Cause of index attack
Males (%)
Females (%)
Cause not evident
79.3
88.9
Strain
10.9
4.3
Proved diska
7.6
5.6
Ankylosing spondylitis and neoplasms
0.5
0.6
Direct trauma
0.5
–
Others
1.1
0.6
The population reported on by Dillane et al. [19] is not the population routinely seen by spine surgeons; however, the notion of nonspecific low back pain has persisted and remains a common retort for those against spine interventions for both chronic low back pain patient and patient with persistent low back or leg pain patient after surgery. Many physicians persist in offering only pharmacologic management, cognitive behavioral therapy, functional restoration, and/or physical therapy under the belief that the spinal pain is primarily psychogenic in origin or that the pain has become centralized, and therefore the search for a pain generator is futile and unsupported by evidence. Interesting, as briefly noted above, an important historic study by Rowe [31] was published in 1969 shortly after the Dillane study, but has been ignored from an epidemiologic perspective. Rowe (1969) studied 500 employees with chronic low back pain for 7 years at the Kodak factory [31]. Rowe states the reasons for his 7-year observational study best: “Studies based upon short-term observation of patients with low back disability have yielded information nearly as tunnel-sighted as that gleaned from the single etiology approach. Experience indicates that backache is characteristically intermittent, episodic, and recurrent and can meaningfully be studied only as a continuum which stretches through the active years in a man’s life.” Rowe [31] reported a diagnosis in approximately 80 % of his patients, including disk pain (54 % both intrinsic discogenic pain and pain due to disk herniation), inflammatory backache (14 %), and miscellaneous causes (10 %). Rowe did not have use of CT scans, MRI, or diagnostic spine injections to identify facet or sacroiliac joint pain. The use of provocative discography is not reported. Furthermore, in the late 1960s, discography had fallen out of favor based on the negative discography study by Holt [32]. Holt’s study was later discredited as he utilized a nonstandard technique on prisoners receiving incentives for study participation.
In summary, the statements on low back pain being almost entirely “nonspecific” and “idiopathic” are based on a single, general practice study on acute low back pain (less than 2 weeks) in the late 1960s [19]. The terms “idiopathic low back pain” and “nonspecific low back pain” then reentered the literature with the 1982 Symposium on Idiopathic Low Back Pain publication by eminent spine surgeons Augustus White and Alf Nachemson [20]. Research published at the same time as the Dillane study was ignored [31]. While Dillane et al. [19] accurately reported his experience as a general practice doctor (in 1950s Boston, MA USA) caring for patients with less than 2 weeks of low back pain, his results should not be applied beyond that time or narrow clinical setting. However, the statistic continues to be taught in medical school and utilized by many authors, payors, and policymakers to apply to all causes of low back pain and most importantly to patients with chronic low back pain. The statistic is an example of a “literature legend” wherein the original study, clinical setting, and relevance have been lost over time. “Nonspecific low back pain” and “idiopathic low back pain” are terms used primarily by detractors of spine surgery and spine injections. Just as the majority of etiologies of failed back surgery syndrome have been elucidated, so have the etiologies of “nonspecific low back pain.” The terms “idiopathic” and “nonspecific” low back pain are not supported by the current published evidence.
38.3 History of “Failed Back Surgery Syndrome (FBSS)”
It is useful to examine the history of failed back surgery syndrome (FBSS) or persistent pain after lumbar surgery (post-lumbar surgery syndrome) before looking to the present state-of-the-art in diagnosis and treatment of the condition. Patients with persistent pain after lumbar spine surgery represent a heterogeneous group which has changed over the years due to advances in surgical techniques and diagnostic/therapeutic spinal injections. Until the early 1980s, largely single-surgeon or single-institution case series results were reported, but no evidence-based, critical quantitative studies. A brief historical review demonstrates the significant advances achieved in both diagnosis and treatment of low back and leg pain before and after lumbar surgery. Reviews of spine surgery outcomes published in the 1980s [5, 33] lacked MRIs and diagnostic/therapeutic spine injections and were unable to diagnose a significant percentage of PLSS patients. The authors attributed PLSS in many patients to psychosocial comorbidities and secondary gain as primary pain drivers. Long et al. [33] were unable to find a cause for the patients’ pain in up to 44 % of patients: 21 % of patients were classified as “normal,” and 21 % were diagnosed as having expected postoperative changes/mild spondylosis – not consistent with their persistent pain. A majority of patients were instead diagnosed with primary psychiatric and addiction problems as well as prominent motivations for secondary gain. Pain medicine specialists, also lacking diagnostic tools at that time, diagnosed a majority of patients with persistent pain after lumbar surgery as having a “centralized pain syndrome,” as opposed to ongoing, correctable peripheral nociceptive lesions. Patients with centralized pain syndromes and “learned chronic pain behavior” were relegated to functional restoration, medication management, and cognitive behavioral therapy interventions.
Seven seminal quantitative studies are reviewed here (see Table 38.2). In 1981, Burton et al. [5] published the first quantitative study on the etiologies of “failure of surgery in the lumbar spine.” Collectively, this paper reflects the experience of surgeons who treated an average of “800 FBSS cases per year.” The authors summarize the status of patients with persistent pain after lumbar surgery, circa 1970s: “The FBSS entity represents a highly complex challenge to the physician. Patients with this condition can rarely be “cured” because FBSS is actually a spectrum of organic disease processes complicated by secondary financial gain and learned chronic pain behavior. While many of these patients can be “salvaged” to varying degrees by comprehensive rehabilitation programs, it is uncommon to achieve complete pain relief by any combination of therapeutic measures. This is due in part to the great difficulty in quantitating pain and associated psychologic, occupational, social, monetary, intellectual, motivational and educational factors” [5]. During the 1970s, diagnostic imaging was limited to x-ray, CT scan, CT myelography, and selective use of discography. MRI (particularly with and without gadolinium postoperatively) has contributed significantly to understanding the etiologies of PLSS.
Table 38.2
Comparison of studies on failed back surgery syndrome (reported as %)
Burton et al. [5] | Burton et al. [5] | Long et al. [33]c | Bernard [34]d | Fritsch et al. [2] | Waguespack et al. [35] | Slipman et al. [6] | DePalma et al. [36]i | |
---|---|---|---|---|---|---|---|---|
Surgical | ||||||||
Spinal stenosis, total | 64 | 72 | 5 | 29 | – | – | 21.5 | – |
Stenosis, foraminal | – | – | – | – | – | – | 12.4 | – |
Stenosis, centrala | 7 | 14 | – | 29 | – | – | 5.9 | – |
Stenosis, lateral | 57 | 58 | – | – | 0 | 29 | 3.2 | 0 |
Internal disk disruption/disk degeneration | – | – | – | 29 | 12 | 25f | 21.5h | 11j |
Severe spondylosis | – | – | 5 | – | – | |||
Instability | – | – | – | 2 | 12 | 5 | 0.5 | 0 |
Spondylolisthesis | – | – | – | 4 | 1.6 | |||
Recurrent or retained HNP | 12 | 16 | 1 | 33 | 62e | 11g | 12.4 | 0 |
HNP at a new level | – | – | – | 7 | 22 | – | – | – |
Scoliosis | – | – | 1 | – | – | – | – | – |
Pseudoarthrosis | <5 | – | – | 29 | – | 14 | – | – |
Foreign body | <5 | – | – | – | – | – | – | – |
Surgery performed at the wrong level | <5 | – | – | – | – | – | – | – |
Traumatic meningocele | – | – | 1 | – | – | – | – | – |
Tarsal tunnel syndrome | – | – | 1 | – | – | – | – | – |
Fractured hip | – | – | 1 | – | – | – | – | – |
Compression fracture | – | – | 1 | – | – | – | – | – |
Synovial cyst | – | – | – | – | – | – | 1.1 | – |
Vascular claudication | – | – | – | – | – | – | 1.1 | – |
Pseudomeningocele | – | – | – | – | – | – | 0.5 | – |
Nonsurgical | ||||||||
Arachnoiditis | 6b | 16b | 13 | 11 | – | 1 | 0.5 | 0 |
Epidural or intraneural fibrosis | 6b | 8b | 14 | – | 4 | – | 14.6 | 0 |
Nerve injury during surgery/battered root | <5 | – | 6 | – | – | – | 1.6 | – |
Chronic mechanical pain | <5 | – | – | – | – | – | – | – |
Transitional syndrome | <5 | – | – | – | – | – | – | – |
Unknown | <5 | – | – | – | – | 6 | 5.6 | 0 |
Normal | – | – | 21 | – | – | – | – | – |
Expected postop/minor spondylotic | – | – | 21 | – | – | – | – | – |
Cancer | – | 4 | ||||||
Musculoskeletal abnormality | – | – | 3 | – | – | – | – | – |
Degenerative spondylosis | – | – | – | 9 | – | – | – | – |
Mechanical low back pain | – | – | – | – | – | – | 9.1 | – |
Radiculopathy | – | – | – | – | – | – | 5.4 | – |
Radicular pain | – | – | – | – | – | – | 4.8 | – |
Deconditioning | – | – | – | – | – | – | 3.8 | – |
Facet syndrome | – | – | – | – | – | – | 2.7 | 18 |
Sacroiliac joint syndrome | – | – | – | – | – | – | 1.6 | 43 |
Complex regional pain syndrome | – | – | – | – | – | – | 0.5 | – |
Fibromyalgia | – | – | – | – | – | – | 0.5 | |
Diskitis/infection | – | – | – | – | – | 1 | 0.5 | – |
Hardware/soft tissue irritation | – | – | – | – | – | – | – | 14 |
Neuropathic pain | – | – | – | – | – | 9 | – | 0 |
Psychological | – | – | – | – | – | 3 | – | – |
In the first substantive review on FBSS, Burton et al. [5] reported (see Table 38.2) almost 75 % of the patients with persistent pain had correctable lesions caused either by lateral stenosis (58 %) or retained/recurrent disk herniation (12–16 %). Nonsurgical etiologies diagnosed (without MRI) included: arachnoiditis (6–16 %) and epidural fibrosis (6–8 %). With the introduction of MRI (with and without gadolinium) and diagnostic spine injections, diagnostic sensitivity and specificity improved markedly over the next three decades. Surgeons also responded to this early data with improved surgical techniques or revision strategies to eliminate or reduce certain persistent postsurgical pain problems, such as residual lateral/foraminal stenosis, re-herniation/residual disk herniation, “battered root syndrome”/traumatic neuritis, epidural fibrosis, and arachnoiditis.
