INTRODUCTION
Spinal cord injury (SCI) results from trauma or damage to the spinal cord, causing disruption of communication between the brain and spinal cord to end organs and limbs, with resultant sensory, motor, and autonomic dysfunction. The causes of SCI can be divided into traumatic and nontraumatic. Traumatic causes are typically high-velocity events producing neurologic deficits that occur with an acute and recognizable onset. Nontraumatic injuries tend to have a subacute to chronic course, with slower onset of neurologic deficits.
GENERAL CONSIDERATIONS
Current estimates indicate that between 236,000 and 327,000 people in the United States are living with SCI. There is an estimated annual incidence of SCI, not including those who die at the scene of the accident, of 40 cases per million people in the United States, or approximately 12,000 new cases per year. About 13% of new SCI cases are captured by the National Spinal Cord Injury database, which has a network of SCI model systems that first began collecting data in 1973. There have been 28 SCI model system sites since 1973, and 14 are currently active. From 1973 to 1979, the average age of injury was 28.7 years and most injuries occurred between the ages of 16 and 30 years. Since 2005, the average age of injury have increased to 41.0 years, with 80.6% of injuries occurring in males. Before to 1980, 81.8% of SCIs occurred among males. The incidence of SCI has been decreasing in Caucasians and increasing in minority populations, particularly African Americans and Hispanics. This likely is due, at least in part, to general population trends in the United States. Epidemiologic data for traumatic SCI in British Columbia, Canada, yield similar results, with a median age of injury of 35 years and an 80% gender preference for males. In mainland China, epidemiologic data covering the period from 2001 to 2007 indicated that patients with spinal trauma accounted for 4.58% of all patients hospitalized due to trauma. Among these patients, 69.02% were male and 30.98% were female. The most common causes of spinal trauma were motor vehicle accident (33.6% of SCIs), high falls (31.25%), and falls without height (23.23%), and these causes combined accounted for about 88% of the spinal traumas documented.
Data compiled since 2010 show that motor vehicle collisions account for 36.5% of reported traumatic SCIs, falls for 28.5%, violence for 14.3%, sport injuries for 9.2%, and other or unknown causes for 11.4%. In the category of sports injuries, American high school football players have the highest incidence of severe cervical injuries per year of all high school athletes. Neck and cervical spine fractures accounted for 4.1% of the 517,726 American high school football injuries reviewed from the 2005–2006 season.
Since 2010, the most frequent neurologic category at discharge of patients with SCI is incomplete tetraplegia (40.6%), followed by incomplete paraplegia (18.7%), complete paraplegia (18.0%), and complete tetraplegia (11.6%). Less than 1% of affected individuals experience full neurologic recovery by discharge from hospital. Over the past 15 years the percentage of patients with incomplete tetraplegia has trended upward, with a corresponding decrease in the incidence of complete paraplegia and complete tetraplegia. Currently the causes of death that have the greatest impact on life expectancy of the traumatically spinal cord–injured population are pneumonia and septicemia. The determining factors in statistical life expectancy are age at the time of injury, level of injury, and degree of injury (completeness or incompleteness).
The spinal cord resides in the vertebral canal, the walls of which are defined as follows: the anterior wall border is the posterior longitudinal ligament and the posterior wall border, the ligamentum flavum. The spinal cord is suspended within three layers of meningeal tissue, the innermost being the pia mater and the outermost, the dura mater; in between is the arachnoid mater. The spinal cord itself terminates in the adult no further than the L2 vertebral body, although there can be some variation, with termination occurring as high as T12. The vertebral canal itself continues to the sacral hiatus, just superior to the coccyx. In newborns, however, the spinal cord extends as low as the L3 vertebral body.
There are 33 vertebral bodies in the human spine, divided into 7 cervical, 12 thoracic, 5 lumbar, 5 sacral, and 4 bodies that form the coccyx. They are matched with 31 pairs of peripheral nerve roots, 8 cervical, 12 thoracic, 5 lumbar, 5 sacral, and 1 coccygeal. It is important to distinguish upper and lower motor neurons, as pathologic conditions affecting each of these structures have significantly different clinical presentations. An upper motor neuron (UMN) is a neuron that begins in the cerebral cortex and connects to a motor nucleus in the anterior horn of the spinal cord. A lower motor neuron (LMN) connects with the motor nucleus in the anterior horn and ends as it synapses with skeletal muscle tissue, outside of the spinal cord. The conus medullaris is the distal tip of the spinal cord. The cauda equina (“horse’s tail”) is a bundle of nerves containing the five lumbar nerve roots, the five sacral nerve roots, and the coccygeal nerve root. The nerves of the cauda equina consist of LMNs.
