Mean age
55 ± 10 year
Sex
Female
n = 16
Male
n = 12
Spondylolisthesis
Isthmic
n = 15
Degenerative
n = 13
Meyerding
Grade 0
n = 3
Grade I
n = 16
Grade II
n = 9
TLIF segments
L3/4
n = 3
L4/5
n = 12
L5/S1
n = 13
Postop Meyerding
Grade 0
n = 15
Grade I
n = 12
Grade II
n = 1
Clinical improvement (VAS 3–6 months)
Radicular pain
95 %
Back pain
60 %
40.3.3 Surgical Technique
The surgical workflow involves acquiring a CT of the patient’s spine for the preoperative planning stage (Figs. 40.1 and 40.2). In the operating room, the robotic platform is attached to the patient’s spine involving pins to the spinous process and to the iliac crest or to the OR table. Two fluoroscopic images (anteroposterior and 60° oblique to the lateral plane) of the spine and the robotic platform (marked by a 3D fiducial array) are used for registration and matching to the preoperative CT. Following 3D synchronization, the robot is attached to the platform and the robotic arm is dispatched to the calculated trajectories. After stab skin incision (1–1.5 cm) and robot-assisted k-wire insertion, the four pedicle screws are placed percutaneously followed by removal of the robotic system. In the majority of cases, the rod construct is inserted and temporarily tightened. A small midline incision (about 3 cm) is followed by subperiostal placement of the retractor system to gain access to the ipsilateral lamina and facet joint. Microsurgical facetectomy is performed using high-speed burr and rongeurs. Bone may be harvested for graft material. If indicated, ipsilateral and/or bilateral decompression of the dural sac and the exiting nerve root is feasible. Eventually, lateral exposure of the annulus allows for meticulous preparation of the disk space, and implantation of a TLIF cage was performed under fluoroscopic control. This is followed by compression of the screw-rod system and final tightening of the screw caps.



Fig. 40.1
Surgical workflow for SpineAssist. (a) Referencing of the spine-fixated platform prior to robot assembly with marker array and fluoroscopy. (b) Robot-guided trajectory of the percutaneous drill cannula. (c) Intraoperative lateral fluoroscopy with k-wires in L4 and drill cannula directed to L5. (d) Inserted k-wires before screw placement

Fig. 40.2
Example case of a 54-year-old isthmic L5/S1 spondylolisthesis grade I for MIS TLIF using SpineAssist. (a) Preoperative sagittal CT scans. (b) Pedicle screws, placed via SpineAssist on coronal CT scan. (c) Postoperative axial CT scans showing the TLIF cage after right lateral decompression and facetectomy. (d) Postoperative (3 months) skin scarring of 3 cm midline incision and lateral stab incision for percutaneous pedicle screws
Postoperative CT scans were assessed for screw placement accuracy. Ninety-six percent of the overall 120 screws met Gertzbein and Robbins criteria A and B. Misplaced screws (grades C and D, three screws) were located at the lateral pedicle wall but were not associated with neurological deficits as deviance was to the lateral superior aspect of the pedicle. The overall mean operation time was 165 ± 30 min and mean blood loss was assessed to be 162 ± 99 ml, which are on the lower range of the previously reported MIS procedures. Under robot guidance, a mean of 17 ± 7 min was needed for k-wire placement and further 15 ± 8 min for final screw insertion. Radiation time per screw was assessed 4.1 ± 1.9 s and radiation dose was 3.4 ± 2.1 mGy per screw. Observed complications included one patient with postoperative epidural hematoma that needed evacuation and one patient with a L5 syndrome postoperatively that was not related to screw misplacement. No infections were observed.
40.4 Discussion and Future Outlook
The literature is replete with reports of spinal surgery outcomes, yet varies widely in the diagnostic criteria and methods employed to judge clinical efficacy. Image-guided navigation in spinal surgery has demonstrated its feasibility and qualities for spinal instrumentation in numerous studies, but lacks sufficiently powered data to prove the benefit on clinical outcome. Correspondingly, the available, mainly observational, data established a superior accuracy for robot-assisted pedicle instrumentation in the thoracic, lumbar, and sacral spine to conventional surgery along with a reduced radiation exposure. The only two RCTs, however, show inconsistent results [20, 25]. Here, further prospectively and randomized controlled data on accuracy and especially patient outcome is still needed for the evaluation of robotic guidance systems.
Besides, clinical studies have to identify treatment strategies and surgical procedures where robotic systems can realize their potentials best. Minimally invasive and percutaneous procedures represent a reasonable field of application. A growing number of technological advancements have been implemented in this field to enhance the possible benefits of MIS spine surgery, i.e., reduced soft tissue trauma and recovery period, reduced complication rates, and improved outcome. The integration of robotic systems could in addition reduce radiation exposure and accelerate the workflow.
