• Training errors
• Sudden increase in exercise intensity and/or frequency
• Poor footwear
• Leg length discrepancy
• Coxa vara
• Pes cavus
• Insufficient energy intake
• Low baseline bone density/mass
• Menstrual dysfunction
• Low dietary calcium intake
• Low lean muscle mass in lower extremity
• Diagnosis of eating disorder
• History of prior stress fracture or reaction
Female athletes are at a higher risk for developing femoral stress fractures than their male counterparts [13]. Irregularity of menstrual cycles and bone mineral density are closely related. Female athletes who experience oligomenorrhea or amenorrhea appear to have an increased risk of femoral stress fractures [14]. Other contributing factors include inadequate calcium and vitamin D intake, low baseline bone density, insufficient energy intake, low lean muscle mass in the lower extremities, and long-term high intensity training [15]. Female athletes who manifest one or all components of the female athlete triad are at particularly high risk for developing femoral stress fractures. The female athlete triad consists of low energy availability (with or without concomitant eating disorder), menstrual disturbances, and altered bone mineral density [16]. Similarly, female athletes who participate in high intensity physical activity before the onset of puberty are at increased risk of primary or secondary amenorrhea, stress fractures, and non-healing fractures [14].
Recently, increased attention has been paid to the risk of developing femoral stress fractures with long-term use of bisphosphonates. Neviaser and colleagues described case reports of low energy atypical subtrochanteric femur fractures with prolonged use (typically greater than 5 years) of alendronate [17]. Several other case reports and series further raised concerns that atypical femur fractures were occurring in patients taking bisphosphonate medications as well as other medications such as glucocorticoids and proton pump inhibitors (Fig. 8.1) [18–25]. However, results from subsequent large registry-based studies and meta-analyses and systematic reviews have been somewhat controversial and inconclusive. Some studies have found no difference in the ratio of typical intertrochanteric to atypical subtrochanteric fractures in untreated and alendronate-treated groups, suggesting that both fractures should be considered osteoporotic in nature [21, 26]. However, a systematic review and meta-analysis by Gedmintas et al. reported an increased risk of atypical femur fractures, subtrochanteric fractures, and femoral shaft fractures in those taking bisphosphonate medications [27]. A report by Shane et al. and the Task Force of the American Society for Bone and Mineral Research indicated that while atypical femur fractures are very rare, there is increasingly compelling data that a causal link between bisphosphonates and atypical femur fractures exists [28].
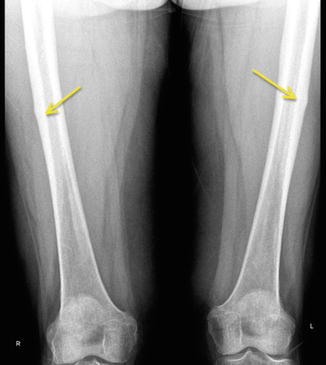
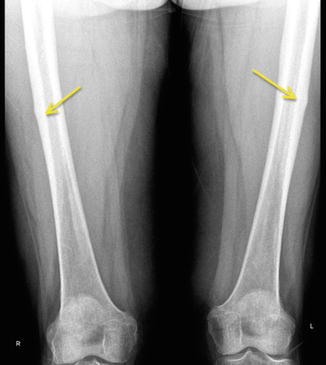
Fig. 8.1
Bilateral tension side femoral shaft fractures in an individual taking prolonged bisphosphonate therapy for osteoporosis
Pathophysiology and Classification
The femur is the largest bone in the body and transmits significant force with any loading activity. In general, bone is strongest in compression and weakest in tension. The femur is typically well suited to handle the compressive forces sustained with physiological activity. Unfortunately, in certain situations bone can fail, resulting in fracture. Running can impose ground reaction forces on the femur 3–8 times that of walking [29]. An insufficiency fracture occurs when the bone quality or structure is not able to withstand normal forces that are placed upon it [3, 30]. In these cases disrupted or abnormal bone remodeling weakens the bony structure and allows injury at otherwise normal physiological strain levels. This can occur when bone is weakened most commonly from osteoporosis but can also be attributed to various medications or other abnormal metabolic processes [22, 23, 27, 31, 32] (Table 8.2). A fatigue fracture occurs when repetitive or excessive strain is placed upon normal bone and the subsequent frequency or intensity of the strain exceeds the bone’s ability to repair itself. Initially microscopic fractures occur and the bony remodeling process, whether from too frequent or too intense loading, cannot keep up with the excessive strain. Instead of the microfractures healing, the process propagates to a macroscopic crack and may eventually lead to a fracture.
