Fig. 1
Lateral (a) and anterior (b) view of a left shoulder. The arrows indicate the subacromial space. * The long head biceps tendon. ** Rotator cuff
Coracoacromial Arc
Acromion
The acromion is flat in shape and extends laterally, then antero-laterally. We distinguish an upper surface, in close contact with the skin, bearing rough scores and vascular orifices; an inferior concavity, which forms the tip of the glenohumeral joint; a lateral margin, the bundles from which the deltoid muscle originates; and a medial margin where the surface of the acromioclavicular joint is.
In the last 30 years, the shape of the acromion has been the object/topic of several studies because it was considered the cause predisposing aliments such as subacromial impingement and rotator cuff tendon tears [1]. In an anatomical study, Bigliani et al. [2] have classified the acromion as types I, II, and III based on the orientation and shape of their lower surface and identified the type likely to cause a reduction in the anatomical space between the acromion and the humeral head. According to this classification, the lower surface of the type I acromion is flat (flat acromion), while in the II and III acromion type it is curvilinear (curved acromion) and hooked (hooked acromion), respectively (Fig. 2a–c). Shoulders with a type III acromion are more prone to have a narrow subacromial space. Other studies have confirmed the correlation between subacromial impingement and rotator cuff tear [3–5].


Fig. 2
(a) Type I (flat) acromion; (b) Type II (curved) acromion; (c) Type III (hooked) acromion
In a study of ours, during which we examined 500 dry scapulae belonging to Caucasians, we evaluated the shape (on the basis of the Bigliani’s classification) [2] and some morphometric features of the acromion. The shape of the acromia was flat in 38.9 %, curvilineous in 39.4 %, and hooked in 21.7 % of the scapulae examined. The percentage of hooked acromia was higher in the scapulae of those aged over 60 (26 %); thus, the hook-shaped acromion is currently considered as being acquired (ossification of the coracoacromial ligament) and not as something that is genetically determined. This observation seems to be confirmed by the studies of Natsis et al. [7] and Schippinger et al. [8]. Other kinds of acromion recently described are the type IV (convex) [9] and the chiglia-like [10]. In an anatomical study, Zuckerman et al. [11] have not been able to identify the three types of acromion described by Bigliani. The authors concluded that the correlation between type of acromion and cuff tear is not clear and further studies are needed to support the role of extrinsic factors in the genesis of cuff tear [11]. Chang et al. [12], after having performed a three-dimensional analysis of the acromion with MRI, came to the conclusion that impingements of any kind caused by the acromion are not the primary cause of cuff tendon rupture.
In our study [6], the average thickness of the acromion was 8.5 mm; in addition, there was a direct linear correlation with the size of the scapula [6]. The acromia of the scapulae as well as the type III acromion belonging to male subjects were significantly thicker than those of females. The torsion angle of the acromion is between 0° and 40° and that of inclination between 20° and 70° [13].
In another study, we conducted on 200 dry scapulae [13], the acromia were distinguished on the basis of Edelson’s classification [14] that differentiates them according to the position of the articular facet with respect to the acromioclavicular joint tip. In 33 % of the acromia, the facet of the acromioclavicular joint was at a distance from the apex of the acromion (type “cobra head acromion”), 22 % were on the apex (type “squared acromion”), and 45 % in an intermediate position (such as “intermediate acromion”) (Fig 3a–c). The average length of the scapular facet of the acromioclavicular joint was 12.7 mm (range: 8–22 mm). Two forms of facet were identified: one to “drop” (31 %) and “elliptical” (69 %). The “drop” type belonged to elderly subjects, and the edge of the veneer often presented degenerative changes. No dependency between the form and the spatial arrangement of the facet was discovered/found.


Fig. 3
(a) Cobra-head acromion; (b) squared acromion; (c) intermediate acromion
The lack of fusion of one or more growth centers located in the apex of the acromion (os acromialis) occurs in about 8 % of scapulae (Fig. 4) [15]. When the unfused core is found at the apex of the acromion, it is defined as preacromion; instead, when it is found more distally, it is named, respectively, mesacromion, metacromion, and basiacromion [16]. The correlation between os acromialis and subacromial impingement is still a matter of discussion [17–19]. A study of ours has shown that the longer the distance between the acromioclavicular joint and the apex of the acromion, the higher the possibility that fusion of the growth nuclei will not occur [20].


Fig. 4
MR scan of a left shoulder. Axial view. The arrow indicates the os acromialis
Baechler and Kim [21] have observed that there is a relationship between the degree of humeral coverage by the lateral margin of the acromion and rupture of the rotator cuff. This association is thought to be due to the friction that is likely to occur during abduction. Nyffeler et al. [22] consider that the sum of the forces brought to bear in flexion/abduction favors humeral proximal migration in cases of greater degrees of acromial coverage. Torrens et al. [23] observed that the prevalence of acromia with higher degrees of humeral coverage was greater in patients with cuff tears than in those belonging to the control group.
Coracoacromial Ligament (CAL)
It is located between the base of the coracoid and the inferomedial surface of the acromion (Fig. 5). It fits on top of the acromion, right in front of the acromioclavicular joint’s surface, and along the entire lateral section of the coracoid. An artery (a branch of the suprascapular) is constantly present on the posterior surface of the ligament. Macroscopically, it presents no homogeneous morphological characteristics.