Long et al. [33] studied 78 patients admitted to the inpatient Johns Hopkins multidisciplinary pain clinic program for an average of 18 days. Of 1,541 admitted to the pain clinic, two-thirds were noted to suffer from disabling low back and leg pain. The “typical patient” had undergone three spine surgeries and six myelograms and was “misusing narcotics and psychotropic agents.” Approximately one-third of patients were diagnosed with primarily iatrogenic complications from spine surgery. A prospective study of patients admitted from 1979 to 1981 was performed. The average age was 43 years old (range of 19–67 years). The average duration of pain was 7.2 years. The first surgery occurred in the early to mid-1970s. Fifty-three patients (68 %) were involved with disability litigation or receiving disability compensation. This study is the only PLSS study obtained from a pain clinic. Patients were independently evaluated by both a neurosurgeon and orthopedic surgeon. Demographically, this patient cohort represents the “worst of the worst” in terms of persistent pain after lumbar surgery. The authors summarized their findings, noting that 67 % of patients did not meet generally accepted surgical criteria per neurosurgery. Twenty-six patients (33 %) met clinical criteria for surgery. Second surgery criteria were met in 18 patients (40 %). The authors found that 52 patients (67 %) had “persistent pain” of with normal preoperative imaging or showed “nonspecific DDD.” These patients also had significant “underlying psychiatric abnormalities.” Sixteen patients (21 %) had no physical abnormalities to explain their complaint and were classified as normal. Another 16 patients (21 %) had “minor postoperative changes insufficient to cause disabling pain.” Twenty-seven patients (35 %) had a complication from the previous surgery. (Sixty-four patients underwent 171 surgeries; patients underwent an average of 2.6 surgeries.) Thirteen patients (17 %) had spondylotic disease and six patients (8 %) received a new diagnosis. The authors found significant psychiatric pathology to which persistent pain after lumbar surgery was attributed: 10 patients (13 %) had a definitive psychiatric diagnosis, 34 patients (44 %) had a maladaptive personality disorder, and 34 patients were normal. Thus 57 % were classified with significant psychiatric disorders. Sixty-seven patients (86 %) experienced reactive depression. In terms of pain medication used, the authors reported extensive aberrant pain medication usage: 58 patients (74 %) “misused narcotics,” 9 patients had (12 %) “drug addiction,” and 54 (69 %) patients had withdrawal symptoms. In summary, the most common diagnoses given were “normal” in 16 patients (21 %) and “expected postop changes/minor spondylosis” in 16 patients (21 %) and various primary psychiatric disorders. Expected postop changes were defined as: “insufficient to warrant any interventional procedure and did not necessarily explain intractable pain.” Therefore in 42 % of patients, no primary diagnosis and/or no “objective physical cause” was identified to explain pain. The remaining most common diagnoses (total 33 %) were attributed to surgical complications: localized epidural scar, 11 patients (14 %), arachnoiditis, 10 patients (13 %), and traumatic neuritis 5 patients (6 %). (The surgical complication rate among this 78 patient cohort was 12 %.) Six patients had an unexpected source of back/leg pain which was incorrectly diagnosed. Viewed historically, Long et al.’s [33] ability to diagnose the cause of persistent pain suffered greatly from lack of advanced imaging (MRI) and diagnostic spine injections; moreover, many of these patients were given an incorrect primary psychiatric diagnosis for pain and labeled as addicts or substance “misusers.”
Bernard [34] reported on etiologies of PLSS in 45 patients in a single-author practice audit. His diagnostic workup of the etiology of persistent pain was extremely robust – with extensive diagnostic imaging and an average of 2-year follow-up after surgery for correctable causes of pain. The author reported that intrathecally enhanced CT, MRI, and provocative discography with CT scan were critical to obtain a diagnosis; a single imaging study revealed surgical abnormalities in only 61 % of cases. The following are variables found to be statistically significant in predicting a successful repeat surgery: noncompensable injury (p < 0.04), return to work (p < 0.002), and achieving a solid fusion (p < 0.0012). Variables not statistically significant (p > 0.05) are as follows: age, absence of litigation, number of previous operations, psychological diagnosis, and postoperative diagnosis. Bernard’s study made significant contribution in terms of the role of diagnostic imaging to obtain a specific diagnosis. CT myelography was conclusive in 40 % of cases; however, it missed contained disk herniation at 21 adjacent levels. Diagnosis of contained disks can only be shown by provocative discography with CT scan. Intrathecally enhanced CT scanning picked up lateral recess stenosis and small disk herniations overlooked with CT myelography. MRI use in this study was limited due to the recent introduction in the technology. MRI with gadolinium is highly sensitive and specific for distinguishing scar tissue from disk herniations. MRI did show decrease in T2-weighted signal in patient disks; however, provocative discography was necessary to determine if the disk was a true pain generator. Provocative discography disclosed 25 symptomatic contained disks. Lumbar discography was conclusive in 32/34 patients. The author did not find that a “poor psychological profile” predicted an unsuccessful surgical outcome. The author stated: “patients with poor psychological profiles who are experiencing pain may improve when the pain is alleviated” [34]. Thirty-six out of 45 patients underwent fusion for their persistent pain. Fusion was undertaken when the workup revealed recurrent disk herniation, contained painful discogenic disease (with positive discogram), or instability. A solid fusion was achieved in 34/36 patients. Achieving a solid fusion predicted a successful outcome (P < 0.0012). Diagnostic facet blocks or SI joint blocks were not done. It is likely that some degree of symptomatic facet arthropathy was present in this cohort. Fusion addresses more than one pain generator: disk, facet, and instability of the index segment.