Table 8.2
Conditions that predispose to insufficiency fractures
• Osteoporosis |
• Osteomalacia/Ricketts |
• Fibrous dysplasia |
• Paget disease |
• Osteogenesis imperfecta |
• Osteopetrosis |
• Hyperparathyroidism |
• Irradiation |
• Diabetes mellitus |
It is believed that muscle fatigue and conditioning may play a role in the development of stress fractures. The muscles about the femur have broad attachments and have the ability to help transmit and distribute forces [33, 34]. With muscle fatigue or improper conditioning, this ability is lost and forces may be concentrated at certain points along the bone. The geometry of the femur and the distribution of bone mass play a large role in where the femur is loaded and where fractures occur [35]. The femur can be divided into three basic zones: the proximal femur, the femoral shaft, and the distal femur. The proximal femur can be further broken down into three regions: the femoral head, neck, and the peritrochanteric region.
The femoral head experiences the majority of forces on its superior surface where it articulates with the acetabulum. This can be demonstrated anatomically as the trabecular pattern forms a principle compressive group that extends from the medial calcar to the superior aspect of the femoral head. The principle compressive group is a bony strut that helps transmit forces through the femoral head to the shaft (Fig. 8.2). When the bony trabecular structure is weakened or repetitively overloaded, compression fractures typically occur in this location [36].
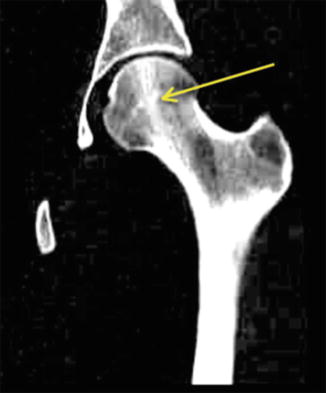
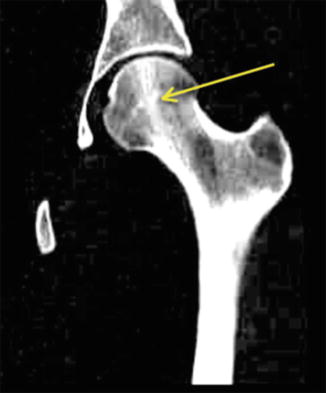
Fig. 8.2
CT scan of femur with arrow demonstrating the principle compressive group of the bony trabeculae within the femoral head and neck
Given that the mechanical axis of the lower extremity falls medial to the majority of the femur, the majority of the medial aspect of the femur is under compression and the lateral aspect is under tension. As a result, repetitive strains to the neck will typically cause compression fractures on the medial aspect of the femoral neck or tension type fractures on the lateral aspect. There have been several classification schemes that have been used to describe and guide treatment of femoral neck stress fractures. Early attempts at classifying femoral neck stress fractures simply classified them into two groups: complete and incomplete fractures [37]. This classification was aimed at guiding treatment, as incomplete fractures were believed to heal with conservative measures. However, complete fractures typically did not have satisfactory outcomes. Devas subsequently attempted to radiographically classify femoral neck stress fractures based upon their biomechanics [38]. Femoral neck stress fractures were classified into compression type fractures and transverse fractures. Compression fractures were seen on the medial or inferior femoral neck where callous or sclerosis was noted (Fig. 8.3). These fractures were felt to be stable injuries that would typically heal with conservative measures alone. Transverse fractures, which were later renamed “distraction” type fractures, typically began as a crack on the tension side, or the superior surface, of the femoral neck. These fractures have a high risk of propagating from the lateral side and may eventually become displaced. The first study to prospectively evaluate the diagnosis and treatment of femoral neck stress fractures was done by Fullerton [39]. Using radiographs and radionuclide studies he followed 54 femoral neck stress fractures. As a result, he modified the classification scheme for femoral neck stress fractures to three major groups: compression side, tension side, and displaced. He found that compression-sided injuries may be radiographically negative and only show increased signal on the compression side of the femoral neck on radionuclide scan, or as noted by Devas, it could show callous or sclerosis in this region. In tension-sided stress fractures, radiographs may also be negative, have significant callous formation, or even an overt fracture line on the superior surface of the femoral neck. With the advent of magnetic resonance imaging (MRI) and its increased use in detecting stress fractures in general, Shin et al. at the Naval Medical Center in San Diego further modified the classification scheme to include the use of both MR and plain radiographic features (Table 8.3) [40] (Fig. 8.4). Their classification further subdivided compression type fractures into those with a fatigue line that was less than 50 % of the femoral neck (Fig. 8.5) and those with more than 50 % (Fig. 8.6) of the femoral neck. Tension-sided fractures typically, along with the previously noted radiographic findings, may demonstrate increased signal on T2 or STIR along the superior aspect of the femoral neck.