Fig. 5
Cadaveric right shoulder. The arrow indicates the coracoacromial ligament
An anatomic study revealed that 60 % of the shoulders had a bipartite coracoacromial ligament, a single ligament in 25 % and tripartite in 15 % of the cases [24]. In the latter case, the coracoid insertion of the third band was more medial and may not be visible until a resection of the lateral third of the clavicle is performed. Kesmezacar et al. [25] argued that there are five possible anatomical variants of the CAL (type I: Y-shape, type II: single broadband, type III: quadrangular, type IV: V-shape, and type V: multiband). The Y-shape inserts itself in a unique manner into the acromion. The two bands of the “Y,” which are inserted on the coracoid, are separated by a thin membrane. Of the two bands, one side is thicker and wider. The width of the two insertions of the single broadband variation (type II) is similar [26]. The ligament maintains its width along its entire extension. In type III, the width of the insertion on the coracoid is wider than that of type II. Type IV differs from type I because of the two arms that appear to be separate after the acromial insertion. Even in this case, the side of the higher band is thicker and wider. Type V does not present homogeneous morphological features. Of all the variants, the most common type is the “Y” (41 %), the rarest are types IV and V (both 11 %). For the authors, none of the variants predisposes rupture of the cuff more than the others. However, CALs with the greatest number of bands seem to have a significant association with the degeneration of the cuff. Kopuz et al. [27] conducted an anatomical study on neonatal CALs and observed that the variants at birth are simply three: square, single broadband, and “U.” This observation suggests that the final shape of the ligament is acquired over time. In rare cases, where the pectoralis minor muscle inserts on the capsule of the glenohumeral joint rather than on the coracoid, the tendon passes through the bands of the CAL [28].
In an anatomical study, Fremery et al. [29] observed that shoulders with cuff tears have CAL bands shorter compared to those without tendon rupture and that the CAL changes its morphological and biomechanical features over time. The insertional areas are constituted by fibrocartilage [30]. With aging, the fibrocartilage is also present in the middle portion of the ligament [30].
It was observed [31] that the CAL contains four types of nerve endings: free, Pacinian corpuscles, and Ruffini and Golgi receptors. In addition to these typical endings, other “atypical” ones were observed. All these endings are equally distributed on the surface of the subacromial side of the ligament and in correspondence with the acromial and coracoid insertion [30, 31]. The number of nerve endings decreases in older subjects and in those with subacromial impingement. This observation suggests that in these two categories of persons, the proprioceptive activity of the shoulder is reduced.
With aging, the portion of the ligament that fits onto the acromion may experience ossification (enthesopathy) [30]. The new bone can modify the profile of the antero-inferior acromion, increasing the downward curvature. This explains why the percentage of the hooked acromion increases with aging. In the case of two-part ligament (anterolateral and posteromedial band), spur formations occur predominantly on the anterolateral band [32]. This has led to the hypothesis that of the two bands, the anterolateral is that subjected to greater functional stresses [32]. Ogata and Uhthoff [33] argued that the development of enthesopathy is the result of the transmission of tensile forces within the ligament and that the formation of this spur determines transition from a dysfunctional syndrome to an organic stenosis. Kijima et al. [34] observed that the modulus of elasticity of the CAL of patients with cuff tear is higher than that recorded in the ligaments of subjects without tendon rupture. This shows that physiological tissue degeneration causes progressive rigidity of the ligament. Even Sarkar [35] and Schiavone-Panni [36] observed that tissue disorganization and loss of normal orientation of the collagen fibers is more common in the CAL of patients with subacromial impingement, especially in the deep layer of the ligament.
The CAL is perhaps the only ligament subtended between two transverse processes belonging to the same bone. It has been speculated that this reduces the movement which both the acromion and the coracoid face during the action, respectively, of the deltoid and the conjoint tendons/pectoralis minor [37]. The CAL opposes the upward migration of the humeral head in the case of massive cuff tears [38, 39]. In a study of cadavers, Fagelman et al. [40] have shown that reinsertion of the CAL prevents upper static instability and contributes to refocusing the humeral head inside the coracoacromial arch.
Coracoid
Anteriorly to the glenoid and laterally to the scapular notch, there is an apophysis that due to its shape, like a crow’s beak, was formerly called coracoid (χ o ρ α ξ = chorax = crow).
The coracoid apophysis originates from the anterior-upper extremity of the neck of the scapula and protrudes at first upwards and forward from the side and subsequently arranges itself almost horizontally. The conjoint tendons (short head of the biceps and coracobrachialis) fit onto the anterior apex of the coracoid; further back and laterally onto the coracohumeral and the coracoacromial ligament; medially, onto the tendon of the pectoralis minor muscle (Fig. 6a); and superiorly, onto a rough surface, the coracoclavicular ligaments (conoid and trapezoid) (Fig. 6b).


