Fig. 11.1
An illustration of the three different zones of the proximal fifth metatarsal [2]. Reprinted with permission from Dameron TB Jr. Fractures of the proximal fifth metatarsal: Selecting the best treatment option. J Am Acad Orthop Surg 1995; 3(2)
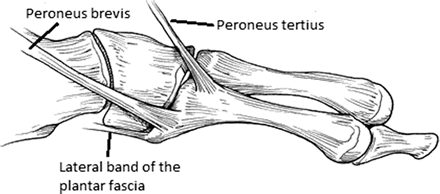
Fig. 11.2
The tendinous and fascial attachments at the proximal fifth metatarsal make this portion of the metatarsal more rigid than the distal portion [25]. Reprinted from Lawrence ST, Botte MJ. Jones’ fractures and related fractures of the proximal fifth metatarsal. Foot Ankle. 1993;14: 358–365
Smith et al. [9] have described the blood supply to the fifth metatarsal. It arises from three sources: the nutrient artery, metaphyseal perforators, and periosteal arteries. The nutrient artery enters medially into the middle third of the bone and then divides into a distal and proximal branch (Fig. 11.3). The proximal branch is shorter than the distal branch and does not reach to the most proximal portion of the metatarsal. An abundance of small metaphyseal vessels feed the metatarsal at each end of the bone.
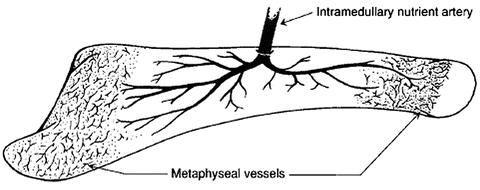
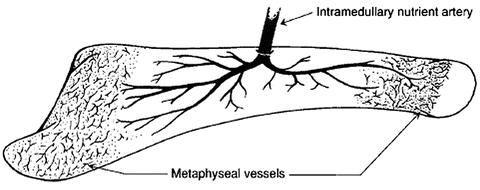
Fig. 11.3
Diagram illustrating the main blood supply to the fifth metatarsal. The watershed area lies at the transition between the proximal branch of the intramedullary nutrient artery and the proximal metaphyseal vessels [2]. Reprinted with permission from Dameron TB Jr. Fractures of the proximal fifth metatarsal: Selecting the best treatment option. J Am Acad Orthop Surg 1995; 3(2)
Pathophysiology
A stress fracture is a result of an accumulation of microdamage from repetitive loading that results in fatigue failure of the bone. The fifth metatarsal is considered a high-risk site stress fracture due to the stresses that it experiences combined with its discontinuous blood supply [8, 10–12].
In regard to blood supply, the area of the fifth metatarsal just distal to the metaphyseal–diaphyseal junction is considered a watershed area. At this location, the metaphyseal perforators and nutrient artery meet but are unable to provide diffuse equal blood flow to the entire area. The bone proximal to this is supplied well by the perforators, and the bone distal to this is supplied well by the nutrient artery. This poor blood supply leads to an impaired reparative biologic response to stresses at the junction [5].
Not only does this area possess a poor blood supply, but it also experiences a high level of stress, especially in athletes. Stress on the fifth metatarsal occurs when the heel is off the ground and body weight is primarily carried through the lateral column. This causes adduction of the fifth metatarsal, which is resisted proximally by ligamentous attachments, effectively resulting in a repetitive varus stress at the metaphyseal–diaphyseal junction [1, 13]. As a consequence, stress typically starts laterally and progresses medially. This results in the worrisome plantar lateral gap due to the tensile forces in this region accompanied by compression at the dorsomedial cortex [8, 14]. Additionally, the metatarsal head is more mobile than the proximal base, which has strong ligamentous and capsular attachments, causing a fulcrum effect to occur at the watershed metaphyseal–diaphyseal junction [15]. Biomechanical studies by Arangio et al. [16] have demonstrated that the principal stress across the bone peaks when the load is directed 30–60° off the horizontal plane. The location of this maximum stress was found to be concentrated in the proximal diaphysis of the bone in the region 3.38–4.05 cm distal to the tuberosity (Fig. 11.4). Further studies in athletes have determined that the greatest pressure differential between the base of the fifth metatarsal and the head occurs during the acceleration phase of running [17]. Based on this study, some prevention programs emphasize longer recovery time between accelerations to prevent build up of stresses in this high-risk location.