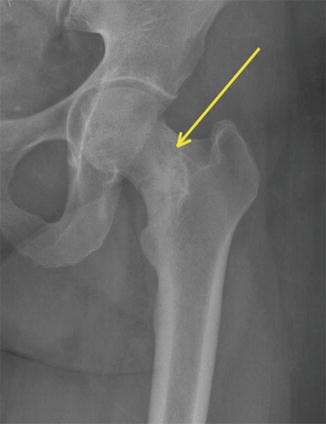
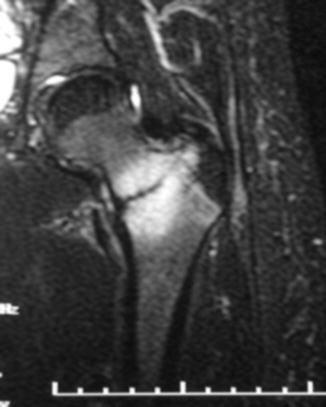
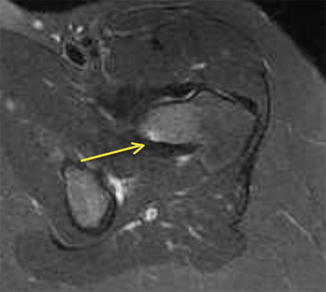
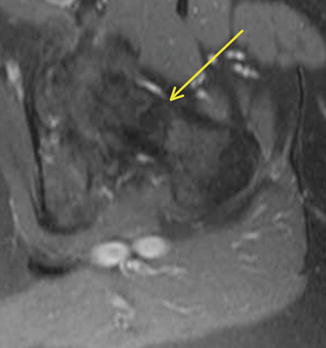
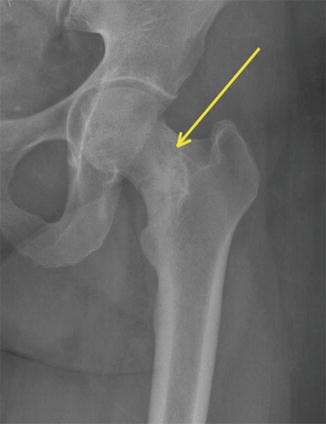
Fig. 8.3
Plain radiograph of femur demonstrating compression side stress fracture of the femoral neck (arrow)
Table 8.3
Naval Medical Center femoral neck stress fracture classification
Type | MRI/radiograph feature | Treatment |
Compression | No visible fracture | Nonsurgical |
<50 % | Nonsurgical | |
>50 % | Surgical | |
Tension | Any | Individually based decision |
Displaced | Any | Emergent surgical fixation |
Atypical tensile | Any | Nonsurgical |
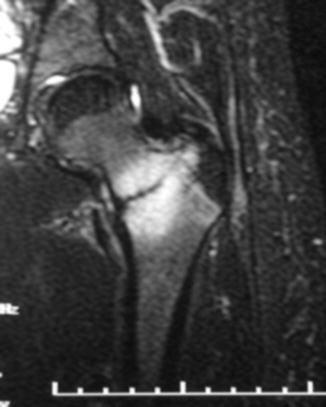
Fig. 8.4
Coronal T2 MRI demonstrating a nearly complete femoral neck stress fracture
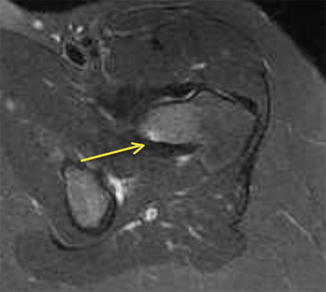
Fig. 8.5
Axial STIR MRI of the proximal femur demonstrating a stress fracture of femoral neck less than 50 % of the total width of the femoral neck (arrow)
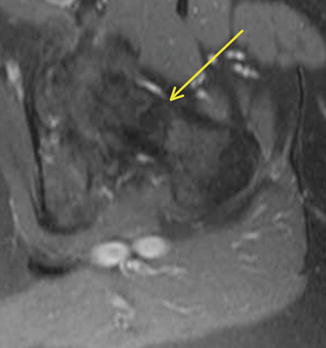
Fig. 8.6
Axial STIR MRI of the proximal femur demonstrating a femoral neck fracture greater than 50 % of the total width (arrow)
Stress fractures in the subtrochanteric region and shaft are relatively rare. They typically occur in athletes or military recruits, but they may also occur in individuals with osteoporosis and have been correlated with prolonged bisphosphonate use as previously noted (Fig. 8.7). Femoral shaft fractures are typically classified anatomically based upon their location in the femur. They may be divided into proximal third, middle third, and distal third, as well as based on whether they occur on the medial or lateral cortex. The majority of femoral shaft stress fractures, especially in runners, appear to occur in the posteromedial aspect of the femur at the junction of the middle and proximal third of the femoral shaft [6, 41–43] (Fig. 8.8). This is the area of the femur that experiences the greatest compressive forces [44]. Stress fractures in the distal shaft are more commonly seen in military recruits [45]. Distal third of the femur may be further classified into supracondylar, condylar, or subchondral insufficiency type stress fractures, and all of these are quite rare [9, 46].
