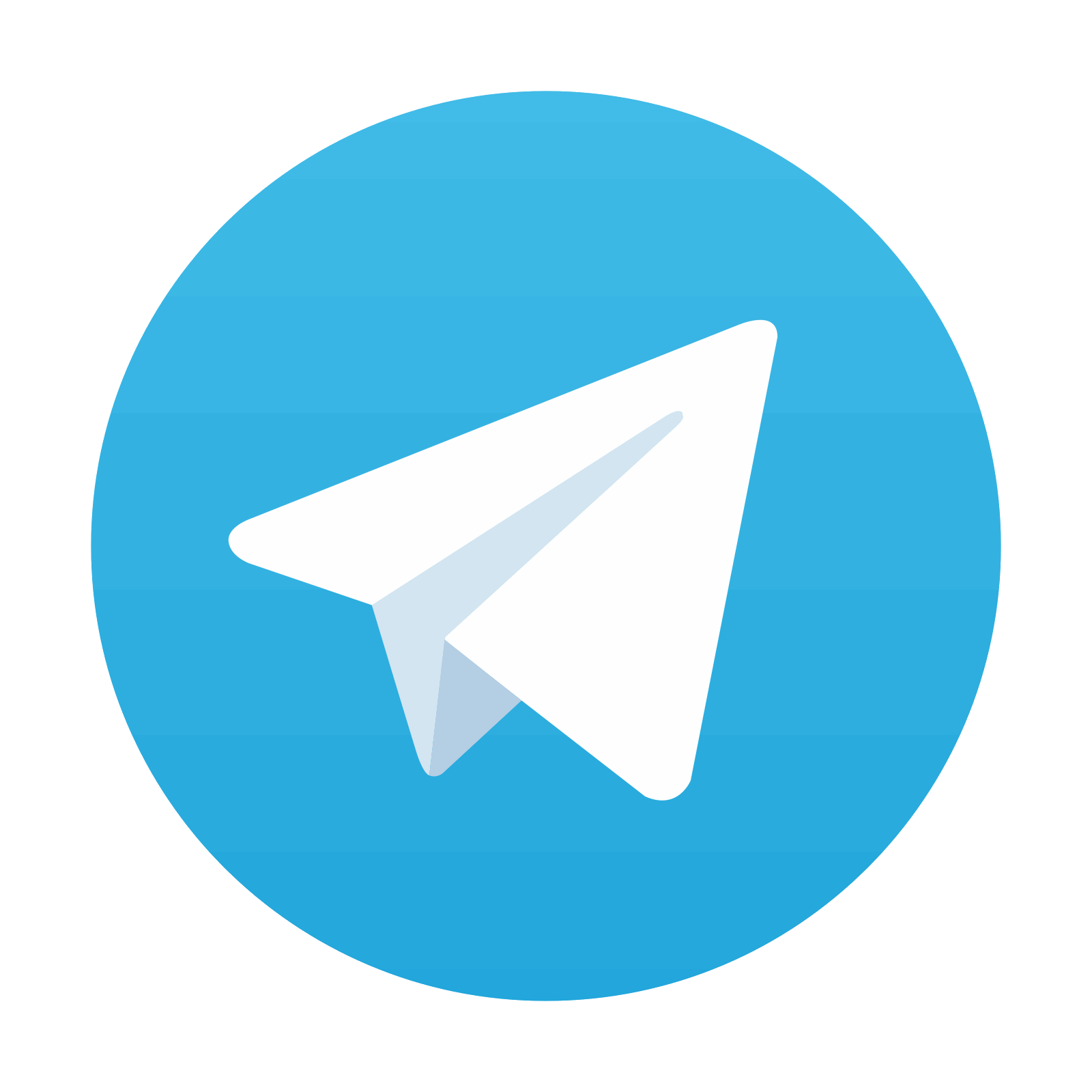
Stay updated, free articles. Join our Telegram channel

Full access? Get Clinical Tree
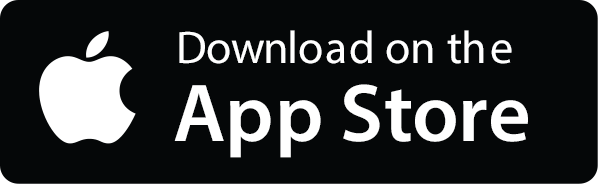
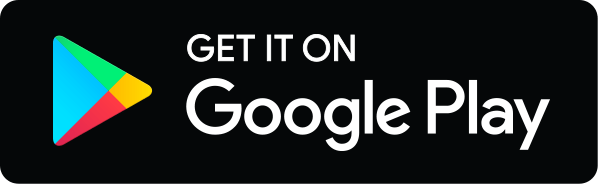