The arterial blood supply of the spinal cord consists of the anterior spinal artery and two posterior spinal arteries. They begin in the posterior cranial fossa as branches of the vertebral arteries. Radicular branches of the anterior and posterior spinal arteries feed individual levels. The artery of Adamkiewicz is a large anterior radicular artery that typically originates between spinal cord segments T9 and L2. There are two watershed areas of the spinal cord, which include the cervicothoracic junction (distal cervical cord through T4) and the thoracolumbar junction.
The spinal cord in cross section reveals the central butterfly-shaped gray matter surrounded by white matter that contains ascending and descending spinal cord tracts, each with specific structural architecture made up of columns of various neural tissues. The deficits associated with specific patterns of injury to the spinal cord are related to the course and location of these tracts
The major tracts discussed in this chapter are depicted in Figure 12–1. The lateral corticospinal tract is composed of descending nerve fibers that are responsible for motor control. Nerve fibers of this tract originate in the cortex of the brain, travel caudally through the midbrain, and decussate at the level of the medulla, with 90% of the fibers crossing to become the lateral corticospinal tract. Of note, a small portion of the descending fibers split to travel both anteriorly and ipsilaterally to become the anterior corticospinal tract and the uncrossed lateral corticospinal tract, respectively. The ascending anterior and lateral spinothalamic tracts convey sensory information to the brain for nociception, temperature, and some simple light touch fibers. These fibers decussate at the ventral white commissure of the spinal cord and ascend a nerve root level before crossing over and continuing their ascension to the cortex of the brain. The dorsal columns (fasciculus gracilis and fasciculus cuneatus) also contain sensory information. These sensory fibers differ from the spinothalamic sensory fibers in that they relay information regarding vibration, proprioception, and light touch. These fibers cross in the medulla as they continue up to the cortex of the brain.
The autonomic system is composed of the sympathetic nervous system and the parasympathetic nervous system. The two systems work in concert to affect many visceral functions, including defecation, heart rate, blood pressure, and respiration, among others. Sympathetic nerves originate from the lateral horn in the thoracic spinal cord and the intermediolateral cell column at spinal levels T1–L3. The parasympathetic nervous system originates from cranial nerves III, VII, IX, and X; the midbrain; and sacral nerve roots.
PATHOGENESIS
The spinal cord is composed of grey matter on the inside surrounded by white matter on the outside. Gray matter at a particular segment provides peripheral output at that level. Subsequently, segmental injury to gray matter results in compromised function at or near that level. White matter includes long ascending and descending tracts. Injury to white matter at any particular level can result in compromised function at all levels below that level.
In traumatic injuries, primary injury to the spinal cord results from compression or laceration of the cord, resulting in disruption of axons and neuronal membranes. This can occur with fractures, dislocations, stab wounds, and gunshot wounds. Secondary injury can occur within 8–24 hours (early) following the primary injury, resulting from a cascade of events that leads to hemorrhage, edema, infarction, and necrosis. Late secondary injury can be seen weeks, months, or years after the initial injury and can result in neuroma formation, wallerian degeneration, arachnoidopathy, and syringomyelia.
In most adults, the spinal cord terminates between the first and second lumbar vertebral bodies. As a result, fractures occurring above L1 frequently result in an SCI characterized by UMN findings. After initial spinal shock (absence of all deep tendon reflexes), increased tone may be seen, manifested by brisk reflexes or involuntary spasms, or both. Fractures at and below L2 frequently result in a cauda equina injury, characterized by LMN symptoms (hypotonia, flaccidity, diminished or absent reflexes, muscle atrophy, flaccid bowel and bladder). Fractures at the level of L1 or L2 frequently affect the conus medullaris, and if nerve root injury also occurs, can present with a mixed UMN and LMN presentation.
Bearing in mind the anatomy of the spinal cord, most SCIs result from cervical- or thoracic-level injuries rather than lumbar- or sacral-level injuries. There are several notable cervical-level fracture–dislocations. Atlantooccipital and atlantoaxial dislocations usually are fatal. Of note, individuals with rheumatoid disease, Down syndrome, and congenital skeletal dysplasias are at significantly increased risk of atlantoaxial instability and subluxation. A burst fracture of C1 is called a Jefferson fracture. Fractures involving the odontoid process, or dens, are classified based on the areas of fracture involvement. In type 1, fractures are limited to the tip of the dens (and surgery is frequently not needed). Type 2 odontoid fractures involve the base of the dens or junction with the vertebral body of C2; this is considered an unstable fracture and generally requires surgical fixation. The rate of nonunion with external fixation alone is upward of 60%. Type 3 odontoid fractures involve the body of C2 and frequently can be managed nonsurgically (90% of fractures heal with use of a halo device for 3 months). Hyperextension of the cervical spine can result in SCI (classically known as central cord syndrome, in which upper extremities are weaker than lower extremities). C4–5 is the most common level at which cervical hyperextension injuries occur. Compression fractures of the cervical spine result from cervical flexion or axial loading. C5 is the level at which cervical compression fracture most commonly occurs. Unilateral facet joint dislocation can occur from a cervical flexion–rotation injury. Bilateral facet joint dislocations occur from cervical flexion injuries. C5–6 is the most common level for both unilateral and bilateral facet joint dislocations.