Fritsch et al. [2] retrospectively reviewed 182 revisions on FBSS from 1965 to 1990. The authors previously reported a reintervention rate of 10.8 % in 1,500 lumbar diskectomies. A total of 182 revisions were performed on 136 patients; 44 patients (34 %) were revised multiple times. Imaging was used to assist in diagnosis as follows: CT myelography in 54 patients (40 %), CT scan 41 patients (33 %), and MRI 4 patients (4 %). Clearly, MRI now provides much greater diagnostic information than CT scan; moreover, with the dyes previously used in CT myelography, the risk of arachnoiditis was not trivial. Re-intervention was primarily for recurrent or un-influenced sciatic pain and neurologic deficiency. A total of 84 % patients were diagnosed with: true re-occurrent disk herniation (44 %); true reoccurrence and new herniation (22 %); and new herniation (22 %). Four percent (4 %) of patients had epidural fibrosis as a primary diagnosis. The other reason for reoperation was “instability,” reported in 12 % of patients based on history, x-ray, CT, and physical exam findings. Laminectomy performed in the primary surgery was reported as the only factor leading to a higher rate of revisions. Epidural fibrosis and instability increased to greater than 60 % in multiple revision patients.
The next quantitative study on the etiology of long-term failures from lumbar spine surgery was published by Waguespack et al. [35]. This study was the first to employ diagnostic spine injections to precisely identify pain generators. A total of 181 patients with persistent pain after lumbar surgery between 1995 and 1997 underwent extensive diagnostic testing including: high-resolution CT scan, MRI, standing flexion-extension x-rays, discography, additional diagnostic spinal injections, and a psychiatric evaluation. With the advent of MRI and improvements in surgical techniques, the incidence of residual stenosis dropped to 29 % (from 58 % in Burton et al. [5]). Four other etiologies were identified as causes of persistent pain: retained/recurrent disk (7 %), painful disk (17 % at surgical level, 3 % at nonoperated level), neuropathic pain (9 %), and instability (5 %). Recognition of the adverse effects of dyes (i.e., iophendylate (Pantopaque®)) used in CT myelography and the introduction of MRI reduced the incidence of arachnoiditis to very rare. Painful internal disk disruption was increasingly recognized as a significant contributor to persistent axial low back pain through the use of discography (albeit controversial).
Two additional quantitative studies, performed by interventional spine physicians, have since been published. Slipman et al. [6] studied 197 patients and identified 23 various diagnoses. He found that 55 % of patients with persistent pain after lumbar spine surgery had a surgical etiology and that 95 % of patients could be provided a diagnosis. The most common diagnoses were: residual stenosis (21.5 %), retained/recurrent disk (12 %), painful internal disk disruption (22 %), and neural fibrosis (14.5 %). DePalma et al. [36] used modern zygapophyseal joint blocks, sacroiliac joint blocks, provocative discography, and hardware blocks to evaluate 28 patients presenting with primarily persistent axial pain after lumbar fusion. Given this referral population bias, certain types of pain patients were likely referred to other centers for treatment of persistent nonsurgical leg pain with spinal cord stimulators. Evidencing improved diagnosis and treatment of PLSS, DePalma et al. [36] did not diagnose any patient with PLSS from residual stenosis, retained/residual herniation, or epidural fibrosis. He reported the following etiologies of PLSS: sacroiliac pain (43 %), painful internal disk disruption (IDD) (25 %), facet pain (18 %), and hardware pain/soft tissue irritation (14 %). All patients (100 %) received a diagnosis. The early studies by Burton (1981) [37] and Waguespack et al. [35] did not report the sacroiliac joint (SIJ) as a potential pain generator; Slipman et al. [6] reported a 1.6 % incidence of SIJ pain vs. 43 % by DePalma et al. [36]. Painful internal disk disruption incidence stayed relatively stable from 17 % in Waguespack et al. [35], 22 % per Slipman et al. [6], to 25 % with DePalma et al. [36]. All these investigators used provocative discography to diagnose painful internal disk disruption. Facet joint pain also took greater prominence, from no report of this pain generator in the earlier surgical studies to 2.7 % by van Wijk et al. [38] and then 18 % reported by North et al. [39]. Fortin et al. [40] also reported more hardware-related pain than prior investigators (14 %).
38.4 Spinal Fusion and Spine Injections: The Socioeconomic Controversy
To estimate the number of patients per year with persistent pain after lumbar surgery, accurate numbers on the how many spine surgeries are performed per year as well as surgical success rates are needed. In 1997, there were more than 293,000 spine surgeries performed [41] with costs at ~$5 billion dollars. In 2002, not long after the introduction of pedicle screws and the “cage rage” (based on data derived from proprietary sources on the spine industry), more than one million spinal surgeries were being performed per year: 600,000 uninstrumented and 400,000 instrumented cases, respectively [37, 42, 43]. In 2002, orthopedic industry data sources reported a yearly growth rate 3–5 % for uninstrumented cases and 6–8 % for instrumented cases [44–46]. In 2002, the spine market was forecast to compound at 22 % annually due to increased use of spinal instrumentation and aging of the baby boomer generation [37, 46]. By 2004, spinal fusions costs alone generated $16 billion dollars. Researchers critical of the rise in spine surgery noted that the United States had the highest rates of lumbar fusions in the world (see Fig. 38.1), two to five times higher than Sweden, the Netherlands, Australia, and the United Kingdom [47].


Fig. 38.1
Ratios of back surgery rates in selected countries to back surgery rate in the United States (1988–1989) (Adapted from Cherkin et al. [47])
Deyo [48] reported a 220 % increase in lumbar fusions between 1990 and 2000 without an apparent improvement in clinical outcomes [49, 50] (see Fig. 38.2). This 2007, “anti-fusion” publication was widely read and disseminated by the US national media, thus placing spinal fusion under intense scrutiny and apparently single-handedly leading to decrease spinal surgery rates in the United States.


Fig. 38.2
Lumbar spine fusion rates, US National Inpatient Sample (Adapted from Deyo and Mirza [50])
Responding to the increase in spinal fusion rates and costs, policymakers and payors began scrutinizing the medical necessity of lumbar fusion, particularly for degenerative disk disease. In 2010, the insurer CIGNA set a new policy for lumbar fusions: to obtain a lumbar fusion, patients had to first participate in a 6-month, physician-directed functional restoration program with behavioral modification and physical therapy [51]. Policymakers and payors are also looking more closely at the risks of spinal fusion. One researcher reported increased mortality after spinal fusion. Juratli et al. [52] examined mortality after spinal fusion among Washington state injured workers from 1994 to 2001. A total of 2,378 patients underwent lumbar fusion; 103 (4.3 %) were deceased by 2004. Analgesic-related deaths were the number one cause of death among this patient group, responsible for 21 % of all deaths. The risk of analgesic-related death was highest in men ages 45–54 years with degenerative disk disease (rate 7.45) who received instrumentation or intervertebral cage devices. Further investigation is needed into this report of increased mortality after lumbar fusion and to determine if patients receiving instrumentation indeed were at increased risk of analgesic-related death versus patient receiving bone grafts alone.
In 2008, beginning with the Great Recession and a confluence of other factors, the number of spine surgeries began to decline. Factors affecting the decline in number of spine surgeries include multiple publications critical of lumbar fusion [48, 50], increased scrutiny from payors and policymakers on spine surgery rates, costs and outcomes, and increased scrutiny on physician-owned distributorships. By 2011, a total of ~465,000 fusion operations were reported according to the Agency for Healthcare Research and Quality [51]. The research firm GlobalData reported that about 87 % of spinal procedures in 2013 were fusions [51].
According to spine industry analysts, a continued reduction in the number of spinal fusions per year is projected. The Sunshine Act (2013) is expected to inhibit collaboration and innovation between spine surgeons and industry [53]. GlobalData lowered its predicted compound annual growth rate for spinal fusions from 10 % annually to 5 % through 2020 due to multiple factors [54]: stricter reimbursement policies with surgeons reporting more denials for fusion, healthcare reform with mounting economic pressures, loss of physician autonomy over patient care, and a shifting focus to motion-preserving spinal technologies and intradiscal biologics. In January 2014, the Millennium Research Group reported that the global market for spinal non-fusion technologies is expected to triple in size through 2022, surpassing $1.6 billion, driven by emerging markets in Asia Pacific, Brazil, India, and China [54].
Spine interventionists have also come under increasing scrutiny and control due to dramatic increase in spine injections over the last decade. Interventional pain management (IPM) techniques for chronic spinal pain increased markedly from 2000 to 2011 based on data from the CMS (Centers for Medicare & Medicaid Services) [55]. IPM techniques overall increased by 228 % over this interval or 177 % per 100,000 Medicare beneficiaries (see Fig. 38.3). The population growth of this demographic during this interval was 18 %. The highest increases were noted for facet injections and sacroiliac blocks with a total increase of 386 % and 310 % per 100,000 beneficiaries, respectively. Other types of injections reported the following percent increases (total and per 100,000 Medicare beneficiaries): 168 % and 127 % for epidural and adhesiolysis procedures, 150 % and 111 % for other types of nerve blocks, and 28 % and 8 % for percutaneous disk procedures. The geometric annual average was 11.4 % for all IPM procedures with facet injections showing the highest increase at 13.7 % per year.


