CHAPTER 3
Spasticity and Other Signs of the Upper Motor Neuron Syndrome
Nathaniel H. Mayer
The noted 19th-century neurologist John Hughlings Jackson was one of the first to recognize that a lesion of the central nervous system could simultaneously result in the development of positive and negative signs, although he did not believe that the lesion directly caused the observed signs. In humans, a lesion of the descending corticospinal motor system is capable of producing the negative sign of muscle weakness during voluntary effort and, at the same time and in the same muscle, the positive sign of increased resistance to passive stretch. The combination is the key feature of muscle spasticity, although it is important to recognize that spasticity is only one of a number of positive signs that materialize after an upper motor neuron (UMN) lesion. The aggregate of positive and negative signs after a UMN lesion comprises the upper motor neuron syndrome (UMNS).
Jackson conceptualized the central nervous system as a hierarchical system operating across a number of levels. He believed that negative signs represented dissolution of the highest, most flexible, most volitional level of neural function, whereas positive signs resulted from less flexible, more stereotypic levels of neural circuitry that became excessively excited or released from higher level inhibitory controls (1). Negative signs reflected a loss of a particular capacity ordinarily controlled by the lesioned area of the brain, whereas positive signs reflected release phenomena, abnormal or exaggerated behaviors that were stereotypic in nature and potentially explained by withdrawal of inhibition from neural tissue normally mediating that behavior. A loss of inhibition resulted in positive signs, manifested clinically by stereotypic movements and postures that were generated by stereotypic linkages of overactive muscle groups. Positive and negative signs interact often at the same time.
Inequalities of muscle weakness and muscle overactivity within and across muscle groups often lead to a net balance of muscle torques acting across joints shared in common by these groups. For example, a positive behavior such as an associated reaction may promote involuntary elbow and finger flexion in a hemiplegic patient. Because the lesion in this example also impairs voluntary elbow and finger extension, the patient is unable to actively extend the elbow and fingers to reverse the flexed joint attitude produced by the associated reaction. Based on clinical experience, passive stretching exercises do not fend off the persistence of UMNS postural patterns. In time, this combination of unidirectional, unreversed flexion of elbow and fingers leads to stereotyped flexion postures in our patient. Thus, the stereotypy inherent in UMN release phenomena gives rise to movements and postures familiar to clinicians (eg, flexed elbow, clenched fist, equinovarus foot) (2). These movement patterns and postures, generated by various positive signs and unreversed by negative signs, often have detrimental consequences for patient care and require treatment. For example, persistent posturing often creates overlapping skin folds, underneath which maceration, erythema, and malodor flourish. Fixed, excessive postures can cause stretch injury of nerves. Fingernails can dig into the palm, causing pressure, pain, and laceration. Moreover, clinical experience reveals that UMN patterns are more diverse than perhaps commonly noted. For example, a flexed elbow is very common, but an extended elbow is also found. A flexed wrist is common, but an extended wrist is also seen. No matter the diversity of pattern, it still reflects an underlying net balance of torques brought on by positive sign stereotypy and deficient voluntary reversal of negative signs.
In summary, a lesion of the descending motor system according to Jackson’s hierarchical notion produced higher-level neural dysfunction or frank absence of voluntary movement (negative signs) and simultaneous (although not necessarily instantaneous) stereotypic behaviors (positive signs) that reflect released lower-level, premorbidly inhibited behaviors of an involuntary nature. In a sense, the influence of a UMN lesion, variable in its severity, can cause any given muscle to behave variably in two ways: a negative way (loss of volitional command) and a positive way (acquisition of involuntary behaviors). As a result, the interaction between positive and negative signs, the aggregate effect of a UMN lesion on all muscles surrounding a joint, makes the analysis of joint movement and limb function complicated. What follows is a clinical description of the features of the UMNS with respect to negative and positive signs. For the sake of brevity, this chapter focuses primarily on upper limb examples.
NEGATIVE SIGNS
Impairment of Meaningful Action
With patients commonly complaining of weakness, loss of strength is considered the premier negative sign of UMNS, and voluntary strength testing can be significantly reduced. Studies at the physiological level have shown that reduced motor unit recruitment and frequency contribute importantly to the clinical effect of weakness (3). Against increasing loads, the orderly recruitment of small to larger units may also be impaired (4). Nevertheless, many patients retain considerable strength, especially if tested isometrically. In some cases, it is striking how much forward push a patient can develop against an examiner’s hand isometrically while being unable to reach forward even an inch isotonically. This observation raises questions regarding the value and validity of the testing of muscle strength in this population. On further reflection, weakness does not seem to be the best description of impaired volition in UMNS. Two features stand out. The first is a loss of selective control, that is, a loss of independent joint movement and inability to perform separate movements of individual joints or to operate and control several joints selectively as a unit at will. Instead of autonomous control, the patient with UMNS, attempting to make selective joint movement, makes multijoint obligatory patterns of movement that reflect obligatory muscle activations within and across joints. A patient of mine with UMNS after 7 years following a head injury reported to me the recovery of selective control of thumb, index, and long fingers. When he sat down at the piano one morning, he was able to strike keys separately with his thumb, index, and long fingers. However, when he attempted to selectively strike a key with the ring or little fingers, they coupled together and two keys were struck. He was unable to operate the ring and little fingers selectively no matter how much voluntary effort was made.