Surgery of the cervicothoracic junction and cervical spine, in which the need for accuracy is crucial, is likewise dependent on fixation implants. The target bone volume is much more delicate and the critical anatomical structures, i.e., the vertebral arteries, the spinal cord, and nerve roots, are in close proximity and their violation is related with significant morbidity. The preliminary application of SpineAssist devices at the cervical spine clearly demonstrated the limitations as the cervical spine displays a high intersegmental mobility and even more vulnerable structures. However, robotic assistance may be helpful in revision cases, which will confront surgeons more frequently due to the increasing number of spinal instrumentation. The altered anatomy and scar tissue in a previously operated situs make orientation more difficult and bears the risk for further complications.
Despite strong evidence for the accuracy of robotic systems, continuous refinement is necessary. For the SpineAssist system, for example, technical hardware and software solutions are meant to enhance the stability of the mounted platform, to minimize the influence of tool deviation due to skiving or soft tissue pressure and to reduce the rate of conversion to conventional manual instrumentation, which in some reports lies between 10 % and 16 % [19, 22].
Future advancements, e.g., including a range of different surgical tools, are conceivable and should be evaluated.
The available surgical robot technology does not only facilitate spinal instrumentation and guided bone work (SpineAssist) but has also demonstrated a high degree of dexterity for soft tissue dissection and preparation in non-openly exposed deep cavities (da Vinci). To combine these robotic philosophies would, at least in theory, potentiate their individual potential.
Integration in other upcoming technologies, e.g., intraoperative imaging modalities such as 3D fluoroscopy and intraoperative CT and MRI, would promote direct intraoperative planning and omit the preoperative preparation steps (imaging and planning), thus further condensing the workflow. Moreover, a gain in accuracy and direct on-site control over the robot-assisted procedures would be feasible.
To connect imaging studies, 3D anatomy, presurgical planning, and in vivo execution through a computerized platform create a new interface that gives essential support but also requires special attention. So far, in a shared-control and hands-on robotic system, the surgeon is the eventual executing factor of the process, which allows but also demands for a continuous reinsurance and validity check of each step. This dichotomy is further continued in the issue to what extent an extension of robotic automation is possible and justifiable. For instance, the tasks of drilling the pedicle and driving-in the screw implants into the sometimes fragile bony structures require tight control of the trajectory axis, which could certainly be performed with high accuracy and precision by a robotic arm. Whether surgeons should leave these critical steps, where the bony anchorage of the implant is prepared and perceived, to the robot computer is debatable.
The question whether the surgeon enables a robot to completely perform delicate positioning of screws remains unanswered. Even markedly reduced, there is an immanent risk of nerve lesion due to the robot, a responsibility bearded solely by the surgeon. The more robots are autonomous, the more likely it is that the responsibility seems to drift away from the surgeon. However, the decision-making process of the surgeon is completely different than that of the robot, as the surgeon additionally incorporates patient’s history, his personal experiences, and intuition to the case.
The financial investment in a surgical robot or other computerized surgical systems is substantial. While navigation systems have been introduced over two decades ago and have proved to produce superior results, only 11 % of spine surgeons responding to a survey reported using such technology in their surgical protocols [52]. Cost-efficiency is a critical factor for the appraisal and adoption of new technologies in the clinical setting, and expectations will increase further. If the clinical value of computer-assisted spinal surgery, for example, in MIS TLIF surgery, which is promising but has to be further validated, consists in significantly reduced hospital stay, reduced re-convalescence and postoperative pain, and thus improved patient outcome, general acceptance and financial compensation will escalate.
Moreover, the growing acceptance of computer-assisted systems, to the degree of newly defined standards of care, will have medicolegal implications for spinal surgery as well. Although experienced spine surgeons achieve high accuracy and excellent results in conventional freehand techniques, the application of these technologies facilitates a detailed documentation of every single step of a surgical procedure, starting from preoperative imaging studies and planning, referencing of the virtual anatomy to the surgical situs, execution according to predefined pathways, and eventually confirmation by intraoperative imaging modalities.
It has become obvious that computer-assisted guidance technology, navigation, and robotics have a significant learning curve for surgeons and OR staff [53]. The preoperative planning, the robot assembly, and referencing do consume extra time that can only be minimized by sufficient training and experience. To reserve the application of these systems for the few really demanding cases seems not advisable. Only use on a daily basis, i.e., for standard instrumentation as well, will provide the necessary experience to enable rapid workflows, short operating times, low complication rates, and eventually satisfactory outcome.
References
1.