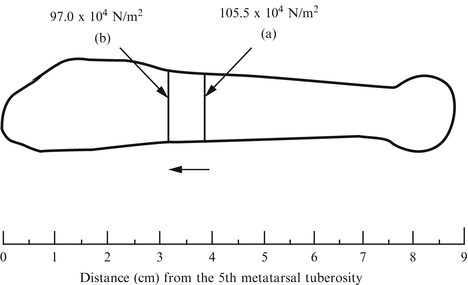
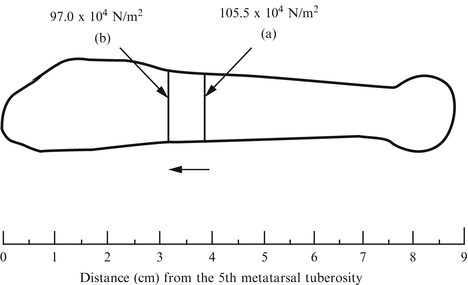
Fig. 11.4
This diagram delineates the location in the proximal fifth metatarsal that withstands the maximum principal stress under a 1 N vertical (a) and horizontal (b) load [16]. Both occur in the area of the metaphyseal–diaphyseal junction. Reprinted from Arangio GA, Xiao D, Salathe EP. Biomechanical study of stress in the fifth metatarsal. Clin Biomech. 1997;12(3):160–164
Classification
A classification system originally described by Torg [4] is commonly used for proximal fifth metatarsal fractures (Fig. 11.5). Torg type I indicates an acute fracture occurring with a chronic process. Radiographs will typically show prior periosteal reaction, a plantar-based fracture lucency, and no medullary sclerosis. Torg type II fractures occur in conjunction with medullary sclerosis and narrowing and are associated with delayed union. Radiographs of a Torg type III fracture reveal obliteration of the medullary canal, and this type frequently represents a nonunion.
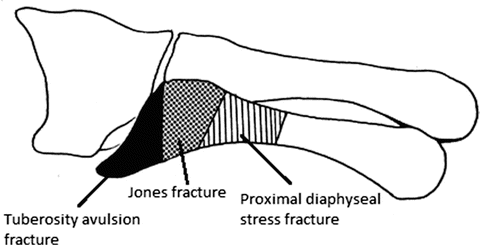
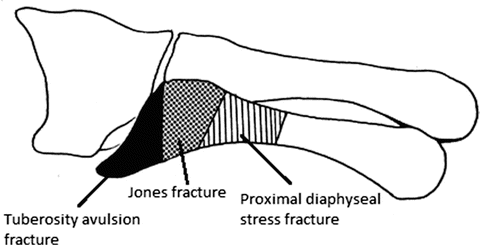
Fig. 11.5
An illustration of the different types of fractures occurring at different areas of the proximal fifth metatarsal [25]. Reprinted from Lawrence ST, Botte MJ. Jones’ fractures and related fractures of the proximal fifth metatarsal. Foot Ankle. 1993;14:358–365
A second classification system was more recently described by Lee et al. [8, 14]. This system is based on the chronicity of the fracture as well as the plantar gap of the fracture, which is measured from the standard oblique radiographic view (Fig. 11.6). Type A1 represents an acute, complete fracture of the proximal fifth metatarsal at the metaphyseal–diaphyseal junction. Type A2 represents an acute on chronic complete fracture at this location. A type B1 fracture is an incomplete fracture with a plantar gap measuring less than 1 mm. Finally, a type B2 fracture is an incomplete fracture with a plantar gap measuring 1 mm or greater.
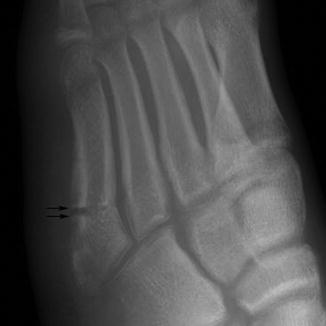
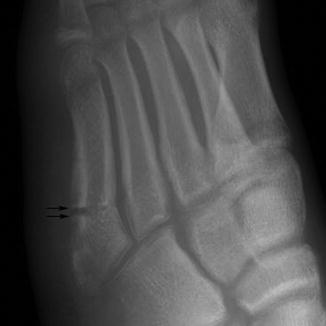
Fig. 11.6
An example of an oblique radiograph of the foot displaying a plantar gap in a fifth metatarsal stress fracture [14]. Reprinted from Lee KT, Young UP, Young KW, Kim JS, Kim JB. The plantar gap: another prognostic factor for fifth metatarsal stress fracture. Am J Sports Med. 2011 Oct;39(10):2206–2211
Diagnosis
Identifying a fifth metatarsal stress fracture begins with a thorough history and physical exam. This should include a review of systems including any hormonal or nutritional irregularities, past medical history, and current medications. Additionally, the clinician should initiate a detailed assessment of training techniques. An injured athlete may present with an acute fracture or an insidious onset of pain with tenderness over the proximal fifth metatarsal. Swelling may be present and inversion of the foot typically exacerbates pain symptoms. An athlete presenting with an acute onset of symptoms may or may not have experienced prodromal symptoms leading up to this acute event [5]. The history and physical exam should focus on identifying both extrinsic and intrinsic risk factors for fifth metatarsal stress fracture [11]. Extrinsic factors include an overly intense training regimen or incorrect footwear and other equipment. Intrinsic factors include a high longitudinal arch, leg-length discrepancy, and excessive forefoot varus.