Fig. 38.3
Illustration of distribution of procedural characteristics by type of procedures from 2000 to 2011 (Adapted from Manchikanti et al. [55])
In 2008, the Office of the Investigator General (OIG) of the US Department of Health and Human Services focused its attention on this explosive rate of growth. Sixty-three percent (63 %) of injections did not meet criteria for medical necessity. Subsequently, facet joint injections have declined by 6 % since 2008. A recent audit by Noridian reported 60–95 % of facet joint injections to be medically unnecessary [56]. The United States Office of Inspector General (OIG) in 2008 [57] reported that Medicare paid over $2 billion in 2006 for IPM procedures. These investigators reported that 63 % of facet joint injection services and 34 % of transforaminal epidural injections did not meet the medical necessity criteria, leading to a total of $175 million of improper payments between 2003 and 2007.
The spinal interventionalist faces many of the same challenges as spine surgeons from payors and policymakers. IPM physicians have to take into account the risks and technical difficulties of with each procedure while keeping in mind the external payor pressure from CMS and the current healthcare cost reform environment. One of the goals of this chapter is to provide the high-quality evidence from the literature for the appropriate and efficacious use of diagnostic and therapeutic spine interventions in the PLSS patient.
38.5 Lumbar Spinal Surgery Outcomes: A Brief Review
Spine surgeons and spine interventionalists are under increased scrutiny for spine surgery and spine injection indications and outcomes. Increasingly, payors and policymakers are dictating how surgeons should diagnose and treat chronic low back pain patients. Spine specialists and their patients will be best served by an evidence-based diagnostic and therapeutic approach to spinal pain to rebut critics. Spine surgeons and interventionalists must independently establish transparent, rigorous indications for spine surgery, continue technical refinements, and publish high-quality literature – or spine care will be increasingly withheld from patients. The lower the reported success rate for primary and revision surgeries, the less likely payors and policymakers will pay for spine surgery. A self-critical look at the definition of success rates is also needed among spine specialists. It is no longer sufficient for an author to personally ask if a patient is satisfied with their surgery. A surgeon’s or spine interventionalist’s results are considered more robust and therefore unbiased, if independent assessment of success is undertaken, along with inclusion of a range of primary outcomes, including: functional status (ODI), reduction in medication use, and return to ADLs/work. Evidence now is also weighted by type of study: retrospective reviews are ranked much lower than randomized controlled trials. In plain terms, to a payor or policymaker, a patient who is not a success is a failure; thus, that term is used in this chapter. However, among spine specialists it is well known that even partial pain relief, when a patient suffers from intractable pain, is meaningful.
Based on literature from the 1960s to 1980s, FBSS is quoted to affect up to 40 % of patients undergoing lumbar spinal surgery [39]. Based on recent data using the most stringent criteria for success, reports of persistent lumbar and/or leg pain after lumbar surgery can range from 20 % to 67 % for microdiskectomy and lumbar fusion, respectively [58, 59]. Patients undergoing microdiskectomy for primary leg pain have the best outcomes. Fusion outcomes vary considerably. Reports of success also vary considerably according to the “view” of the publishing authors, i.e., whether the authors are considered to be “pro-spine surgery” or not. Some surgeons also are very conservative in their use of fusion for DDD. Each study must be scrutinized carefully, particularly in terms of how the authors define “success.” The true prevalence of PLSS is difficult to assess because of the heterogeneous nature of the syndrome, variable definitions of success, and access to public and proprietary data sources for the number, types, and outcomes of surgeries performed each year. Both chronic spinal pain and persistent pain after lumbar surgery present huge individual and societal costs. In an era of increasing healthcare restructuring and “reform,” spine specialist are “awash” in “guidelines” and increasing restrictions on spine surgery and spine injections.
Regarding surgery outcomes, all interested parties question spine surgery studies which report greater than 90–95 % success rates. When Asch et al. [58], a neurosurgeon, used more rigorous criteria for assessing success after microdiskectomy among his patients, he reported leg pain relief (per VAS) at 80 % and low back pain relief at 77 % (success per VAS score defined as pain 0–4; VAS failure pain 5–10). These figures mean 20–23 % of microdiskectomies could be classified as unsuccessful. Santiago-Dieppa et al. (2014) reported on outcomes in older patients with spinal stenosis after non-instrumented arthrodesis, finding a ~60 % incidence of persistent back pain and leg pain with 31 % of patients requiring reoperation over the 7-year follow-up [60]. Regarding lumbar fusion, Fritzell et al. [61] reported a 63 % success rate with 37 % of patients who were unchanged or worse. Using very strict success criteria, from surgeons who perform fusion for DDD as a last resort, success rates are reported as low as 33 % and 43 % [59, 62].
The definition of success after spine surgery remains controversial, with marked variation in criteria applied often lacking independent review. Currently “success” in spine surgery is primarily being defined by authors well known for taking a conservative approach to fusion for DDD. Carragee and Cheng [63] performed a study on 165 patients undergoing spinal fusion to determine a preoperatively determined, patient-acceptable definitions of minimally acceptable outcomes for success. In summary, the patient-acceptable, minimal clinical important difference (MCID) was: at least a 3/10-point decrease in pain, an improvement in Oswestry Disability Index (ODI) of 20 or more*, discontinuation of opioid medication, and return to some occupational activity. Of note, increasing the definition of a successful functional outcome from an 11-point ODI increase to a 20-point increase is appropriate. Historically, spine surgery studies have only set the bar at an 11-point change, which is not a meaningful functional improvement. A 20-point change in ODI is also the most likely to improve a patient by at least one disability grade. The ODI scoring system includes a description of degrees of disability relating to scores. Scores from 0 % to 20 % indicate minimal disability; 20–40 %, moderate disability; 40–60 %, severe disability; 60–80 %, crippled; and 80–100 %, bedbound or exaggerating [64]. Both the Asch and Caragee’s studies are commendable for increasing the bar for a successful functional improvement by raising the ODI to a 20-point change.
Using the most rigorous composite definition of success to date in the surgical literature, Mirza et al. [59] reported success rate of 33 % for spinal fusion for painful internal disk disruption/discogenic pain, thus obtaining a failure rate of 67 % [59]. Published lumbar fusion failure rates range from 30 % to 67 % [60–62, 65–68]. Reports of surgical success rates have come under increasing scrutiny for setting the bar to low [58]. As the bar for success is raised, often by opponents of spinal fusion, published success rates fall. It is generally accepted that success rates for spinal fusion are best for spondylolisthesis (78–91 %) as opposed to degenerative disk disease [62]. Mirza et al. [59] are well known for taking a more “conservative” approach to spinal fusion for DDD. A statistical “composite” definition of success was defined as 30 % improvement in Roland Score, 30 % improvement in pain, and no opioid medication use and working (if relevant). This definition for success appears to be the most stringent presented to date in the spine literature. However, despite the high-bar, surgical intervention was superior to nonsurgical intervention at 1-year. Nonsurgical treatment obtained a 15 % success rate. A more detailed survey of additional lumbar surgery studies is discussed later in the chapter.
The authors of this chapter report the data from the literature with an attempt to provide a “balanced” view of current evidence, from the spectrum of both “positive” and “negative” spine surgery publications, as well as publications between these extremes. This is not a critical review or systematic review of the evidence. Every attempt has been made to identify the group publishing the report as knowing the authors’ surgical approach typically predicts the outcomes they will report. Across the board, outcome reporting obtained by the operating surgeon from the patient underreports pain; therefore, careful attention has been paid to the demographics of the study and the definition of success. Throughout the orthopedic community, surgical outcome reporting has become more stringent out of medical necessity and increased scrutiny and external pressure from non-physician stakeholders in the age of healthcare reform. It is now standard to include functional outcomes (Oswestry Disability Index), medication intake, and return to work. Ultimately, this expansion of the definition of success will benefit both patient and surgeon. To estimate the number of patients with persistent low back and/or leg pain, current outcome data on the most commonly performed surgeries in the lumbar spine are reviewed for: microdiskectomy, spinal stenosis decompression, lumbar fusion, and lumbar total disk arthroplasty. To arrive at best estimates of success after lumbar surgery, this brief focused review of the literature includes recent systematic reviews, randomized controlled trials, and large cohort studies.