Kosmopoulos V, Schizas C. Pedicle screw placement accuracy: a meta-analysis. Spine (Phila Pa 1976). 2007;32:E111–20.CrossRef
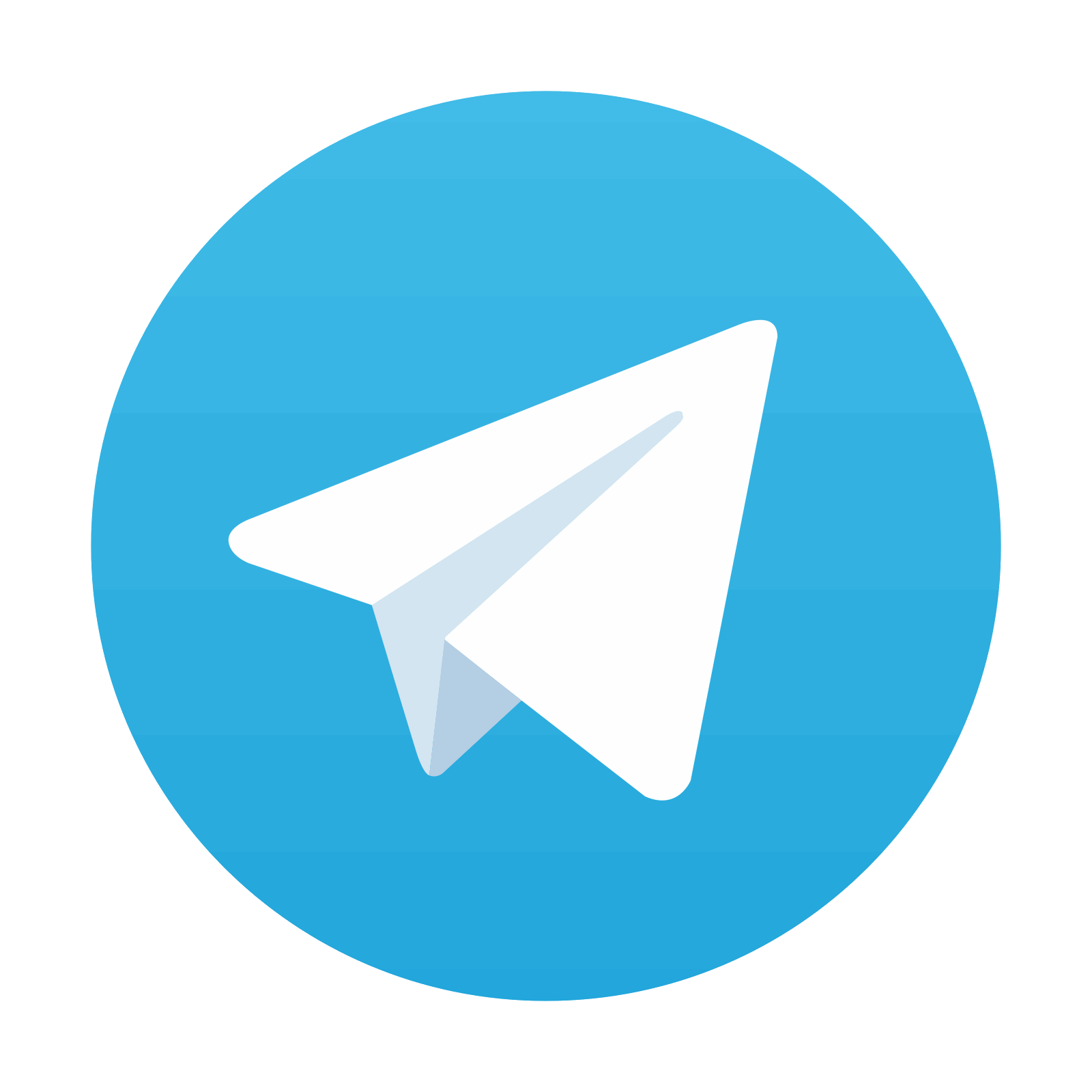
Stay updated, free articles. Join our Telegram channel

Full access? Get Clinical Tree
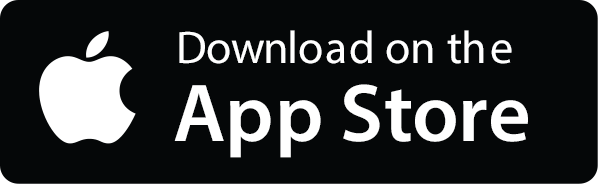
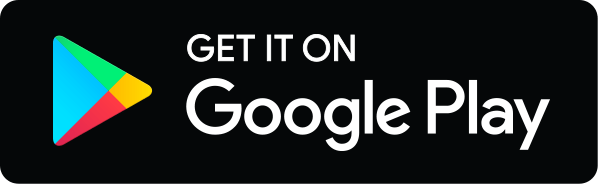