The commonest example of obligatory linkages across jointed limb segments during a movement effort is the so-called flexor synergy pattern (Figure 3.1): scapular retraction, shoulder abduction and external rotation, elbow flexion, and forearm supination. When asked to perform a task such as reaching forward to grasp an object (Figure 3.2), the patient dominated by flexor synergy reveals many, if not all, of these joint movements. When asked to perform a totally different task (Figure 3.3), the flexion “synergy-bound” patient produces a similar stereotyped behavior. The patient is locked into an obligatory movement pattern that is initiated (and terminated) by volitional effort. It is beyond the scope of this chapter to discuss the nature of voluntary movement, but for now, we note that a number of voluntary features are retained by a patient with UMNS during synergy production, including initiation, termination, and varying the speed of synergy pattern movement. The key characteristic of an obligatory flexor or extensor synergy pattern is that it is meaningless with respect to the task at hand. On seeing repeated flexion synergy patterns of movement, for example, a remote observer would become puzzled about the patient’s intended actions because the movement pattern would be very similar across differently intended tasks. No intent can be discerned, no outcome of the movement effort makes sense, and analogous to aphasia in the language system, the obligatory flexion synergy pattern seems to produce an inscrutable “aphasic” response or meaningless behavior. The stereotypic behavior of a flexor synergy pattern is without apparent meaning from the point of view of intentional action. The patient dominated by flexor synergy pattern seems unable to generate an appropriate set of task-related instructions for transmission to muscles of the upper limb in order to produce meaningful motor behaviors that reflect different intentions. Extrapolating from Jackson’s hierarchical model, high-level neural entities that assemble and organize the movements comprising an action task are seemingly absent in the synergy-bound patient. Lacking higher level, task-related neural function—a negative consequence of UMNS—what becomes manifest clinically with volitional effort is released positive lower level neural activity, neural circuits with fixed muscle linkages across limb segments, resulting clinically in the stereotypic behavior of obligatory synergy patterns. The behavior starts and stops by volitional effort, but its content is not meaningfully related to an intention-driven task, its instructional set is empty, and the resulting stereotypic behavior ends up as a kind of released default pattern. In the synergy-bound patient, an attempt to produce meaningful action ends up initiating the same repetitive default pattern, time after time. Chronic unidirectional joint movements with little, if any, redirection or reversal of joint movement are a source of recognizable UMNS patterns, generated by persistence of stereotypic, positive or release behaviors resulting from higher level dissolution of nervous control, Jackson’s negative sign.
FIGURE 3.1 Flexor synergy pattern. Note: Shoulder (scapular) retraction, relative shoulder abduction and external rotation, elbow flexion, and forearm supination.
FIGURE 3.2 Effort by a hemiparetic patient to reach forward and grasp a door handle does not produce the intended movement pattern but only components of flexor synergy (shoulder retraction, abduction, and elbow flexion).
FIGURE 3.3 Effort by the same hemiparetic patient to touch her chin, a totally different task from reaching forward to grasp a door handle, produces a similar meaningless stereotypy of movement as in Figure 3.2.
STRETCH-SENSITIVE POSITIVE SIGNS
Stretch Reflexes
Spasticity is a term linked to sensitivity of a muscle to stretch. Clinically, stretch sensitivity results in exaggerated stretch reflexes. Spasticity as a phenomenon has a specific definition, but it is often used (confusingly) as a collective term for all positive signs of UMNS, many of which are not based on sensitivity of a muscle to stretch. Strictly speaking, spasticity is a clinical behavior based on increased excitability of phasic and tonic stretch reflexes that are present in many patients with a UMN lesion. In normal individuals, passive stretch, including rapid passive stretch of a muscle group, does not produce noteworthy resistance. Electromyographically, little, if any, activity is generated (Figure 3.4), and when present, the electromyographic (EMG) activity is brief, reminiscent of a tendon jerk response, and typically produced only by the most rapid of stretches.
In a spastic patient, however, once the threshold of the EMG activity is triggered at a given degree of muscle stretch, the EMG activity persists until stretch is relinquished (Figure 3.5). The examiner experiences increased resistance shortly after threshold activity begins, and resistance persists and usually continues to increase until stretch is released. Starting from a position of maximal muscle shortening and “rest” (ie, the patient eschews voluntary effort), the defining characteristic of spasticity as experienced by an examiner doing the stretching is a velocity sensitive increase in resistance. Velocity sensitivity means the following: the examiner performs several trials of stretch at different rates of stretch for each trial. The target muscle group is stretched through the full (available) range of joint motion at different rates of stretch, for example, slow, moderate, fast, and very fast rates of limb segment motion. If the patient is spastic, resistance to stretch will be felt by the examiner beginning at some joint angle during the stretch maneuver, and the intensity of resistance will increase the faster the examiner stretches the muscle group in each subsequent trial. An increased tendon jerk reflex is also considered a manifestation of muscle stretch sensitivity and is discussed as follows.
FIGURE 3.4 In normal individuals, passive stretch generates little, if any, EMG activity. When EMG is present, the activity is of short duration, reminiscent of a tendon jerk response, and typically only produced by the most rapid of stretches.
EMG, electromyography.
FIGURE 3.5 This patient with UMNS shows different EMG thresholds (ie, onset of EMG activity) at different wrist angles and different amounts of EMG activity as a function of the initial velocity of stretch (slowest rate in the left record, fastest rate in the middle record).
EMG, electromyographic; UMNS, upper motor neuron syndrome.
The character of the stretch reflex was first identified by Sherrington’s seminal studies of the cat’s myotatic reflex (5,6). Before his studies, clinicians were well aware of tendon jerk responses, but they thought the jerk phenomenon was a local response generated by muscle. Sherrington’s studies demonstrated that stretch responses could be abolished by cutting the relevant dorsal roots. By doing so, he established the afferent-efferent nature of the stretch reflex, a circuit that required transmission through the central nervous system, and he established a basis for understanding later descriptions of clinical reflex phenomena. One of my favorites is the succinct description of the clinical characteristics of spastic stretch reflexes by Peter Nathan (7): “Spasticity is a condition in which stretch reflexes that are normally latent become obvious. In spasticity, the tendon reflexes have a lowered threshold to tap, the response of the tapped muscles is increased, and additional muscles besides the tapped one respond; tonic stretch reflexes are affected in the same way” (7).