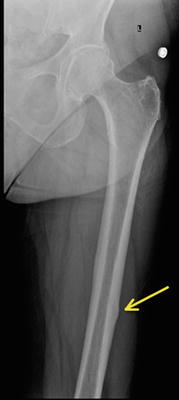
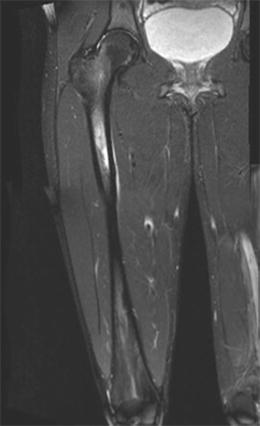
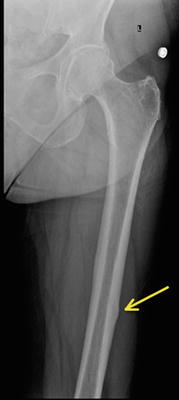
Fig. 8.7
An atypical femur fracture with cortical beaking (arrow) on the tension side of the femoral shaft
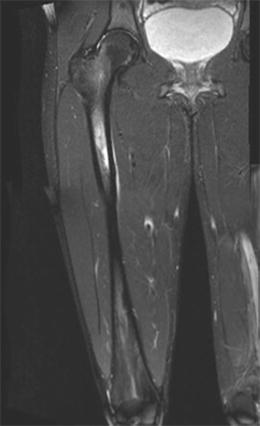
Fig. 8.8
Coronal T2 MRI of the femur demonstrating the common location of femoral shaft stress fractures at the posteromedial junction of the proximal and middle third of the femur
Diagnosis
The diagnosis of femoral stress fractures includes a thorough history, comprehensive physical examination, and imaging studies. Laboratory studies, although not typically necessary for diagnosis of stress fracture, may help to explain the etiology of an insufficiency or pathologic fracture [47]. Prompt diagnosis of femoral stress fractures requires a high index of suspicion, as many stress fractures can present with vague, nonspecific symptoms. Up to 75 % of femoral stress fractures can be missed or misdiagnosed on initial evaluation [48]. Femoral stress fractures can be mistaken for muscle strains, synovitis, bursitis, infections, or neoplasms [49]. The differential diagnosis for femoral stress fractures can also include slipped capital femoral epiphysis (SCFE), Legg–Calve–Perthes syndrome, or hip dysplasia [10, 49] (Table 8.4). Obtaining a thorough history is key. It is important to gather information regarding the athlete’s exercise regimen, dietary intake, history of prior stress fractures, past medical history, and menstrual cycle. Typically athletes with femoral stress fractures will report an insidious onset of gradually worsening leg, thigh, or hip pain that may worsen with activity. Athletes with femoral neck stress fractures may report symptoms as early as 2 weeks after increasing exercise intensity, and typically report pain in the groin [39]. With femoral shaft fractures, athletes often present with activity-related pain in the thigh or ipsilateral knee [45]. Pain may limit athletes from participation in athletic activities, and sometimes may be associated with night pain.