Injuries to the thoracolumbar spine are most commonly seen with motor vehicle accidents. Other organ injury is seen in up to 50% of individuals with thoracolumbar trauma.
There are many nontraumatic causes of spinal cord injury, including spinal stenosis with myelopathy, spinal neoplasms, spinal vascular disease, infections, degenerative and demyelinating diseases, and toxic and metabolic myelopathies.
Spondylosis and spondylolisthesis of the spine can cause compression of the spinal cord. This is more frequently seen in the cervical spine but can also affect the thoracolumbar spine. Depending on the location of the stenosis (central or neuroforaminal), one may see more UMN presentations with hyperreflexia and spastic muscle weakness in myelopathy or LMN presentations with hyporeflexia and flaccid muscle weakness in radiculopathy.
Spinal neoplasms can affect the spinal cord either directly (primary spinal cord tumor) or indirectly (spinal column tumor causing compression of the spinal cord). The most common neoplasms of the spinal cord are intradural primary tumors (nerve sheath tumors, eg, schwannomas and neurofibromas; meningiomas; ependymomas; and astrocytomas). However, metastatic disease (from lymphoma, lung, breast, and prostate cancer) to the spinal column that secondarily compresses the spinal cord occurs almost 25 times more commonly than primary spinal cord tumors.
Spinal vascular disease includes spinal arterial infarction (most often as a consequence of profound systemic circulatory impairment, surgical cross-clamping, or disease of the aorta or its major branches), spinal venous infarction (most commonly in hypercoagulable states), spinal vascular embolic disease (most commonly from atheromatous or fibrocartilaginous embolism), and spinal vascular malformations (usually manifesting acutely due to hemorrhage).
Infections affecting the spinal cord can include abscesses (epidural, subdural, or intramedullary), suppurative spinal leptomeningitis (accompanying intracranial meningitis), Pott’s disease (tuberculosis of the spine), tabes dorsalis (neurosyphilis), and viral myelitis or myelopathy (poliovirus, West Nile virus, HIV).
Degenerative or demyelinating diseases include multiple sclerosis, amyotrophic lateral sclerosis (ALS), primary lateral sclerosis, spinal muscular atrophy, Friedreich’s ataxia, and hereditary spastic paraplegia.
Toxic or metabolic myelopathies can be seen in cases of vitamin B12 deficiency, postintrathecal injection (with anesthetics, alcohols, hypertonic saline, steroids, or chemotherapeutic agents), and postangiography.
CLINICAL FINDINGS IN ACUTE SPINAL CORD INJURY
Severe SCI is associated with an immediate rise in blood pressure; however, this stage is brief and is followed by spinal shock, characterized by hypotension, bradycardia, and hypothermia. Hypotension can also result from other types of shock and should be distinguished and treated accordingly. Spinal shock is caused by decreased tone and lack of reflexes below the level of the SCI, and the drop in blood pressure is related to changes in the sympathetic tone of the vasculature. Various signs have been identified to signal that a patient is coming out of spinal shock. This transition can occur anytime from 24 hours to 3 months post-SCI and includes disappearance of the delayed plantar reflex, appearance of cutaneous reflexes (eg, bulbocavernosus and cremasteric reflexes), return of deep tendon reflexes, and return of reflexive detrusor function.
Ditunno and colleagues have proposed that spinal shock be divided into four phases. Phase 1 occurs over day 0–1 and is marked by areflexia or hyporeflexia attributed to a loss of descending nervous stimulation. It has also been postulated that the lack of reflexes and flaccidity noted are due to hyperpolarization of the nerves caudal to the SCI.
Phase 2 starts on days 1–3 and is marked by the initial return of reflexes, attributed to supersensitivity of spinal cord tissue below the level of denervation. Proposed mechanisms for this supersensitivity include reduced excitatory neurotransmitter reuptake, increased synthesis and insertion of neurotransmitter receptors into the postsynaptic membrane, decreased degradation of the postsynaptic receptors, and altered synthesis and composition of receptor subunits. The first reflex to return in this phase is the delayed plantar reflex, which has been observed as early as several hours after the injury. Persistence of this reflex beyond 7 days is associated with poor prognosis for ambulation and motor recovery. Additional reflexes tend to return in the following sequence: bulbocavernosus reflex, cremasteric reflex, ankle jerk, Babinski sign, and knee jerk. In general, cutaneous polysynaptic reflexes are recovered before deep tendon reflexes.
Phase 3 spans weeks 1–4 and is characterized by hyperreflexia attributed to new synaptic growth. Considerable variability is seen in the return of reflexes during this phase. Autonomic function also improves, with improvements in vagus-mediated bradyarrhythmias and hypotension as well as the emergence of autonomic dysreflexia.