Spasticity and muscle tone are often confused. Tonus or tone of a system generally refers to baseline physiological activity of a system. For example, normal bowel sounds reflect baseline peristalsis in the intestinal tract. Historically, muscle tonus, like baseline activity in other bodily systems, was originally thought to be an active phenomenon, the result of a small degree of physiological muscle contraction occurring “at rest.” When electromyography became available, no EMG activity was found in normal muscle at rest, and baseline muscle tone could not be explained as an active contraction phenomenon. It was then realized that normal muscle tone was more likely a product of its inherent rheological (elastic, viscous, and plastic) properties. As described earlier, passive stretch of normal (in vivo) muscle tissue produces little, if any, EMG response and normal muscle shows little stretch sensitivity (Figure 3.4). The tone or resistance felt by an examiner when passively stretching normal muscle is rheological (related to the physical properties of muscle tissue) and not dynamic or actively contractile. The problem arises when a muscle develops stretch sensitivity after a corticospinal system lesion. Passive stretch of a stretch-sensitive muscle results in dynamic contraction generated by the stretch reflex. In theory, spastic muscle at rest (if there is no associated increased tone) is silent (a finding demonstrated as early as 1941 by Hoefer and Putnam [8]), but a spastic muscle develops active contraction after stretching has begun and stretch reflex activity has been elicited. Muscle tonus, that is, baseline tone of muscle as an organ (like baseline peristalsis of the intestinal tract) cannot be measured by stretching because a stretched UMNS muscle is no longer at baseline, with reflex tension typically developing when it is stretched. Logically, baseline tone of a spastic muscle needs to be measured before it is stretched to avoid generating stretch reflex tension. We note that nerve blocks can eliminate reflex contraction (a variation on Sherrington’s “dorsal root” strategy) and reveal the state of muscle tone freed from reflex contraction. Many studies have shown a change in rheological properties of the UMNS muscle and have found that noncontractile hypertonia can be considerable (9). Nevertheless, clinicians seem to ignore this contradiction and prefer to equate muscle tone with muscle sensitivity to passive stretch, that is, the degree of resistance elicited by an examiner during passive muscle stretch, often sorting patients into “high tone” and “low tone” categories. If one prefers to use resistance to passive stretch as a measure of tone, it is important to realize that it has neural and nonneural components. The neural component produces stretch reflex activity. The nonneural component comes from rheological or physical properties intrinsic to muscle and other soft tissues. Patients early in recovery may start with increased muscle tone on a neural (spastic) basis, only to develop advanced stiffness and contracture later, resulting in a similar finding of increased muscle resistance that now has a nonneural basis (10). Such nonneural stiffness may be associated with reduced tonic stretch reflex and tendon jerk activity. Under circumstances of advanced stiffness and contracture, separating neural from nonneural contributions to muscle tone may be very difficult at the bedside. This distinction is not merely academic because treatment of muscle overactivity by drugs such as botulinum toxin and dantrolene sodium depends on mechanisms that block muscle contraction, not mechanisms that mitigate rheological properties. In particular, the presence of contracture (fixed shortening of soft tissues) reflects an end-stage condition for the nonneural component and typically requires physical strategies such as serial casting or surgery.
Returning to Peter Nathan’s description of stretch reflexes, he refers to an increase in phasic stretch reflexes (tendon jerks) and tonic stretch reflexes (progressive passive stretch). Generally, the term phasic implies time varying, whereas tonic is relatively time invariant. Neilson (11) identifies the tonic stretch reflex as a reflex whose response can be described as a linear transformation of the stimulus waveform (progressive passive stretch). On the other hand, the reflex mechanism of a tendon jerk is one that generates a triggered response, having little or no relationship to the mechanical waveform of the tendon strike. Latash (12) identifies the term phasic stretch reflex with the response to a change in the level of a stimulus specific to muscle stretch receptors. When an examiner performs a tendon tap, the joint angle and, hence, muscle length are held at a steady level of tautness (ie, stretch) before the tendon strike. A sudden tap of the taut tendon produces a sudden change in muscle length, resulting in a jerk response. Phasic behavior usually represents a brief excitation of muscle that leads to a twitchy movement, a tendon jerk being one example. In this respect, all monosynaptic reflexes are phasic. Tonic stretch reflexes, on the other hand, respond to the level of a stimulus and lead to sustained muscle contraction for the duration of the stimulus. For example, passive stretch of a muscle, that is, progressive lengthening of the muscle, generates a change in the level of the stimulus (muscle length), and in spastic patients, once the threshold of excitation is reached, sustained reflex contraction is produced until stretch is relinquished. Some authors use “phasic” and “tonic” to describe the nature of the input stimulus, whereas others describe the output response of the system as either “phasic” or “tonic.” Lance (13) characterized spasticity as an increase in velocity-dependent tonic stretch reflexes with exaggerated tendon jerks. In Lance’s consensus definition, “tonic” stretch reflexes referred to the output response of a muscle group that was stretched at different velocities. In our view, the two bedside ways of assessing phasic and tonic stretch reflexes are tendon taps and passive muscle lengthening at different rates of stretch, respectively. From a Jacksonian perspective, phasic and tonic stretch reflexes are stereotypic positive signs. Patients with a stable UMNS have phasic and tonic stretch reflexes that do not vary much from day to day at the bedside.