38.6 Lumbar Microdiskectomy: Success Rates
For lumbar microdiskectomy, patient satisfaction rates in the literature have varied from 40 % to 98 % [69] with microdiskectomy having an 88–98 % satisfaction rate over open diskectomy. However, in this same study, patient outcomes are not as robust when reported by grade. Patients reported the following outcomes after microdiskectomy: excellent (39 %), good (34 %), satisfactory (19 %), and failed (9 %). Moreover, the longer patients are followed up after spine surgery, the greater decline in satisfaction. Fritsch et al. [2] reported on results of 182 patients undergoing revision diskectomy between 1965 and 1990. In 80 % of the patients, the results were satisfactory in short-term evaluation, decreasing to 22 % in long-term follow-up (2–27 years).
Asch et al. [58] were critical of publications reporting greater 90–95 % success rates after microdiskectomy. The authors concluded that outcome success rates of 75–80 % are more “realistic” than studies claiming greater than or equal to 90–95 % improvement. A prospective study of surgical outcomes in 212 consecutive patients was performed. Independent examiners collected and analyzed outcome data. The author points out that potential bias may be introduced into the literature when outcomes are reported by the operating surgeons and not an independent examiner. Results obtained from independent observers are typically less favorable. More recently, important functional outcome measures have been added to strengthen postsurgical assessments. Asch et al. [58] reported the following: leg pain relief, 80 %; back pain relief, 77 %; improvement in Oswestry Low Back Disability Index, 78 % (ODI <40); satisfaction with surgery results, 76 %; return to normal daily activities, 65 %, and, return to work, 61 %. Worker’s compensation status and increasing age had negative effects on outcome. Based on this data, 20–25 % of patients would have persistent pain after microdiskectomy. Asch et al. [58] estimates are consistent with some of the most recent figures on outcomes after microdiskectomy as reported by other authors. Klessinger [70] reported a 25 % (120/479) prevalence of persistent axial low back pain after lumbar microdiskectomy. A 9 % reoperation rate was reported for recurrent lumbar disk herniation.
McGirt et al. [71] performed a meta-analysis of 54 studies with 13,359 patients comparing incidence of short-term and long-term (2-year) low back or leg pain after aggressive (AD) versus limited diskectomy (LD) between 1980 and 2007. After 2 years the reported incidence of recurrent back or leg pain was 11.6 % for LD versus 27.8 % for AD or a 2.5-fold decrease in persistent pain among patients undergoing limited diskectomy. Aggressive diskectomy involves aggressive removal of the herniated disk material with curettage of the remaining disk. The limited diskectomy technique involves removal of the disk fragment only with minimal incursion into the disk space. The AD technique has been criticized as leading to accelerated disk degeneration with loss of disk height and increased incidence of persistent low back and/or leg pain. Conversely, the LD technique has been criticized for an increased incidence of recurrent disk herniation (RDH). Overall, this paper suggests that ~10–30 % patients may have persistent axial low back pain or leg pain based on the type of microdiskectomy. Repeat surgery is less successful, and according to several studies, only 60–82 % of patients with recurrent disk herniation improve after surgery. In patients who have only epidural scar tissue, the success rate of reintervention is as low as 17–38 % [72, 73]. Recently, Lurie et al. (2014), known to belong to a conservative care spine research group, reported 8-year follow-up results from the Spine Outcomes Research Trial (SPORT) for surgical versus nonsurgical treatment for lumbar disk herniation. Researchers found superior outcomes for pain, physical functions, and ODI in the surgical group [74].
38.7 Non-instrumented Lumbar Fusion for Spinal Stenosis: Success Rates
Santiago-Dieppa et al. [60] reported long-term (median follow-up 7.7 years) outcomes of 376 patients undergoing non-instrumented lumbar arthrodesis at a single institution over a 20-year period. The primary outcomes were: symptom resolution, development of adjacent segment disease (ASD), and need for reoperation. The patient’s mean age was 61 years at presentation with neurogenic claudication due to multi-level spinal stenosis. At follow-up, the presence of back pain decreased from 91.5 % to 61 %; radiculopathy decreased 81–58 %. The cumulative rate of ASD was 18 % (69 patients). The reoperation rate due to non-improvement or worsening of symptoms was 31 % (115 patients), necessitating additional stabilization techniques. Overall, 60 % of patients continued to have back pain, 42 % of patients continued to have leg pain, and 31 % needed reoperation.
38.8 Lumbar Fusion: Success Rates
Studies vary widely regarding lumbar fusion success rates. Rates of success also vary widely based on diagnosis and on the definition of success. Complications after lumbar fusion include: instrument failure (7 %), iliac crest bone-donor site pain (11 %), neural injuries (3 %), and pseudoarthrosis (15 %) [75]. Secondary surgeries for persistent pain or surgery-related complications are reported in 20 % of patients after lumbar surgery [76]. Lumbar spinal surgery success rates drop to 30 % after a second spine surgery, 15 % after a third surgery, and approximately 5 % after the fourth surgery [77].
Philips et al. [65] published a systematic review of 3,060 patients undergoing lumbar spine fusion for CLBP due to degenerative disk disease (DDD). The weighted average improvement in back pain was 37/100, Oswestry Disability Index (ODI) 22, and 12 points on the SF-36 physical component scale. Patient satisfaction averaged 71 %. These results indicate that ~30 % of patients were not satisfied. The reoperation rate was 12.5 %, with 9 % at the index level.
Caragee et al. [62] compared outcomes of fusion for lumbar DDD versus unstable spondylolisthesis. In the spondylolisthesis group, 23 of 32 patients (72 %) met the highly effective success criteria compared to 8 of 30 (27 %) of the presumed discogenic pain cohort. The minimal acceptable outcome was met in 29 of 31 patients (91 %) in the spondylolisthesis group versus 13 of 30 (43 %) of the discogenic pain group. From this data approximately 10 % of patients would be considered a failure after fusion for spondylolisthesis versus 57 % for presumed discogenic pain.
In a randomized controlled trial with 2-year follow-up, Fritzell et al. [61] reported fusion to be superior to usual physical therapy care directed by a family practice doctor in patients with severe axial low back pain of an average of 8 years. All primary outcomes measures favored surgical intervention with statistically significant improvements. Back pain was reduced by 33 % (64–43) or 19-point/~decrease of 2-point VAS in the surgical group vs. 7 % (63–58) in the nonsurgical group. ODI score was reduced 25 % (47–36) or 11 points. In the surgical group, a total of 63 % of patients rated themselves as “much better” (29 %) or “better” (34 %) vs. 29 % in the nonsurgical group. Among fusion patients 29 % rated themselves as “much better” vs. 14 % in the nonsurgical group. The net back to work rate was in favor of surgical treatment versus usual care group, 36 % vs. 13 %, respectively. In total, 37 % of surgical patients failed to respond to the intervention and rated themselves as unchanged (24 %) or worse (14 %). On every metric, surgical patients did better than nonsurgical patients. In patients with an average of 8 years pain, cure is not likely; the objective instead is to meaningfully reduce the amount of the patients’ pain. In summary, substantial improvement was seen in 25–33 % of surgical patients vs. 4–8 % of nonsurgical patients. In addition, depression was reduced by 20 % in the surgical group vs. 7 % in the nonsurgical group. Of note, 28 % of patients randomized to usual care ultimately decided to undergo surgery at 2 years. This study reported surgical treatment of DDD as superior to usual care, 63 % of patients reported being “much better” or “better,” leaving 37 % of patients with no improvement or worse pain. Success in this study was a VAS decrease in 2 points (i.e., from six to four) and an ODI decrease of 11 points. The criteria for success were minimally based compared to more rigorous independent outcome measures. Overall, 29 % of patients rated themselves as much better, 34 % better, 24 % unchanged, and 14 % worse. This data suggests a 38 % failure rate. Three other RCTs, comparing fusion to intensive rehabilitation [78] and cognitive behavioral therapy with exercise [66, 68], did not show fusion to be a superior intervention.