Phase 4 occurs over months 1–12 and is characterized by further hyperreflexia attributed to soma-supported synaptic growth, particularly of long axons. Cutaneous reflexes become hyperactive. Detrusor tone has been noted on urodynamic studies to improve at about 4–6 weeks after injury, although this may not correlate clinically with improved function of the bladder.
Associated with acute SCI are arrhythmias, most commonly bradycardia, although supraventricular tachycardia and ventricular tachycardia have also been reported. The proposed mechanism for bradycardia is disruption of the autonomic tone causing an excess of parasympathetic tone to be exerted on the myocardium. Hypotension is also commonly seen due to loss of vasoconstrictor tone and peripheral arterials, thus causing pooling of blood in the peripheral vasculature. There is often loss of bowel control and urinary retention due to an atonic and flaccid bladder acutely. Priapism is commonly noted immediately following a complete SCI and typically resolves in 1–2 days. It tends to be of a high flow, arterial, nature and is self-limited. Female patients are often amenorrheic after SCI.
CCS is the most common of the incomplete SCI syndromes and results from cervical cord injury. It comprises 50% of incomplete injuries and 9% of all traumatic SCIs. Individuals with CCS present with greater weakness in the upper limbs than in the lower limbs, and often with bladder dysfunction and variable sensory loss. Most commonly CCS occurs in elderly individuals owing to hyperextension injury of a degenerative cervical spine, often as the result of a fall with a blow to front of head. CCS can occur with or without fracture or dislocation of vertebrae. The suspected mechanism of injury involves compression of the cord anteriorly by degenerative bony and disc components, and posteriorly by an inward bulging of the ligamentum flavum in a narrowed spinal canal. The exact cause of this specific pattern of injury is unclear. There is evidence that it is not related to the center of the spinal cord directly. Patients younger than age 50 tend to have a better prognosis for independent ambulation and return of bowel and bladder function. The pattern of recovery typically begins with recovery of strength and sensation in the lower limbs, followed by bowel and bladder recovery; finally, proximal and then distal upper limb strength and sensation recover.
Cruciate paralysis is associated with fractures of the C1 and C2 vertebrae, with neurologic compromise at the cervical medullary junction. The pattern of deficits is almost exclusively limited to the upper limbs, with minimal or no lower limb deficits found. Most patients recover completely. There is no widely accepted explanation for this pattern of deficits.
BSS refers to hemisection of the spinal cord that produces a characteristic pattern of deficits. Two to four percent of all traumatic SCIs are in a Brown–Séquard or Brown–Séquard-like pattern of injury. Classically, BBS is associated with knife or gunshot injury but it can result from any damage that causes hemisection of the spinal cord. The classic pattern of deficits is ipsilateral flaccid paralysis at the level of injury, ipsilateral loss of position and vibratory sense below the level of injury (dorsal column), ipsilateral UMN findings below the level of injury (cortical spinal tract), and contralateral loss of pain and temperature below the level of injury (spinal thalamic tract). Injuries that contain some elements of this pattern but not the complete pattern are referred to as Brown–Séquard “-like” or “-plus” syndrome. Of all SCI syndromes, BSS is associated with the greatest chance of recovery, with 75–90% of patients able to ambulate independently and 70% independently performing activities of daily living (ADLs) at discharge from an inpatient rehabilitation facility. Greater than 80% of these patients regain bowel and bladder continence. The recovery of muscle function correlates with pain and temperature sensory deficits in the given area. Typically, ipsilateral proximal extensors recover first and distal flexor muscles, last.
ACS is a pattern of injury that affects the anterior two thirds of the spinal cord, sparing the posterior columns. Most often, ACS results from vascular compromise of the spinal cord, but other causes include retropulsed discs or bone fragments. The pattern of deficits involves loss of motor function and pinprick sensation; light touch sensation is preserved, as is proprioception and deep pressure sensation. This condition carries a poor prognosis, with only 10–20% of patients showing some degree of motor recovery.
PCS is the least common of the incomplete SCI syndromes. It typically involves loss of dorsal column function, including light touch, proprioception, and deep pressure sensation, with preservation of pain, temperature, and light touch sensation and varying degrees of motor function. On physical examination, Lhermitte’s sign may be present. PCS may be caused by trauma but is also associated with various metabolic and infectious diseases, such as advanced syphilis and vitamin B12 deficiency. It carries a poor prognosis of recovery.
This SCI involves the segment above the conus medullaris, consisting of L4–S1 cord segments, with sparing of the reflex function of the sacral segments. It manifests as a UMN lesion of L4–S1.
Conus medullaris syndrome involves the terminal end of the spinal cord between the L1 and L2 vertebrae. It is typically a symmetric LMN lesion of the S2–4 nerve roots. Pain is less common than in cauda equina syndrome, although low back pain may be present. Patients present with a flaccid bladder and rectum. Lower limb strength may be preserved or may be flaccid with findings typical of an LMN lesion. Bowel and bladder are typically areflexic with LMN dysfunction.