Phasic Stretch Reflexes and Clonus
A phasic jerk response is produced by briskly tapping a tendon held taut by the examiner. In this way, stretch of the tendon-muscle system occurs virtually at the instant of tap. Stretch of extrafusal muscle fibers is detected by the muscle spindle and transmitted to the central nervous system by Ia afferents that project through the dorsal roots and make a number of connections in the spinal cord. These include monosynaptic excitatory connections with homonymous alpha motor neurons that innervate the tapped muscle group and from which afferent outflow originates, along with monosynaptic, excitatory connections to heteronymous synergists. The clinical observation of reflex radiation to muscles other than the one whose tendon has been tapped is explained by Burke (14) as the result of muscle spindles excited by spreading vibrations originating from the site of tap. Monosynaptic connections are also made to the Ia inhibitory interneuron that projects to alpha motor neurons of antagonist muscles. Consequently, when a muscle is stretched, motor neurons innervating antagonist muscles are inhibited. The pattern of simultaneous inhibition of antagonists and excitation of homonymous and heteronymous motor neurons underlies the mechanism of reciprocal inhibition. Interneuronal circuitry, therefore, plays an important role in regulating segmental spinal reflex activity. After a UMN lesion, a net loss of inhibition impairs descending control over motor neurons. A loss of inhibitory control over interneuronal pathways in the spinal cord also occurs. As a result, there is enhancement of the central excitatory state, and tonic and phasic stretch reflexes become manifest clinically.
Clonus is a low-frequency rhythmic oscillation observed in one or more limb segments. It may be generated by rapid stretch and hold of a muscle group. It may also be patient generated when a muscle group is stretched during limb positioning or passive exercise. Clonus may also be triggered during voluntary movement, for example, during a reaching effort when voluntary elbow extension triggers clonus in elbow flexors. Electrophysiologically, clonus represents short-duration electrical activity of involved muscles that occur at typical frequencies of 6 to 8 Hz (see Figure 3.6). Figure 3.6 shows forearm pronator clonus in a patient with stroke and left hemiparesis of 4 years’ duration. Clonic bursts of EMG develop in both pronators at a rate of about 8 Hz. Clonus can be sustained or unsustained, and it can be stopped by repositioning clonic muscles to a shorter length. Clonus is usually associated with other hyperexcitable phasic stretch reflexes. In addition to rapid stretch, various cutaneous stimuli, especially cold or noxious stimulation, may give rise to ipsilateral or even contralateral clonus (15). Clonus may represent self reexcitation of stretch reflexes in a hyperexcitable stretch reflex loop (16). Rack et al (17) viewed clonus as a self-sustaining oscillation of the stretch reflex pathway, with the frequency of clonus being determined by physical parameters rather than central mechanisms. On the other hand, others felt that a central oscillator was operating (18,19).
FIGURE 3.6 Clonus at a frequency of 7 Hz developed in both pronators during voluntary underhand reaching performed by a patient with UMNS.
UMNS, upper motor neuron syndrome.
Tonic Stretch Reflexes
The defining characteristic of clinical spasticity is excessive resistance of muscle to passive stretch, a resistance that intensifies as the examiner increases the rate of stretch in subsequent stretch maneuvers. Slow stretch may offer modest resistance, but fast stretch can result in a suddenly intensified resistance that may even catch the examiner off guard. Increased EMG activity of the stretched muscle accompanies different clinical rates of stretch (Figure 3.7). Contractile tension generated by the stretch reflex opposes the examiner’s act of stretching. If reflex tension is high, it can decelerate the examiner’s rate of stretch so that a constant “velocity of stretch” cannot be maintained by the examiner. Studies that use machines to apply a constant velocity of stretch do not simulate what clinicians experience because clinicians, unlike machines, cannot maintain a constant stretch velocity when spastic tension runs high. Figure 3.8 illustrates spasticity of wrist flexors in an adult with spinal cord injury of 2 years’ duration. The EMG responses to stretch of the wrist flexors at two different rates of stretch are displayed. Note an increase of EMG in the faster stretch record on the right. Differential muscle responses to stretch can occur within a group as illustrated in Figure 3.9 and likely signifies a lack of homogeneity in the central lesion or in its reorganization. When using focal treatments delivered to individual muscles, it is important to identify whether all or only some muscles of a group are spastic. When a spastic muscle is stretched, reflex activity, once triggered, commonly continues until the examiner stops stretching and immediately releases the muscle group. In some patients, reflex activity persists if the examiner continues to hold the muscle statically in a stretched state after dynamic stretch has ended. Such activity has been referred to as a static stretch reflex. The static stretch reflex has been attributed to secondary muscle spindle endings; primary endings of the muscle spindle are known to have dynamic or velocity sensitivity.
FIGURE 3.7 Using root mean square EMG activity as a quantitative measure, an increase in root mean square EMG activity is observed with increasing rates of stretch, from slow to very fast stretch, applied by a clinical examiner to a patient with spastic wrist flexors.
EMG, electromyography.
It remains unclear what operational mechanisms underlie exaggerated stretch reflex activity in the UMNS. Proprioceptive afferent activity generated by muscle stretch is not increased above baseline, and so far, evidence for the theory of increased fusimotor drive has been lacking (20). Evidence seems to favor a reduction in the threshold of the tonic stretch reflex, namely, less afferent input is necessary to trigger stretch reflex activity in the presence of a UMN lesion.
FIGURE 3.8 Passive stretch of wrist flexors in a patient with spinal cord injury. Note that a constant velocity of stretch is not maintained by the examiner. As the amplitude of stretch increases, stretch reflex activity superimposed on the tension length curve of muscle creates increasing resistance that counters the examiner’s stretching force, causing the rate of stretch to slow down.
FIGURE 3.9 Differential muscle responses can occur within a muscle group as illustrated in this record of passive stretch of elbow flexors in a patient with clinical spasticity. Note considerable stretch-induced activity in brachioradialis with minimal responses in brachialis and pronator teres and no response in biceps. When using focal treatments for spasticity, it would be important to identify which muscles in a group are actually spastic.
Increased stretch reflex activity resulting from increased gain in the system, that is, more output reflex activity for the same amount of afferent input (“more bang for the buck”) is a less favored view. Mechanisms of reduced presynaptic and postsynaptic inhibition and impaired recurrent inhibition in the Renshaw system are likely to contribute to the handling of afferent input by a reorganized spinal cord (21). Herman (22) expressed the view that enhanced reciprocal inhibition is characteristic of spastic hemiplegia, whereas reduced reciprocal inhibition is more characteristic of spastic paraplegia.