Brox et al. [66] compared lumbar fusion with posterior transpedicular screws (not anterior/posterior fusion) to cognitive behavioral therapy (CBT) and exercise at 1 year, using the ODI as the primary outcome. Patients were ages 25–60 years with low back pain lasting longer than 1 year, imaging evidence of disk degeneration at L4–5 and L5–S1, and ODI score of at least 30 points. The average age of subjects was ~43 years, and duration of low back pain ~10 years; working was 24 % fusion group and 22 % CBT/exercise group; and 70–80 % of patients were either on sick leave, in rehabilitation, or on a disability pension. In the Norwegian healthcare systems, individuals on sick leave more than a year are entitled to a rehabilitation benefit or a disability pension. Approximately 50 % were taking analgesics and ~40 % were smokers. In terms of medical comorbidities, approximately ~70–80 % of subjects had comorbidities not specifically described. We know from the SPORT study that subjects with comorbidities such as diabetes and depression have inferior surgical outcomes [66]. Subjects were randomized to fusion or cognitive behavioral therapy. No difference was observed in ODI between groups at 1-year follow-up with only a 3 % lost to follow-up rate. ODI was reduced from 41 to 26 (15 points) after surgery which was not statistically significant from cognitive behavioral therapy (CBT) and exercise 42–30 (8 points). An independent observer rated success, reporting 70 % after surgery and 76 % after CBT/exercise. The early complication rate in the surgical group was 18 %. Seventy percent (70 %) success would give a 30 % failure rate. Brox et al. [68] also studied the 4-year follow-up results. Fifteen (23 %) patients assigned to surgery underwent reoperation. ODI decreased from 14 points, from 44.1 to 29.7 which was not statistically significantly different between groups. Both randomized groups reported less pain and better function at 9 years vs. baseline; more operated patients were on pain medication and not working. In 2006, Brox et al. [67] published another study comparing instrumented fusion with cognitive behavioral therapy plus exercise in patients with CLBP greater than 1 year. Patients had already undergone a diskectomy and had persistent pain. The primary outcome measure was a reduction in ODI. The fusion group averaged a 9-point ODI decrease from 47 to 38 versus the CBT/exercise group with a 13-point decrease from 45 to 32. At 1-year follow-up, such small ODI decreases represent an unimpressive outcome measure for defining success. The authors reported ~50 % success rate in both groups. This would give a 50 % rate of patients with persistent pain after instrumented lumbar fusion for persistent pain after diskectomy. At 11 years, Mannion et al. [79] were to perform long-term follow-up at 11 years and no difference was found in ODI (−0.7 vs. −0.8) or patient self-reported outcomes between fusion and CBT/exercises groups, respectively. The study had a very high lost to follow-up rate of 45 %. Of note, by 4 years, 25 % of surgical patients underwent reoperation. In summary, in the various RCTs by Brox et al., their early study reported 1-year failure rates or unchanged/worsened pain rate of 30 %; the midterm study reported a “failure rate” of 50 %.
Recently, a community-based study comparing surgical versus nonsurgical treatments for discogenic back pain was published by Mirza et al. [59]. This research group is well known for opposition to the increased rates of lumbar fusion and the use of fusion to treat discogenic pain [50]. Mirza et al. [59] enrolled 495 patients; 86 patients (17 %) underwent surgery. Grading success with a never-before utilized composite criteria (30 % improvement in Roland score, 30 % improvement in pain, no opioid pain medication use and working – if relevant), the 1-year success rate was 33 % for surgery and 15 % for non-structured conservative care. Longer-term follow-up is critical to assess outcomes in the surgical group. Based on 1-year follow-up, 33 % of patients had a successful outcome and 67 % of patients did not meet success criteria.
38.9 Lumbar Disk Arthroplasty: Success Rates
Our data on total disk arthroplasty is limited. Summarized here are two RCTs comparing TDR to circumferential fusion and two different TDR implants. Zigler et al. (2012) reported 5-year results of a prospective, randomized trial comparing circumferential arthrodesis to the ProDisc L for single-level DDD [80]; TDR was noninferior to fusion by a 12.5 % margin. Seventy-seven (77 %) patients in both groups were satisfied with surgery. Both groups had a 48 % decrease in VAS scores and similar ODI decreases [80]. These outcomes suggest similar outcomes between TDR and fusion patients. Patients’ satisfaction was reported as 77 % among both groups. Eighty-three percent (83 %) of TDR patients vs. 68 % of fusion patients stated they would have surgery again.
Guyer et al. (2014) compared two different disk arthroplasty implants with a 2-year follow-up [81]. Success was defined by decrease in VAS, 15-point decrease in ODI, no complications, and lack of reoperation. Success rates were similar: 68 % for the Kineflex L-disc versus 67 % for the Charité Disk. At 2-year follow-up, greater than 90 % of patients in each group were satisfied with outcome. Reoperation was performed in 10.3 % of the investigational group versus 8.4 % of the Charité group. A total of 32–33 % of patients did not meet criteria for success. Likely, the disparity between the high self-reported satisfaction rate versus traditional outcome metrics is due to the novelty of the artificial disk. This phenomenon is not uncommon with the introduction of new drugs or medical devices.
38.10 An Algorithmic Approach to the PLSS Patient
An algorithmic approach to working up residual pain after lumbar spine surgery is useful. The spine surgeon or interventionalist utilizes history, physical exam, imaging, and diagnostic/therapeutic spine injections to identify and treat persistent pain after lumbar surgery. Increasingly, spine and pain specialists are also carefully scrutinizing patient’s comorbidities as treatment of these conditions may help optimize patients for better surgical outcomes.
There are various approaches to diagnosing the cause of PLSS: time from surgery, predominance of back versus leg symptoms, and the three-column approach. Briefly, Crock [82] described two postsurgical failure patterns: “outright failure” and “failure following initial temporary relief.” He emphasized that outright failure is usually related to wrong diagnosis. Immediate failure can also be due to technical error, instrumentation failure, or residual disk. There is temporary relief followed by pain within a few weeks: recurrent disk herniation or infection. There is onset of pain more than 6 months after surgery: re-herniation/new herniation, posterior column pain (facet/SI joint), or epidural fibrosis. Longer-term failures are as follows: loss of stability or stenosis at the surgical site or adjacent site, adjacent facet pain, SIJ pain, painful internal disk disruption (IDD), and pseudoarthrosis. Kostuik [83] (see Table 38.3) summarizes the causes of failure after fusion by time and predominance of back versus leg symptoms. Another straightforward approach is to divide the spine into three columns: posterior, middle, and anterior (see Table 38.4).
Table 38.3
Causes of failure after spinal fusion
Time | Back pain predominates | Leg symptoms |
---|---|---|
Early (weeks) | Infection | Nerve impingement by fixation device or cement |
Wrong level fusion | ||
Insufficient levels fused | ||
Psychosocial distress | ||
Midterm (months) | Pseudoarthrosis | Fixation loose |
Disk disruption | Early adjacent disk degeneration | |
Early adjacent disk degeneration | Graft donor site | |
Inadequate reconditioning | ||
Graft donor site | ||
Long term (years) | Late pseudoarthrosis | Disk with pseudoarthrosis |
Adjacent level instability | Adjacent level stenosis | |
Acquired spondylolysis | Adjacent level disk | |
Abutment symptoms | Stenosis above fusion | |
Compression fracture above fusion |
Table 38.4
Algorithmic approach to the most common causes of residual lumbar and/or leg pain after lumbar surgery by column
Column | Posterior | Middle | Anterior |
---|---|---|---|
Facet joint | Radicular pain/ | Discogenic pain: painful IDD at surgery site or adjacent level | |
Sacroiliac joint | Radiculopathy | Recurrent/residual disk herniation | |
Soft tissue: “fusion disease” | Epidural fibrosis | Pseudoarthrosis | |
Myofascial pain | Transition syndrome/vertical disease | Adjacent segment disease | |
Arachnoiditis |
38.11 History
Accurate history can help identify the cause of pain in many cases. The patient should describe their pre- and postoperative pain in great detail (best to worst pain rating, intermittent versus constant pain, nocturnal pain, pain aggravating or relieved by certain positions, etc.), particularly if there is a change in quality or location. The qualities of nociceptive nerve pain and neuropathic pain are well known to the surgeon. With the 2013 publication of Hannah Albert’s work on Modic type I changes [84], greater attention is being paid to patient’s description of constant, compelling, deep, aching axial pain that does not respond to or is aggravated by usual conservative care such a physical therapy. Dr. Albert asserts that Modic type I changes on MRI are due to disk and endplate infection with Propionibacterium acne s which may be treated with 100 days of oral amoxicillin-clavulanate three times a day.