Table 8.4
Differential diagnosis of femoral stress fractures
Proximal femur | Femoral shaft | Distal femur |
---|---|---|
• Bursitis | • Muscle strain | • Femoral condyle avascular necrosis |
• Muscle or bone contusion | ||
• Tendinitis | ||
• Compartment syndrome | ||
• Infection | ||
• Muscle strain | ||
• Knee arthritis (degenerative or inflammatory) | ||
• Infection | ||
• Neoplasm | ||
• Knee cartilaginous or ligamentous injury | ||
• Avascular necrosis | ||
• Patellofemoral pain syndrome | ||
• Muscle strain/injury | ||
• Plica syndrome | ||
• Neoplasm | ||
• Infection | ||
• Neoplasm | ||
• Snapping hip syndrome | ||
• Slipped capital femoral epiphysis (SCFE) | ||
• Osteitis pubis | ||
• Piriformis syndrome | ||
• Sacroiliac injury | ||
• Acetabular fracture | ||
• Pelvic fracture | ||
• Legg–Calve–Perthes syndrome | ||
• Hip impingement | ||
• Arthritis (degenerative or inflammatory) |
The general location of the athlete’s pain may guide physical examination, although the clinical presentation can be variable. A comprehensive physical examination, including the affected limb, contralateral leg, hip, and knee, as well as the pelvis and lumbosacral spine, is required. Athletes with femoral stress fractures may ambulate with an antalgic gait. Typically muscle tone and bulk are normal, and swelling is absent. Overlying skin changes such as erythema and ecchymosis should not be seen. Given the deep nature of the femur, the point of maximal tenderness may be difficult to elicit. Femoral shaft stress fractures may exhibit no tenderness to palpation at all, while more superficial subcondylar fractures may be tender to touch. Deep palpation of the groin may elicit pain in femoral neck stress fractures [39]. Athletes with femoral neck stress fractures may experience pain with logrolling, passive extremes of motion, and straight leg raise. Tuning fork testing, heel striking, and percussion along the femur do not reliably correlate with femoral stress fractures [5]. Athletes with distal supracondylar stress fractures or femoral condyle fractures may exhibit joint line tenderness of the knee and pain with knee range of motion.
Hop testing, where the athlete hops on the affected leg and is asked to localize his or her pain, can be an effective physical examination strategy. An estimated 70 % of athletes with a positive hop test are found to have femoral stress fractures [50]. Fulcrum testing of the femoral shaft can be a useful physical exam tool as well. In fulcrum testing, the athlete sits at the edge of the exam table, with the examiner’s hands placing gentle downward pressure on the knee and anterior pressure on the posterior proximal femur [6]. A positive test will induce pain along the femoral shaft (Fig. 8.9).
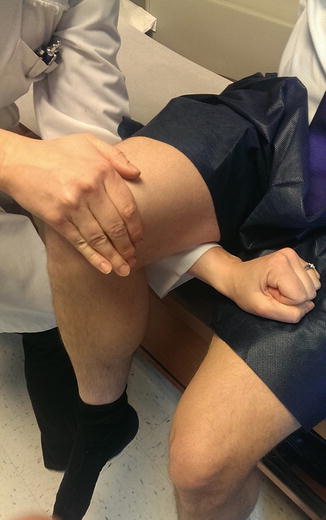
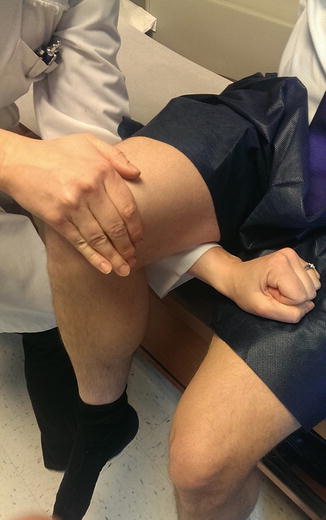
Fig. 8.9
The fulcrum test is used to help detect the presence of femoral shaft stress fracture. The examiner’s arm is placed under the proximal thigh of the affected leg and then the other hand applies a downward force to the distal femur. The test is positive if pain is elicited
Imaging
Imaging studies are readily used in the diagnosis of femoral stress fractures. It is the changing structure of bone in response to stress loading that allows imaging studies to aid as diagnostic tools [51]. The most commonly used imaging modalities include plain radiographs, MRI, and nuclear scintigraphy. Conventional radiographs are typically the first imaging studies obtained in the evaluation of suspected femoral stress fractures. Radiographic evidence of stress fracture may be subtle, and in the early stages may include a faint radiolucency in the cortical bone of the femur [31]. With resultant bone modeling, the endosteum may become irregular, thickened, and show signs of sclerotic change (Fig. 8.10). Periosteal reaction involving both the cortex and endosteum follows as the fracture continues to heal. Generally, new periosteal bone formation can be seen about 10 days after the injury process begins, and peak formation occurs at 6 weeks [31, 52]. Often radiographs may appear negative in early stages of the disease process. A mere 10 % of femoral stress fractures show discrete radiographic evidence within the first week [40]. Obtaining serial radiographs at week 2 or 3 still may not manifest characteristic evidence of fracture healing or new bony callus formation [53]. Therefore, any athlete with persistent femoral pain greater than 2 weeks should be considered highly suspicious for stress fracture despite negative conventional radiographs. In these cases, more advanced imaging techniques are often necessary to confirm the diagnosis.