Stretch-Sensitive Cocontraction
Cocontraction in the UMNS is seen during voluntary effort. Cocontraction is the simultaneous activation of agonist and antagonist muscles. The key feature of cocontraction is that it occurs during voluntary effort and that it is generated by simultaneous supraspinal motor drive to agonist and antagonists (Figure 3.10) (23,24).
Physiologically, Humphrey and Reed (25) found cocontraction to be activated and deactivated at a cortical level. Although cocontraction can be a normal mechanism to provide joint stability under particular circumstances, cocontraction in the UMNS refers to inappropriate antagonist activation that blunts or even reverses agonist-driven movement. Cocontraction, because it originates supraspinally, may occur during isometric effort when antagonist muscle stretch does not occur (26).
FIGURE 3.10 Activity at the onset of movement is seen in both pronator muscles in this record of a voluntary effort to supinate the forearm, made by a patient with UMNS. Note how pronator cocontraction eventually limits, even reverses, forearm movement.
UMNS, upper motor neuron syndrome.
However, antagonist muscles are being stretched during isotonic movements driven by agonists. Therefore, stretch-sensitive antagonist muscles may be subject to stretch reflex activity superimposed on the supraspinal drive of cocontraction, and hence, the term spastic cocontraction has been applied to such antagonist activity. Essentially, the antagonists have two sources of efferent input: supraspinal motor drive and segmental stretch-driven reflex activity. It is difficult to make practical clinical distinction between supraspinal and segmental stretch reflex drives going to an antagonist muscle. Such a distinction is not vital to medications with a peripheral mechanism of action, but theoretically, drugs that have relevant central mechanisms of action could have a differentiating effect. Clinically, patients with spastic cocontraction often move with slowness and with great effort. Problems arise when patients experience a restraining or braking action produced by antagonist cocontraction during voluntary isotonic movements. When cocontraction is present, the performance of alternating voluntary movements about a joint will reveal temporal asymmetry. For example, during alternating flexion and extension movements around the elbow, elbow flexion is typically quicker, whereas elbow extension is typically slower because flexors cocontract during extension phase. The cocontracting elbow flexors restrain elbow extension, causing temporal asymmetry. The amplitude of elbow extension may also be altered.
Stretch-Sensitive Dystonia
Denny-Brown (27) used the term dystonia to describe a variety of postural reactions developed by monkeys after ablations of the cerebral cortex were performed. Lesions were independent of, or in addition to, damage to the pyramidal tract. Holding the monkeys in different spatial positions led to a variety of different postural reactions of the limbs, and these limb postures remained persistent in their attitude. Any attempt by an examiner to pull a limb away from its persistent position was met by an increased resistance of springy quality. The limb would fly back to its original posture when released. Denny-Brown called this fixed attitude dystonia, a term signifying a persistent posture maintained by muscular contraction. He revealed the active nature of dystonia in these monkeys by demonstrating continuous EMG activity in relevant limb muscles. In addition, he showed the dystonia to be efferent. It did not depend on afferent input from the limb because it persisted even after the dorsal roots were cut.
Like Jackson’s thinking regarding the effects of brain lesions, Denny-Brown thought that dystonia after cortical ablations represented released motor behavior generated by parts of the motor mechanism that had direct access to alpha motor neurons (28). Of interest in the present context, he pointed out that monkeys who underwent various cortical ablations did not have spastic features, such as tendon jerk hyperreflexia. Efferent drive at rest was supraspinal in origin and seemingly was unrelated to afferent-efferent stretch reflex arcs. However, Denny-Brown also indicated that the dystonia of his monkeys was affected by the degree of stretch placed on a muscle, and in humans with UMNS, dystonic (at rest) activity in a muscle can be modified by prolonged stretch (29,30). The presence of muscle activity at rest (ie, without obvious source of afferent input, phasic stretch, or volitional effort), sensitive to prolonged (tonic) stretch, has been called spastic dystonia. For example, Figures 3.11 and 3.12 illustrate a patient with right hemiparesis secondary to a stroke sitting quietly at rest.
Persistent EMG activity was recorded from the pectoralis major, and a lesser level of activity is also noted in the long head of triceps. The clinical posture of an adducted/internally rotated shoulder is noted in the figure. The shoulder adductors were spastic on passive stretch. Range of motion exercises with sustained stretching eased her clinical complaint of shoulder stiffness, but passive stretching had to be repeated frequently. Dystonia “sensitive to stretch” can result in a lessening of the dystonic activity as a result of sustained stretch. Phasic or brief duration stretch can elicit a spastic (resistive) reaction. Some are of the opinion that delayed relaxation after voluntary contraction characterized by continuous firing of motor units is also a form of spastic dystonia (31).
FIGURE 3.11 Note an adducted, internally rotated shoulder at rest in this hemiparetic patient due to stroke.
FIGURE 3.12 At rest, persistent dystonic EMG activity was recorded from the pectoralis major, and a lesser level of activity was also present in the long head of triceps. The patient was asked to sit quietly and relax. The pectoralis major is an adductor and internal rotator of the shoulder (correlate with Figure 3.11).
EMG, electromyographic.
If available, EMG equipment can help identify persistent muscle activity that is potentially consistent with the dystonic form of muscle overactivity. However, without using this confirmation method, it may be risky to assume that all patients with persistent limb postures have underlying muscle activity that sustains the posture. Passive tissue stiffness alone may be sufficient to hold a posture. Stretching a muscle group may elicit a spastic response that brings the stretched muscle back to its equilibrium position at which it will remain in equilibrium, balanced by the passive rheological properties of muscles and other soft tissues surrounding the joint. Muscle contracture, that is, fixed shortening, holds a limb in a fixed posture as does heterotopic ossification. Activity generated by an unnoticed associated reaction could mimic dystonic posturing, for example, standing “quietly at rest” while actually weightbearing on a cane with the contralateral limb could promote ipsilateral elbow flexor activity and a flexed elbow posture. Associated reactions in UMNS are described in the next section. Their essential feature is that voluntary activity in one part of the body accompanies involuntary activity in another.