This syndrome involves the segments below the L1–2 vertebral level, affecting the nerve roots referred to as the cauda equina (see Anatomic Considerations, earlier). This syndrome can be thought of as multiple radiculopathies and is characterized by flaccid paralysis and atrophy of the lower limbs. Although sensation may be preserved, it is often painful. The lesion is usually asymmetric. Cauda equina syndrome carries a better prognosis than UMN lesions and often occurs in combination with conus medullaris syndrome. Bowel and bladder are typically areflexic with LMN dysfunction.
Diagnosis of SCI incorporates pertinent information from the history and physical examination, with a focus on elements of trauma to the neural axis and identification of risk factors for SCI. These risk factors include a history of malignancy, epidural abscesses and hemorrhage, and exposure to infections of the spinal cord. Physical examination should include identification of UMN signs in areas innervated caudal to the cranial nerves, as well as changes in sensation, motor strength, and autonomic function in these areas. Physical examination may also be used to assess for findings associated with the underlying cause of the SCI and any concomitant injuries, in the case of traumatic event.
Radiographic and computed tomography (CT) scans allow for quick evaluation of the bony spinal column and can assist in localization of injury and provide information about the stability of the spine. Disruption of the thoracolumbar spine is often stated in terms of the three columns of the spine, as delineated by Denis. Using this approach, the anterior column is viewed as comprising the anterior longitudinal ligament and the anterior two thirds of the vertebral body; the middle column as comprising the posterior one third of the vertebral body and the posterior longitudinal ligament; and the posterior column as comprising the facet joints, ligamentum flavum, and remaining interspinous ligaments. Disruption of two or more of the columns on imaging is considered an unstable spine, requiring stabilization before mobility is permitted.
Radiographic and CT scans can also be used to evaluate for underlying causes of the SCI, such as malignancy of the vertebrae, vascular malformations, infections, or hematomas. Magnetic resonance imaging (MRI) is the ideal modality for viewing the spinal cord, as it is particularly useful for evaluation of soft tissues. It is less sensitive than CT scan to fractures of the vertebrae; however, edema in vertebrae seen on MRI has a high correlation with fracture. MRI is not useful in prognosticating functional outcomes of SCI, but hemorrhagic changes of the spinal cord on MRI correlate with a poor probability of neurologic recovery. Spinal cord injury without radiographic abnormality (SCIWORA) is typically noted in children due to many factors, including large head-to-neck ratio and increased flexibility of the pediatric spine (see later discussion). Consequently, in a high-velocity injury the spine may flex or extend, causing cord damage without injury to vertebrae. SCIWORA in the elderly typically results from a cervical hyperextension force and produces injury to the spinal cord without overt bony fracture or dislocation. As MRI becomes increasingly more sensitive to injuries of the spinal cord, SCIWORA is becoming less common.
Neurologic assessment of a person with traumatic SCI is best done using a standardized neurologic examination as endorsed by the International Standards for Neurological Classification of Spinal Cord Injury (ISNSCI), known as the International Standards (Figure 12–2). The American Spinal Injury Association (ASIA) has developed a web-based training course called InStep, located at www.asialearningcenter.com, to assist in learning how to perform the examination.
Figure 12–2
American Spinal Injury Association International Standards for Neurological Classification of Spinal Cord Injury, 2011. (Reproduced, with permission, from the American Spinal Injury Association: International Standards for Neurological Classification of Spinal Cord Injury, Atlanta, GA; Reprinted 2013.)
The examination consists of two main components, a motor examination and a sensory examination. The latter involves testing for sensation to pinprick and to light touch. Additionally, a rectal examination is performed to test for voluntary anal contraction and sensation to deep anal pressure. Motor strength is a reflection of corticospinal tract integrity, and sensation to pinprick and light touch reflect the functions of the spinothalamic tract and dorsal columns, respectively. Twenty-eight key dermatomes are tested for pinprick and light touch from C2 through S4–5, and 10 key muscle groups are tested for strength from C5 to T1 and from L2 to S1, as outlined in Table 12–1.