Imaging
Radiographs
Imaging studies should include an AP, lateral, and oblique radiograph of the affected foot with weightbearing films included if the patient can tolerate. Although early radiographs may be normal, most will be positive for a metatarsal stress fracture at the time of presentation [11]. In the setting of normal radiographs with persistent symptoms, follow-up films will often show callus or a lucent line indicating a stress process. Depending on the clinical scenario, radiographs may show an acute fracture with evidence of a chronic stress reaction at the metaphyseal–diaphyseal junction.
CT Scan
Computed tomography (CT) scan may be necessary for diagnosis or preoperative planning. In the setting of negative radiographs, a fracture may be seen with the use of CT scan. Chronicity may be difficult to determine from plain radiographs; in these cases, CT scan is useful to identify and quantify sclerosis and intramedullary canal obliteration. This can be helpful for preoperative planning because in fractures with canal obliteration, open reduction with bone grafting may be necessary.
MRI and Bone Scintigraphy
Magnetic resonance imaging (MRI) and Technetium-99m-labeled diphosphonate bone scan are most helpful in the case of a compelling clinical scenario with negative radiographs. They are both highly sensitive in diagnosing a stress fracture or stress reaction [10]. An MRI can reveal endosteal marrow edema and periosteal edema of the proximal fifth metatarsal indicating a stress reaction or impending fracture. It can also show a nondisplaced fracture line. Similarly, a bone scan will show increased uptake in the region of stress reaction which will be present prior to complete fracture. Both of these imaging techniques can make an early diagnosis of a stress process in the fifth metatarsal [2, 5, 10].
Treatment
Fifth metatarsal stress fractures have both nonoperative and operative treatment options. Deciding the best option requires careful consideration of the type of fracture as well as the activity level of the patient.
Nonoperative
There has been some success with nonoperative treatment, but the indications are relatively few. Dameron et al. [2] describe successful treatment of these fractures with activity modification and a metatarsal brace if the diagnosis is made early. Torg type I fractures have been successfully treated with non-weightbearing and cast immobilization for 6–8 weeks as long as intramedullary sclerosis is not present [4, 5, 10, 18]. Clapper et al. [19] described a high nonunion rate and prolonged time to complete union when treating proximal fifth metatarsal fractures in 8 weeks of cast immobilization followed by transition to walking boot, but noted 100 % union rate with operative fixation and faster healing time. Chuckpaiwong et al. [20] recommend non-weightbearing cast immobilization for 4–6 weeks followed by a functional splint for another 4–8 weeks to achieve union in a Torg type 1 fracture.
Other nonoperative types of treatment for fifth metatarsal stress fractures have been explored. Research has shown that there may be a place for extracorporeal shock-wave therapy (ECSWT) in treating these fractures in athletes. The exact mechanism of action is unknown, but animal models have suggested that the high-energy acoustic waves induce neovascularization and therefore stimulate healing. Thevendran et al. [15] reported several studies exploring positive results after ECSWT, but they include different types and locations of stress fractures and small subject numbers. Consequently, strong evidence to support the routine use of this modality is lacking [10].
Electromagnetic bone stimulation has also been used in treating proximal fifth metatarsal fractures and has shown some promising results in the setting of stress fractures, delayed union, and nonunion; however, these are largely level IV studies [15]. This modality may be useful as an adjunct to surgery but high-level evidence is still lacking [15].