POSITIVE SIGNS THAT ARE NOT STRETCH SENSITIVE
Flexor and Extensor Spasms
A characteristic feature of the UMNS, according to Lance (32), is the release of flexor reflex afferent activity. The flexor reflex is a polysynaptic reflex that results in contraction of flexor muscles across several limb segments. It is generated by afferent stimuli collectively known as flexor reflex afferents. These afferents may include cutaneous receptors responding to touch, temperature, and pressure, and nociceptors responding to painful stimuli, secondary endings from muscle spindles (group II afferents), and free nerve endings scattered diffusely in skeletal muscles. The polysynaptic flexor reflex has a long latency (twice the latency of a monosynaptic tendon jerk) due to slow afferent conduction into the cord and to central delay. Flexor reflex afferent activity ascends and descends in the cord, synapsing in the internuncial pool, a system of spinal interneurons influenced by inputs from peripheral as well as central sources. Compared with segmental stretch reflexes, the time course of polysynaptic flexor reflexes is slower, and unlike segmental stretch reflexes, flexor reflexes represent coordinated activity of groups of motor neurons spanning many segments. Polysynaptic reflexes result in muscle contraction across multiple joints, sometimes bilaterally. Recruitment of flexor muscles across a number of joints is an example of an interjoint reflex that can protect tissues subject to noxious stimulation. Extensor reflexes are also polysynaptic and may contribute to body support functions. Flexor and extensor reflexes may be the substrate for more complex coordinative patterns, such as locomotor stepping generators.
After a UMN lesion, particularly after a spinal cord lesion, disinhibition of the flexor reflex becomes more prominent, clinically. Flexor reflexes can range from the familiar Babinski sign to a mass triple flexion reflex involving the hip, knee, and ankle. A patient may call the flexor reflex a muscle “spasm,” but a careful history will reveal that the patient refers to multijoint activity rather than focal spasm of a single muscle group crossing one joint. Flexor reflex afferents from limbs and enteroceptors from bladder and bowel can singly or in combination promote polysegmental reflex activity with contraction of muscles around the ankle, knee, hip, abdominals, even paraspinals. A large, sudden rise in intra-abdominal pressure caused by contraction of the rectus abdominis can result in urinary incontinence as part of the phenomenon. In UMN lesions, the onset threshold of the flexor reflex is reduced, contraction intensity is increased, and more muscles and more joints are recruited. Flexor spasms are more common in patients with lesions of the spinal cord than patients with supraspinal hemiplegia. The studies of Herman (33) indicated that the manner in which afferent activity was transmitted through the spinal cord was diffferent for paraplegic and hemiplegic patients.
Associated Reactions
An associated reaction is another form of involuntary muscle overactivity seen in the UMNS. An associated reaction refers to involuntary activity in one limb that is associated with a voluntary movement effort made in another limb. Figure 3.13(A) to (C) shows the sequence of right elbow motion in a right hemiplegic patient moving from a sitting position to a standing position. Body motion including voluntary arm and leg movements on the uninvolved left side was most active during the stand up phase in Figure 3.13(B). Note how an associated reaction of the right elbow flexors developed on the hemiparetic side and produced an increase in right elbow flexion in Figure 3.13(B) compared with elbow flexion during sitting (Figure 3.13A) and during the subsequent standing equilibrium phase (Figure 3.13C). Associated reactions were first described by Walshe in 1923 (34).
FIGURE 3.13 Note changes in elbow angle as the patient moves through the sequence: (A) sitting, (B) standing up, and (C) maintaining a standing position. The photos illustrate an associated reaction of involuntary right elbow flexion during the standing-up phase (B) as voluntary movement efforts in other limbs are taking place.
Following Jackson’s release phenomena formulation, Walsh referred to associated reactions as “released postural reactions deprived of voluntary control.” Associated reactions may be due to disinhibited spread of voluntary motor activity into a limb affected by the UMNS. Figure 3.14 shows a patient with right hemiparesis throwing a ball with the left upper limb. The patient did not involve the right upper limb with the throw, having no volitional control of this limb. Figure 3.14 reveals the presence of EMG activity in the right-sided shoulder muscles associated with the patient’s effort to throw the ball with her left hand. The intensity of an associated reaction in the limb with UMN may depend on how much effort is made by the voluntary limb. Dewald and Rymer (35) thought that impaired descending supraspinal commands were involved in generating an associated reaction. They hypothesized that unaffected bulbospinal motor pathways may have taken over the role of transmitting descending voluntary commands when the other UMN tracts were damaged.
Although clinicians often seem more concerned about spasticity than other positive phenomena of the UMNS, the clinical impact of spasticity may be less than advertised when one ponders how often patients and caregivers might actually stretch spastic muscles at rates that would elicit intense resistance. We have observed patients and caregivers performing limb manipulations at slow rates of stretch to avoid or minimize rate-sensitive spastic resistance. We also note that what often passes for spastic resistance is, in large measure, decreased tissue compliance due to changes in the intrinsic rheological properties of muscle. Associated reactions, on the other hand, may be unavoidable because voluntary movement efforts of a patient with UMNS must necessarily go on throughout the day (36).
FIGURE 3.14 A patient with right hemiparesis throwing a ball with her left hand. The patient did not involve the right upper limb with the throw, having no volitional control of this limb. The figure reveals EMG activity in right-sided shoulder muscles as an associated reaction to the patient’s voluntary ball throw with her left upper limb. Note that these right-sided muscles, pectoralis major, teres major, and latissimus dorsi, are adductors and internal rotators. The patient presented clinically with the UMN pattern of an adducted/internally rotated shoulder.