Root Level | Muscle Group | Muscles |
---|---|---|
C5 | Elbow flexors | Biceps, brachials |
C6 | Wrist extensors | Extensor carpi radialis longus and brevis |
C7 | Elbow extensors | Triceps |
C8 | Long finger flexors (distal phalanx of middle finger) | Flexor digitorum profundus |
T1 | Finger abductors (little finger) | Abductor digiti minimi |
L2 | Hip flexors | Iliopsoas |
L3 | Knee extensors | Quadriceps |
L4 | Ankle dorsiflexors | Tibialis anterior |
L5 | Long toe extensor | Extensor hallucis longus |
S1 | Ankle plantar flexors | Gastrocnemius, soleus |
Sensation is graded on a three-point scale, from 0 to 2, where 0 represents “absent,” 1 represents “altered” (either diminished or enhanced), and 2 represents “normal.” The control point for sensation is the face, assuming cranial nerves are intact. Motor strength is graded on a six-point scale, from 0 to 5, where 0 is used to represent no movement; 1 is visible or palpable twitch, 2 is active movement through full range of motion (ROM) with gravity eliminated; 3 is active movement through full available ROM against gravity; 4 is active movement through full available ROM with moderate resistance; and 5 is active movement through full available ROM with full resistance. The total number of motor points is obtained by adding the motor strength scores for the upper as well as lower limbs. Similarly, a score is obtained for sensation by adding up the total scores for each dermatome.
The neurologic examination yields several key levels that assist in classification and functional prognostication. The motor level is defined as the most caudal level with a muscle grade of 3 out of 5 or greater with all key muscle groups above this level having a muscle grade 5 out of 5. The sensory level is the most caudal level with normal (2 out of 2) sensation to both light touch and pinprick. These levels can be further divided into right and left. The neurologic level of injury (NLI) is the most caudal level at which both motor and sensory function is intact bilaterally. Paraplegia is defined by injury from T1 and below (caudal), with the upper limbs intact. Tetraplegia results from injuries above T1, with all limbs being affected.
The ASIA Impairment Scale (AIS) classifies SCI as complete or incomplete, using categories A through E as detailed in Table 12–2. This classification schema is helpful for communication and prognostication.
Category | Description | |
---|---|---|
A | Complete | No motor or sensory function is preserved in sacral segments S4–5 |
B | Incomplete | Sensory but not motor function is preserved below the NLI (either deep anal pressure or PP or LT at S4–5) |
C | Incomplete | Motor function is preserved below the NLIa and more than half the key muscles below the NLI have a muscle grade < 3 |
D | Incomplete | Motor function is preserved below the NLIa and at least half (or greater) the key muscles below the NLI have a muscle grade of ≥ 3 |
E | Normal (full recovery) | Motor and sensory function are intact |
Developed in 2009, the Autonomic Standard Assessment Form is a means of documenting the autonomic nervous system in the context of SCI. The grading scale is divided into three parts: general autonomic function, which evaluates autonomic control of cardiac function, temperature regulation, and autonomic and somatic control of the bronchopulmonary system; (2) lower urinary tract, bowel, and sexual function; and (3) urodynamic evaluation.
TREATMENT
The management of an acute SCI begins in the field. Emergency personnel should immobilize all suspected SCIs using a hard cervical collar and secure patients on a hard backboard. Spinal immobilization should be continued if SCI is confirmed until definitive treatment occurs. In traumatic events, a concomitant brain injury should be suspected in a patient with an altered level of consciousness. Other injuries commonly associated with SCI include fractures, intraabdominal and pelvic injuries, and pulmonary contusions and hemorrhage.
As with all traumatic injuries, an immediate assessment of the patient’s airway, respiration, and circulation should occur. Should the patient require intubation due to airway or respiratory complications or to the level of injury itself, the jaw-thrust maneuver should be used to prevent further injury to the spine.
Emergency personnel should be mindful of potential cardiopulmonary compromise associated with SCIs. Hypotension commonly occurs. Hemorrhage must be ruled out as a potential cause of low blood pressure. Next, neurogenic shock (bradycardia, hypotension, and peripheral vasodilation) should be considered as a culprit. The treatment of neurogenic shock starts with fluid resuscitation, typically an isotonic crystalloid solution, with a goal systolic blood pressure of 90–100 mm Hg. Care providers must be cautious to avoid flash pulmonary edema resulting from overadministration of intravenous fluids. Atropine may be used for significant bradycardia.
The use of corticosteroids in the setting of acute SCI has been the focus of much debate. The National Acute Spinal Cord Injury Study (NASCIS) trials I, II, and III were completed in the United States in an attempt to demonstrate functional gains after the administration of methylprednisolone acutely. In NASCIS I, it was determined that the dose of steroid used was inadequate. However, statistically significant adverse effects of steroid usage were still noted. NASCIS I laid the foundation for the following two trials. In NASCIS II, methylprednisolone (30 mg/kg bolus followed by 5.4 mg/kg per hour over 23 hours) was compared with naloxone (5.4 mg/kg bolus followed by 4.5 mg/kg per hour over 23 hours) and placebo. NASCIS II was a negative study. However, post-hoc analysis revealed that patients treated with steroid within 8 hours of injury had statistically significant motor recovery, which lasted up to the final assessment at 1 year. Of note, this motor recovery was defined by an improvement of only 5 motor points. Lastly, NASCIS III, completed in 1997, compared tirilazad (an antioxidant agent) with methylprednisolone (5.4 mg/kg per hour) given over both 24 hours and 48 hours. All patients received an initial bolus of methylprednisolone. This was also a negative study. As with NASCIS II, post-hoc analysis revealed a 5-point motor recovery at 1 year. However, Functional Independence Measure (FIM) scores remained unchanged at 1 year.