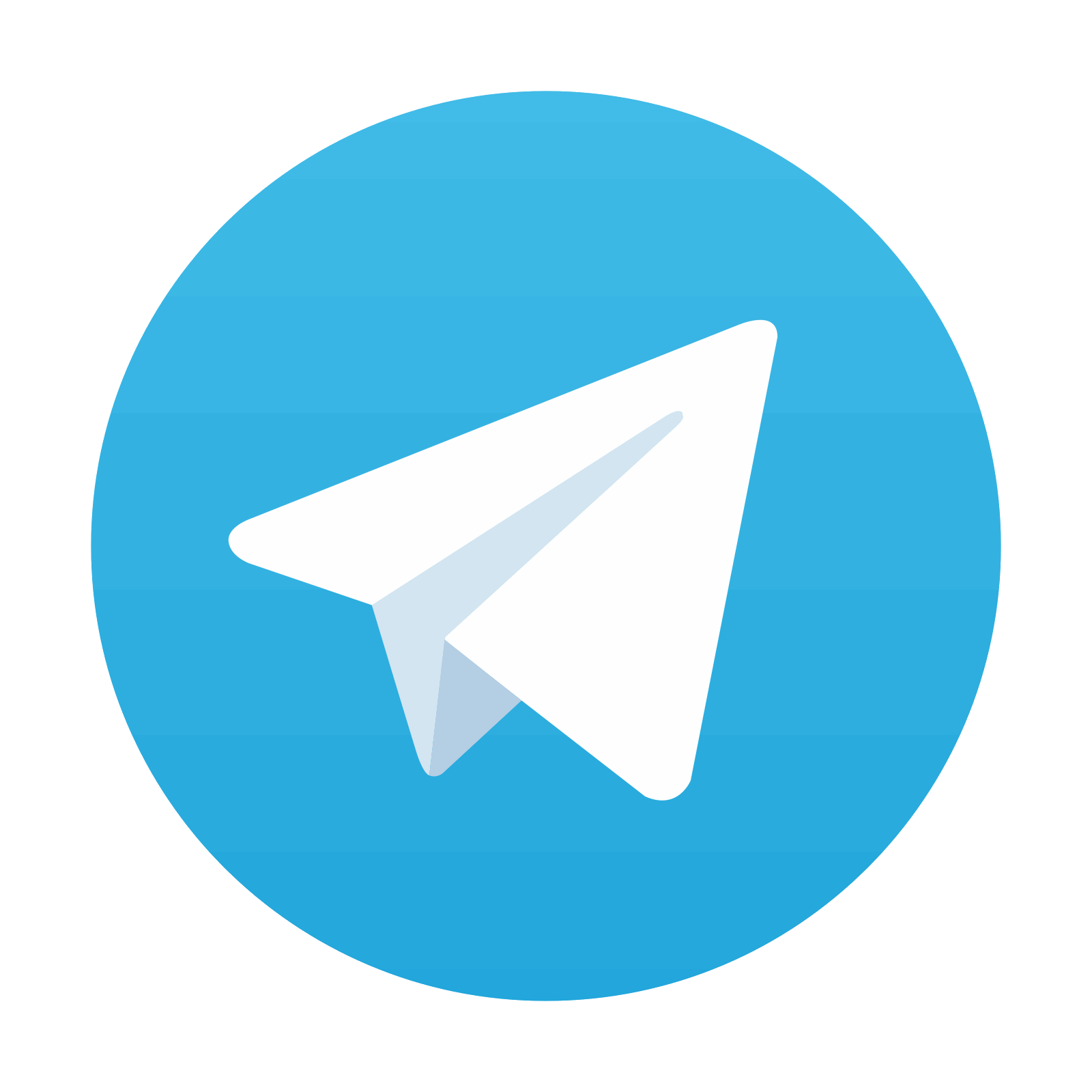
Stay updated, free articles. Join our Telegram channel

Full access? Get Clinical Tree
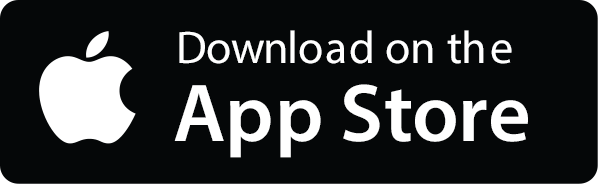
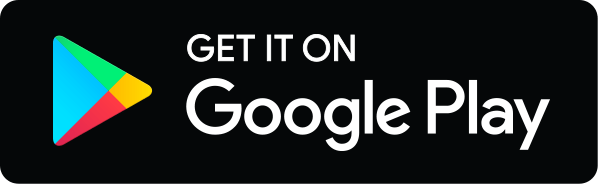