EMG, electromyographic; UMNS, upper motor neuron syndrome.
High-intensity voluntary efforts required during transfers, gait, and many activities of daily living might be expected to provide ample opportunity for the development of muscle overactivity generated by associated reactions.
Muscle Rheology
Although Jackson conceived of positive and negative signs of the UMNS with respect to nervous system production of motor behavior, others subsequently became cognizant of how changes in peripheral soft tissue could also affect extremity movements. Changes in the rheological properties of soft tissues, especially muscle, are important clinically (37). Muscle contracture, a physical shortening of muscle length, limits the operating range of joint motion and, as such, has a broad influence on the performance of activities of daily living and mobility. Muscle contracture is often accompanied by physical shortening of other soft tissues such as fascia, nerves, blood vessels, and skin. (Muscle contracture, an invariant physical state of fixed tissue shortening, is not to be confused with muscle contraction, a dynamic, variable state of internal shortening produced by the sliding action of actin and myosin filaments within a muscle fiber. Contracture implies that muscle length remains the same even if one were to block all muscle contraction by local or general anesthesia.)
The development of contracture is promoted by a number of processes that start when an acute UMN lesion occurs: (a) paresis impairs cycles of shortening and lengthening of agonist and antagonist muscles caused by everyday muscle use; (b) the force of gravity generates positional effects on limb segments and joints; (c) positional effects are also created by a net balance of static soft-tissue forces traversing joints; and (d) the various involuntary UMN motor behaviors described earlier lead to a net balance of dynamic limb torques acting across joints, resulting in the development of chronic one-way positioning of joints that typically leads to stiffness and contracture. Because muscle relaxant medications affect dynamic muscle contraction only, a clinical picture dominated by contracture will not respond to such drugs. Physical methods are necessary to undo contractures. Distinguishing between dynamic tension of muscle overactivity and static rheological tension can be difficult. O’Dwyer et al (38) suggested that what appears clinically as spasticity after stroke is really increased muscle stiffness and muscle contracture. They suggested that mechanical and biological changes in soft tissues played a major role in resistance to both passive and active movements. Muscles immobilized in a shortened position for long periods became shorter and stiffer. When muscle overactivity developed in these shortened muscles, tension was generated at shorter lengths. A lack of voluntary contraction in the antagonists of these shortened muscles prevented their natural reextension, leading to a continuation of the process of stiffness and fixation. In the upper limb, muscles that typically shorten include shoulder adductors/internal rotators, forearm pronators, and elbow, wrist, and finger flexors. In the lower limb, muscles that typically shorten include ankle plantar flexors, toe flexors, and hip and knee flexors. The position of any given joint results from a net balance of static and dynamic torques of muscles acting across the joint, as well as rheological properties of related soft tissues. Variability in central lesion recovery and reorganization can lead to other types of UMN patterns such as the intrinsic plus hand and the hyperextended wrist.
Based on studies of patients with cerebral palsy (CP), contractures are thought to arise from muscle fibers that have fewer sarcomeres in series and, therefore, are shorter than normal (39). Sarcomere lengths are greater than normal when muscle fibers with fewer sarcomeres in series are stretched during normal movement. These longer sarcomeres are thought to be the main reason that muscles of patients with CP have excessive passive tension. Similar processes might conceivably account for elevated passive tension in adults with UMNS. However, Friden and Lieber (40), reexamining the issue of excessive passive tension in CP, have described the mechanical properties of isolated muscle fiber segments obtained from spastic patients with CP undergoing surgical correction of flexion contractures. They found that single fibers developed passive tension at significantly shorter sarcomere lengths than fibers taken from subjects without spasticity. Muscle fibers of patients were almost twice as stiff as controls, and resting sarcomere lengths were shorter. Friden and Lieber also found that the cross-sectional area of spastic fibers was less than one third of normal fibers. This resulted in greater stress forces when spastic fibers were passively stretched. Their study suggested that sarcomeres in CP do not have to be stretched beyond normal lengths to develop excessive passive tension. From this perspective, their study challenges the assumption that tendon lengthenings or even stretching exercises that aim to allow a muscle to achieve normal sarcomere lengths will lessen passive tension to normal levels. The study of Friden and Lieber points to a process of considerable structural remodeling of spastic muscle tissue components. In that regard, their study challenges the current theoretical framework suggesting that muscle−tendon lengths need to be appropriately adjusted by therapeutic exercise or surgical lengthening. Current theory believes that sarcomeres operating over a more normal range will result in a reduction in passive tension along with better force generation if the muscle−tendon length is adjusted appropriately. The study of Friden and Lieber suggests that this view may have to be altered.
MALADAPTIVE CONSEQUENCES OF THE UMNS
We have offered the argument that the interaction between stereotypic positive and negative signs of the UMNS results in chronic one-way joint attitudes giving rise to common patterns of UMN dysfunction. Mechanical and biological changes in soft tissues, such as muscle, play an important role in resistance to passive and active movements. Generated by the various forms of positive signs, muscle overactivity, superimposed on emerging soft-tissue changes, contributes to a net balance of torques that promotes and maintains tissue shortening and chronic one-way positioning of upper and lower limb joints (Figure 3.15). The negative sign of impaired limb usage and weak voluntary contraction of the antagonists of shortened muscles prevents range of motion in the opposite direction, contributing to the continuation of the process of postural fixation and its maladaptive consequences for patient care. Stereotypic movement patterns and postures, generated by positive signs and not reversed because of negative signs, promote maladaptive UMNS consequences (41) under the following personal health categories exemplified for the upper limb:
FIGURE 3.15 This figure illustrates the concept that UMN postural and movement patterns reflect a net balance of torques generated by combinatorial interactions of various positive and negative UMN signs.