As stated in the clinical practice guidelines for health care professionals, “no clinical evidence exists to definitively recommend the use of any neuroprotective pharmacologic agent, including steroids, in the treatment of acute SCI in order to improve functional recovery.” Until recently, the Congress of Neurological Surgeons (CNS) held that physicians who utilize steroids in the treatment of acute SCI should be aware that the known adverse side effects of these agents outnumber any suggested benefits. In March 2013, the CNS released a new statement, indicating that “Administration of methylprednisolone (MP) for the treatment of acute SCI is not recommended” due to lack of clinical evidence to support its use. Despite the lack of substantiation, the use of methylprednisolone in acute SCI has been common practice in many institutions. This may change, however, in response to the CNS’s definitive statement. If steroids are utilized acutely, the NASCIS trials are used as a guide for dosing and treatment length.
Once SCI is diagnosed in an individual with an interrupted spinal column, consideration of definitive treatment begins. Two routes for intervention exist: bracing and surgical intervention. The goal of either treatment is a painless, balanced, stable spine with optimum neurologic function and maximum spinal mobility. Nonoperative management is indicated for stable injuries that do not have the potential for progressive deformity or neurologic injury. Many bracing options exist. The type of brace chosen depends on the level of injury requiring relative immobilization. The principle of bracing is to provide a force vector opposite to that of the injury. Some key bracing options are listed in Table 12–3. For more details, refer to Chapter 28.
Level of Injury | Bracing Options |
---|---|
High cervical | Halo: offers flexion, extension, and rotational control of the cervical spine; used in management of unstable injuries. |
Mid cervical | Philadelphia collar and Miami J: indicated for stable injuries only; provide flexion and extension control. |
Low cervical or high thoracic | Sternooccipital mandibular immobilizer (SOMI): indicated for stable cervical spine fractures or for use after cervical spine fusion. Minerva: may be used in management of unstable cervical injuries. |
Thoracolumbar | Thoracolumbar sacral orthosis (TLSO): provides flexion, extension, side bending, and rotational control. Cruciform anterior spinal hyperextension (CASH) and Jewett: primarily provide flexion control; often used in management of compression fractures. |
Indications for surgical intervention include the need for spinal cord decompression or for spinal stabilization in patients with significant neurologic deficits or with unstable spinal injuries. Examples of injuries that commonly require surgical intervention include fracture–dislocations, flexion–distraction injuries, and burst fractures with neurologic deficits. Relative contraindications to operative intervention include complete SCI for more than 24 hours and a medically unstable patient.
Experimental treatments to decrease or halt acute and late phase spinal cord damage are beyond the scope of this chapter.
The rehabilitation of patients with SCI should begin in the acute care setting and continue in a specialized spinal cord rehabilitation unit once medical and spinal stability are achieved. The rehabilitation approach is interdisciplinary by nature. The team commonly consists of physical therapists, occupational therapists, speech language pathologists, psychologists, case managers, rehabilitation nurses, physiatrists, and consultants.
The goals of rehabilitation are to maintain medical stability, address mobility and ADLs, educate and train the patient and his or her family, plan for discharge (including recommending home modifications, if necessary), and prescribe durable medical equipment. Functional goals for the patient depend on the motor level of injury. Key motor levels of injury and associated potential functional goals are highlighted in Table 12–4. Rehabilitation specialists use numerous techniques and devices to assist their patients in gaining functional independence. A brief survey of notable methods follows.
Level of Injury | Functional Goals |
---|---|
C5 | Independent with feeding after setup using adaptive equipment |
C7 | Independent with bed mobility and transfers; independent at wheelchair level of mobility; can drive with hand controls |
C8–T1 | Independent with all self-care using adaptive equipment |
T12–L4 | Ambulates with bilateral lower extremity orthotics and assistive device |
L4–5 | Ambulates with bilateral lower extremity orthotics |
Functional electrical stimulation (FES) works with intact LMNs and peripheral nerves to stimulate paralyzed muscles. FES can be used to stimulate and pace the diaphragm, to aid in erection and ejaculation, and to improve overall strength and cardiovascular fitness. FES is used in conjunction with other therapeutic modalities.
Surgical reconstruction of the upper extremity in tetraplegia by means of tendon transfer, tenodesis, contracture release, and other techniques can offer some functional restoration of the limb. These procedures alter the mechanics of the extremity, allowing better participation in activities such as self-care, transfers, and wheelchair propulsion.
Outside of the surgical realm, assistive devices can greatly enhance patients’ ability to perform ADLs. There are several categories of such assistive devices, including those that aid in feeding, dressing, hygiene and grooming, and communication. For example, a universal cuff with a utensil holder allows patients with elbow flexion control the ability to self-feed. Tenodesis orthoses facilitate prehension in individuals with wrist extension control. Items such as toothbrushes and writing tools may be built up to allow for better grasp.