The examiner should determine whether the patient has primarily axial lumbosacral pain or leg pain or a combination. The workup for primary persistent lumbar pain is different for primary leg pain. The emerging standard for persistent leg pain (with no correctable surgical cause) is referral for spinal cord stimulator. Some consider spinal cord stimulation a first-choice treatment in PLSS due to lumbosacral fibrosis [85]. The reader is referred to outside sources for a more detailed description of this technology. In the United States FBSS is the most common reason for the use of spinal cord stimulation therapy [86]. The analgesic efficacy ranges from 52 % to 72 % and a recent analysis of the national Italian register of implantable systems found that 81 % of the patients reported a positive assessment for pain control with a lowering of drug needs in 71 % of the positive responders [87].
If axial back pain is the principal complaint, the disk, facets, and SI joint must be considered; painful hardware may also be an issue. Revel’s criteria has not been validated or reproduced for predicting facet pain based on correlation with diagnostic facet blocks. Revel’s criteria for facet pain (5/7) are: age greater than 65 years and pain not exacerbated with coughing, not worsened with hyperflexion, not worsened by forward flexion, not worsened when rising from forward flexion, not worsened with extension-rotation, and well relieved by recumbency. Revel’s results have never been duplicated; moreover, to validate his history questions specific to facet pain, he only performed a single diagnostic anesthetic block [88]. The false-positive rate for a single facet block in this setting is 49 % [89]. The gold standard for the diagnosis of facet pain is dual, comparative diagnostic blockade. However, the finding of axial lumbosacral pain below L5 is a sensitive finding on history and is specific for sacroiliac pain and validity by dual-controlled SI joint diagnostic blocks [90]. The examiner should therefore endeavor to identify lumbar versus sacral pain when evaluating axial pain.
Medical comorbidities should be reviewed. Anecdotally, the first author of the study evaluates all chronic low back pain patients, potential primary lumbar surgery patients, and PLSS patients for presence of obesity, prediabetes/type 2 diabetes mellitus, visceral adiposity, metabolic syndrome, subclinical hypothyroidism, hypovitaminosis D, hypogonadism, andropause, menopause, and osteopenia/osteoporosis. If hardware is being considered for a fusion or if the patient has pseudoarthrosis, a recent DEXA scan for bone density is useful to assess bone health. The lower the bone density, from osteopenia to osteoporosis, the less the bone will be able to protect the disk and the greater risk for increased axial loading on the disk and posterior column as well as soft tissues (muscles, fascia, and ligament dysfunction). Hardware is also more likely to fail in osteopenic/osteoporotic patients, both women and men. Many women undergoing back surgery have entered menopause and no longer have the bone protection afforded by estrogen. Surgeons should take a careful history to see if their female patients have undergone surgery and entered surgical menopause or normal menopause. The average age for women to enter menopause is 51 years old. Estrogen is a key hormone for bone metabolism for men and women. Menopause is associated with a loss of bone mineral density (BMD): 10-year cumulative loss was 11 % in the lumbar spine. Serum estradiol (E2) concentrations predict fractures. Older women with total E2 levels <5 pg/ml have a 2.5 increased risk of hip and spine fractures independent of age and body weight; similar associations are found in men [91].
Similarly, we now appreciate that men also experience an age-related decrease in testosterone. Surgeons may consider asking their male patients about loss of vigor, depression, reduced sexual function, and muscle weakness to assess if their male patients have entered andropause. From a lab standpoint, hypogonadism is defined by some authors as a total testosterone less than 400 ng/dL; total testosterone less than 275 ng/dL is frank hypogonadism. Men with suboptimal testosterone also appear to be more prone to osteopenia/osteoporosis. Reduced muscle mass may also impair spine stability and efficacy of postoperative rehabilitation. We are awaiting the 2015 publication results of the Testosterone Trial which is testing the hypothesis that testosterone treatment of men in unequivocally low total serum testosterone (<275 ng/dL) will increase bone density of the spine [91–93].
Surgeons already make sure that their patients stop smoking for at least 6 months or quit altogether; NSAID use is also discouraged. New research shows that there is much more surgeons and bioidentical hormone specialists can do to help achieve optimal outcomes in surgery. This is clearly an emerging field of research. Koerner et al. [94] identified patient factors associated with the best outcome after diskectomy from the Spine Outcomes Research Trial (SPORT). Patient factors associated with the largest improvement in ODI at 4 years with either surgical or nonoperative treatment included: higher baseline ODI, body mass index (BMI) < 30, not being depressed, being insured, having no litigation or worker’s compensation claim pending, and having symptoms for <6 weeks. Patients with diabetes did not benefit with surgery versus nonoperative care. Knowledge of these factors is useful to both patient and surgeon whether further surgical interventions are undertaken or not. Psychosocial issues, depression, anxiety, and so forth should be identified and treated as they can make the primary organic problem more difficult to diagnose and treat. When treating persistent lumbar and/or radicular pain after lumbar surgery, it is prudent to identify and modify as many risk factors as possible: abnormal lab values, obesity, prediabetes, type 2 diabetes, alcohol intake, smoking, depression and hormonal imbalances, and depression. Anecdotally, the first author’s preference is the use of bioidentical hormones instead of synthetic hormones. Some surgeons may consider checking labs to help stratify and optimize their patients for surgical success or sending their patients to a specialist in bioidentical hormone replacement (a full discussion of the pros and cons of bioidentical hormone replacement versus synthetic hormones or traditional agents used to increase bone density is beyond the scope of this chapter). Briefly, some of the most interesting findings in terms of vitamin and hormone function will be presented. The face validity of optimizing hormonal health and thus tissue health, bone strength, and healing capacity (especially under surgical stress such as fusion) would seem to make sense in terms of decreasing the incidence of persistent pain after lumbar surgery. Anecdotally, when potential primary surgery as well as postsurgical candidates present to the first authors’ practice, they undergo a complete hormone and lab panel, to attempt to remove any barriers to healing. The first author has found the abovementioned medical comorbidities to be much more common in spine patients, especially patients with persistent pain after lumbar surgery. This is an area of research in which little research has been published.
Chronic pain patients, including low back pain patients, are known to have insufficient vitamin D 25-hydroxy (vitamin D3) [95, 96]. Severe to moderate deficiency of vitamin D25-OH, defined as <10 ng/mL, was statistically significantly associated with Modic type I changes, with an odds ratio of 0.30 (95 % CI 0.12; 0.75). The other two-thirds of patients had vitamin D25-OH >20 ng/mL which these authors considered “normal.” Many authors disagree with this as the cutoff for normal, let alone “optimal” for bone and muscle healing. Dissenting authors have defined optimal as >50 ng/mL [97].
Briefly, we will discuss vitamin D3 as it is linked to bone health, falls prevention, immune function, cancer protection, and type 2 diabetes. However, controversy remains about the definition of insufficient versus optimal; thus the normal range is defined from 20 to 100 ng/mL. Deficient is based on the author ranging from <20 to <50 ng/mL. Great interest is emerging in having an “optimal” versus “normal” level of vitamin D. Anecdotally, some physicians work to optimize modifiable factors such as vitamin D3 level and prevention of type 2 diabetes, so patients can achieve the best outcomes if undergoing spine surgery. The optimal level for bone health is >50 ng/mL. According to the Third National Health and Nutrition Examination Survey, 61 % of white and 91 % of black Americans suffer from vitamin D insufficiency (25[OH]D <32 ng/mL). Recent studies have demonstrated that a minimum 25(OH)D level of 32 ng/mL is necessary for optimal protection from fracture and intestinal absorption of calcium. Calcium supplements for bone health will be ineffective if a patient is vitamin D deficient [98]. If a patient has cancer or heart disease, some studies recommended obtaining a blood level of 70–100 ng/mL. An intake of 2,000 IU of vitamin D3 will raise the blood level by 20 ng/mL over several months. Some patients may need to take 5,000–8,000 IU of vitamin D3 to obtain these blood levels. The current US Recommended Daily Allowance (USRDA) of 600 IU of vitamin D3 per day is considered inadequate by many; the RDA in Canada is 800–2,000 IU/day [99]. It may be prudent for surgeons considering fusion to optimize vitamin D 25-hydroxy levels to at least 50 at least 3 months before surgery. Lastly, the combination of any low sex steroid hormone (estrogen/testosterone) and 25-hydroxy vitamin D is associated with an increased fracture risk [91].