UMN, upper motor neuron.
Skin integrity: Clenched fist (Figure 3.16) and redundant skin folds lead to moisture accumulation, bacterial and fungal overgrowth, malodor, skin irritation, skin breakdown, ulceration, and skin and nail bed infections.
Bone and joint integrity: Shoulder and wrist subluxation, adhesive capsulitis, impingement syndrome, osteoporosis, and joint contracture.
Physical pressure and injury: Thumbnail and fingernails digging into the skin of the palm (Figure 3.17); fist and finger pressure against chest, breast, throat, chin or face; traction on the ulnar nerve in the cubital tunnel by a chronically flexed elbow; pressure on the median nerve in the carpal tunnel by a chronically flexed wrist.
Soft-tissue integrity: Stiffness and contracture of muscle, skin, nerve, and blood vessels.
Personal care integrity: Patients and caregivers having difficulty manipulating limbs encumbered by static and dynamic torques during the delivery of personal hygiene, dressing, grooming, and bathroom care.
Body image integrity: Embarrassing “claw” hand, unsightly flexed elbow, bent wrist, clenched fist, and thumb-in-palm with frequently present malodor.
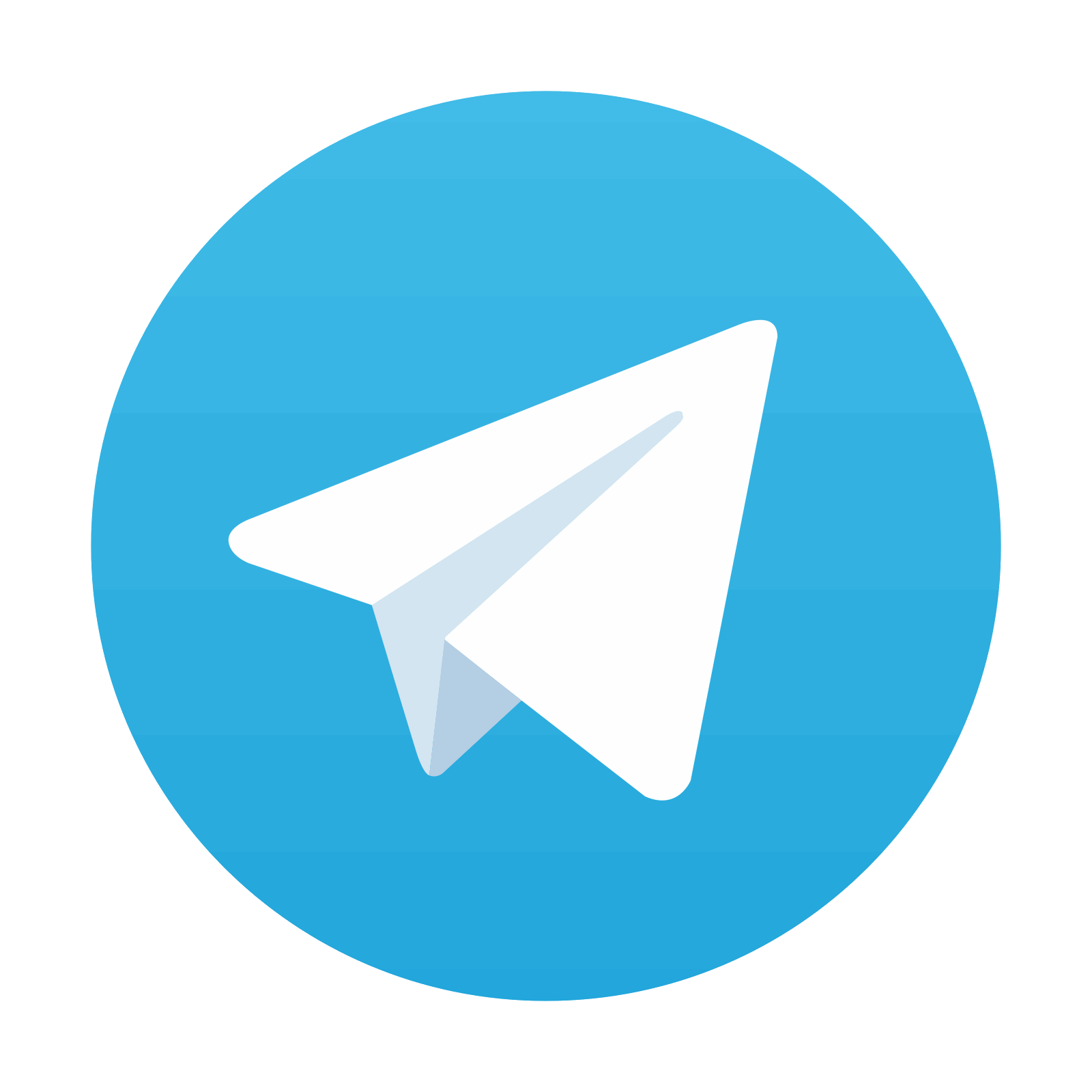
Stay updated, free articles. Join our Telegram channel

Full access? Get Clinical Tree
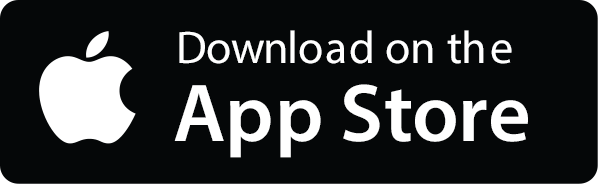
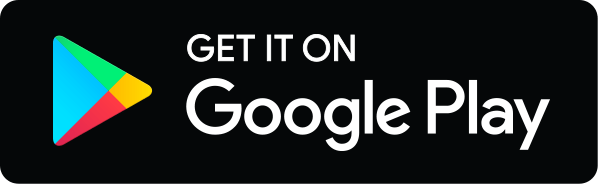