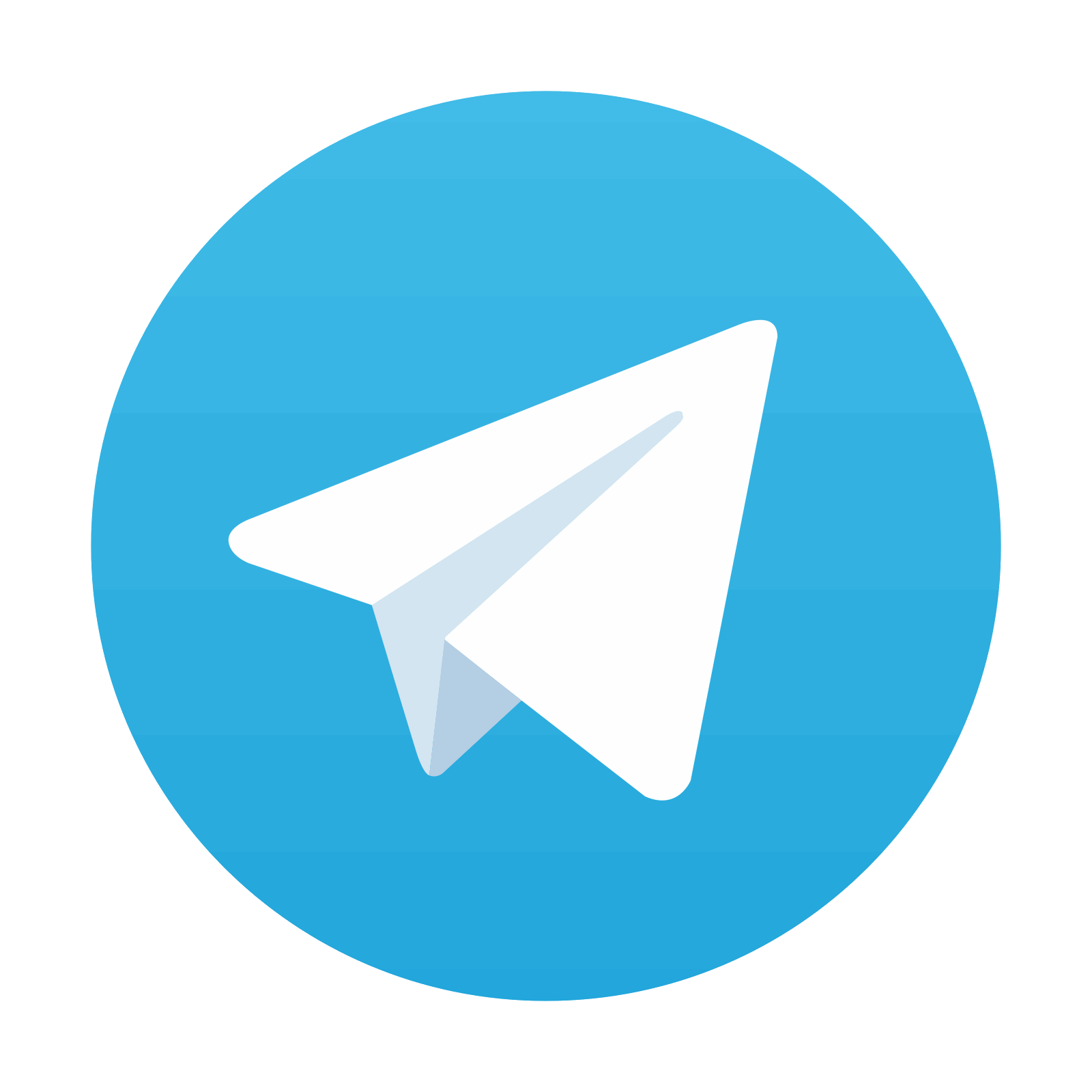
Stay updated, free articles. Join our Telegram channel

Full access? Get Clinical Tree
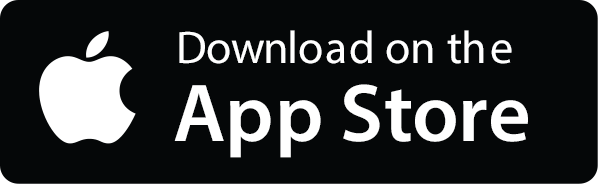
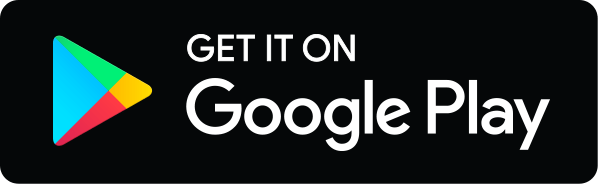