The majority of patients with PLSS are also on opiate medication which depresses the hypothalamic-pituitary-adrenal (HPA) axis. Valverde-Filho et al. (2014) studied chronic spinal and oral-morphine induced neuroendocrine and metabolic changes in non-cancer patients referred to a pain center [100]. The authors compared three groups, patients with intrathecal morphine pumps (0.2–10 mg/day), patients on oral opiates (60–120 mg/day), and patients receiving non-opioid analgesics. All patient groups experienced improvement in pain scores. Libido, reduced potency, hot flashes, and menstrual cycle dysfunction occurred more often in opiate groups vs. the non-opioid group. Low total testosterone (hypogonadotrophic hypogonadism) was significantly more prevalent in the morphine groups (58 % and 70 %, respectively) vs. non-opioid group (17 %). This group reported clinical symptoms concordant with lab findings. Total cholesterol >200 mg/dL and high-sensitivity CRP (C reactive protein) was significantly more frequent in opiate groups. Lastly, total bone mineral density was below normal in men receiving spinal morphine (p = 0.014). Growth hormone, thyroid-stimulating hormone, and adrenocortical hormones were also suboptimal, but did not reach the same degree of statistical significance.
This author does not recommend statins due to the negative effects on nerve and muscle. At a minimum, statin doses should be the lowest possible “effective dose.” In meta-analyses of RCTs, muscle adverse effects (AEs) are greater with statins versus placebo. Factors such as metabolic syndrome, thyroid disease, and genetic mutations linked to mitochondrial dysfunction can amplify AEs of statins. There is emerging evidence of additional statin-induced AEs such as neuropathy and cognitive impairment [101]. The reader is encouraged to read emerging literature in this area to obtain optimal surgical outcomes in patients, especially patients with persistent neuropathic or radicular pain after lumbar surgery.
38.11.1 Physical Exam
For the PLSS patient, the surgeon repeats a detailed preoperative spine exam with a few additions. Comparison of pre- and postoperative exam findings is essential. New pain generators and possible overlooked pain generators are sought. In some cases the patient may have an entrapment of the superior cluneal nerve (amenable to local surgical release), which travels through an osteofibrous tunnel over the medial iliac crest, approximately 7–8 cm lateral to midline at L4–5. Infrequently, osteoarthritis of the hip can refer to the low back, shin, and calf areas [102]. The presence of low back pain was also statistically more common in patients with longer duration of symptomatic end-stage hip osteoarthritis. Rarely, hip labral tears can also refer to the ischial tuberosity or anterior thigh regions [103]. Hip exam maneuvers for osteoarthritis (i.e., decreased internal rotation) and labral tears/femoral acetabular impingement tests (i.e., flexion-adduction-internal rotation – FAIR test) are critical both preoperatively and postoperatively to screen for less typical hip pain referral patterns. A neurologic exam must be performed to assess for focal deficits, particular residual impairment after disk herniation. If patients have symmetric hypoactive or absent ankle jerks, they likely have a comorbid peripheral neuropathy (most likely due to type 2 diabetes, alcohol, or subclinical hypothyroidism). Abnormalities in each of these areas adversely affect generally healing, especially nerve recovery. If the patient is diabetic, further surgical intervention can be expected to have a statistically significantly inferior outcome compared to nondiabetics. This finding also applies to efficacy of various pharmacologic and interventional spine treatments and is helpful in counseling patients regarding expectations for treatment [94].
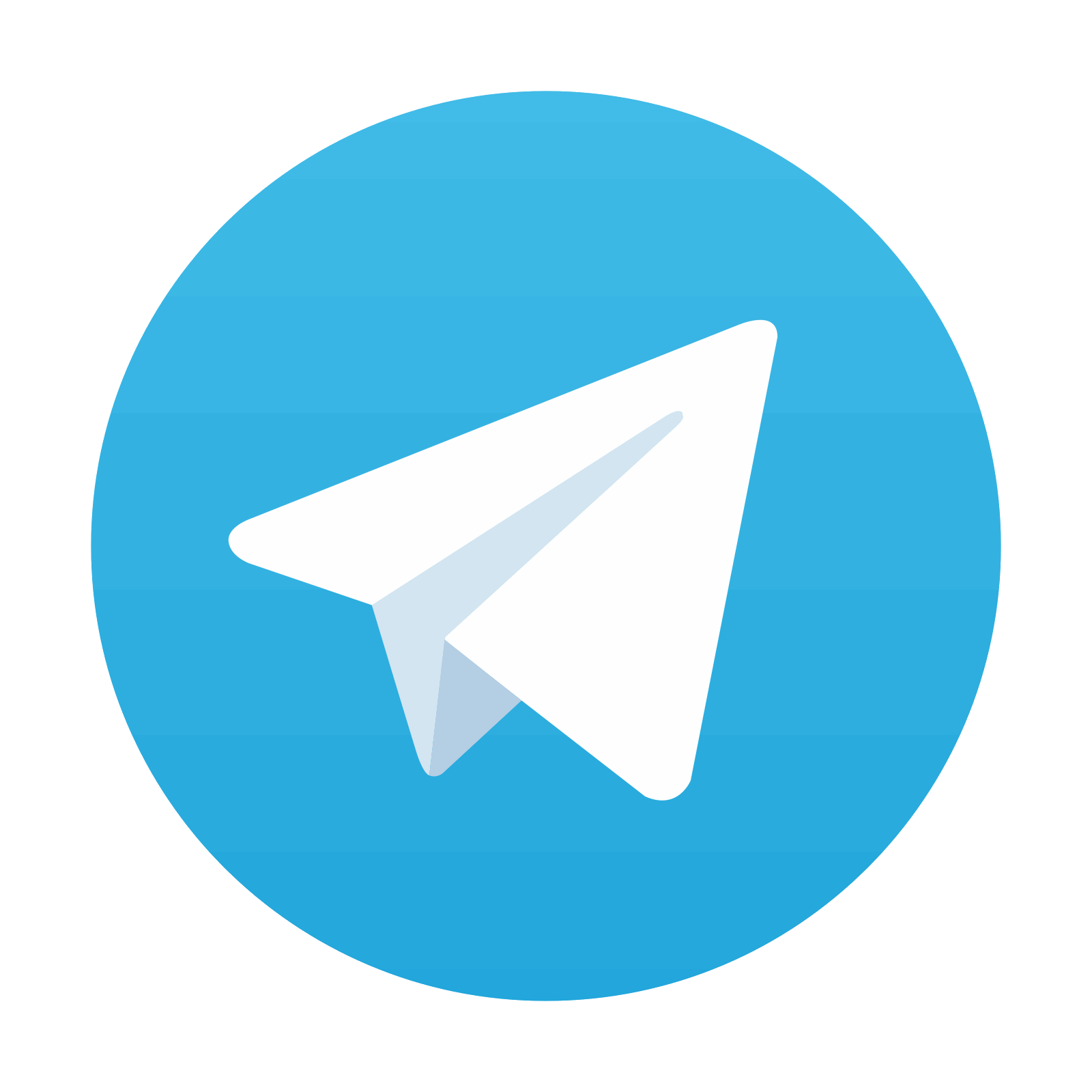
Stay updated, free articles. Join our Telegram channel

Full access? Get Clinical Tree
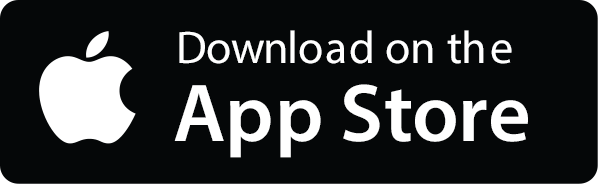
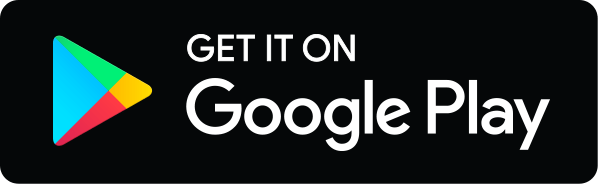