Fig. 6
(a) Left shoulder of a cadaver. cp coracoid process, pm pectoralis minor, ct conjoint tendons, cal coracoacromial ligament. (b) Right shoulder: cl conoid ligament, tl trapezoid ligament
In a study conducted on 204 dry blades [41], we carried out measurements of the length of the coracoid (L) and the thickness of the coracoid tip (T), the apex of the coracoid prominence over the glenoid plane (cp), the minimum distance between the coracoid tip and the anterior-superior margin of the glenoid (cgd), the distance between the horizontal plane, tangential to the lower edge of the coracoid tip, and the horizontal plane tangential to the cranial glenoid (d). The inclination of the coracoid (cs) (Fig. 7a, b), in the cranial-caudal direction, was measured using Edelson and Taitz’s method [14]. Then, we analyzed the shape of the space delimited by the posterolateral margin of the coracoid and the anterior-superior edge of the glenoid.


Fig. 7
(a, b) Coracoid processes (right samples) with different inclination
The range, the mean, and the standard deviation of L, T, cp, cs, cgd, and d were:
L (mm) | T (mm) | cp (mm) | cgd (mm) | cs (°) | d (mm) | |
---|---|---|---|---|---|---|
Max | 50 | 10.2 | 22 | 22.1 | 42 | 12 |
Min | 31 | 5 | 11 | 11.8 | 19 | 0.5 |
Mean | 38.15 | 7.19 | 14.62 | 16.23 | 25.57 | 7.11 |
SD | 3.97 | 1.04 | 1.96 | 1.7 | 4.71 | 1.23 |
Three types of configuration of the coraco-glenoid space were identified (Fig. 8a–c). In the type I configuration, this space had a “ round parenthesis” shape, while in type II and III, respectively, the shape was that of a “ bracket” and a “hook.” The configuration of type I was observed in 45 % of the shoulder blades and type II and III in 34 % and 21 %, respectively. The minimum coraco-glenoid distance was found in the shoulder blades with a type I configuration. In a study of cadavers, Ferreira Neto et al. [42] observed that in females the distance between the apex of the coracoid and lesser tuberosity is lower than that measured in males. Therefore, women appear to be more likely to develop a syndrome of subcoracoid impingement. Richards et al. [43], availing of MRI scans, measured the coracohumeral distance and observed that patients with a lesion of the subscapularis present a significantly smaller distance than the people in the control group. The possible morphological and morphometric correlation between the coracoid and the subcoracoid impingement was challenged instead by Radas and Pieper [44] who correlate the development of this syndrome to anterior glenohumeral joint instability.


Fig. 8
(a–c). Three types of configuration of the coraco-glenoid space (arrows)
Schulz et al. [45] correlated the position of the apex coracoid to rupture of the rotator cuff. Employing true anteroposterior radiographs, the authors divided coracoids into two classes: those whose apex is projected into the lower half of the glenoid (type I) and those which project their peak into the mid-upper glenoid (type II). The study found that type I coracoids are more frequently found in patients with rupture of the supraspinatus, while those of type II are more frequently observed in patients with injury to the subscapularis tendon.
Humeral Tuberosities and Bicipital Groove
The greater tuberosity represents the posterolateral region of the humeral head (Fig. 9). On it, there are three areas onto which the tendon of the supraspinatus, the infraspinatus, and the teres minor (see rotator cuff) may be fixed. The bone mineral density of the two tuberosities is an important factor in the surgical treatment of cuff lesions. Osteopenia of the greater tuberosity may, in fact, complicate surgical repair of the supra- and infraspinatus and hinder the healing of the two tendons. The tendon of the subscapularis muscle is inserted instead onto the lesser tuberosity that is placed antero-medially. Along with the greater tuberosity, the lesser tuberosity helps to delimit the bicipital groove within which the tendon of the long head of the biceps brachii and the arcuate artery, a branch of the anterior humeral circumflex, slide (Fig. 9). Proximally, it is wide and deep and is lost, gradually, on the front face of the shaft, mingling with the roughness of the bone corresponding to the insertional area of the teres major. Data regarding the morphological and morphometric features of the groove emerging from studies of dry shoulders are mixed. This has been attributed to the extreme variability of the ethno-geographical origin and age of the samples examined (Fig. 10a–c). A radiographic study performed on 200 humeri [46], of which the sex and approximate age were known, showed that the average value of the opening angle (Fig. 11a) of the groove is 102° (extreme 28–160°), while the medial angle (Fig. 11b) is 46° (range 16–78°). The depth and the average width of the groove are, respectively, 4.3 mm and 12.2 mm. Statistically significant differences between the sexes were found only for values regarding the average width (M:13.1 – F:10.2).




Fig. 9
Dry (right) humeral head. gt greater tuberosity, lt lesser tuberosity, bg bicipital groove

Fig. 10
(a–c) Dry humeri. Bicipital grooves with different depth and width

Fig. 11
Radiograms of dry humeri. Opening (a) and medial (b) angle of the bicipital groove.
Rotator Cuff
This consists of the supraspinatus, infraspinatus, teres minor, and subscapularis tendons (Fig. 12). The first three (external rotators) are fixed onto the greater tuberosity, the other (internal rotator) on the lesser tuberosity. At about 15 mm from the insertion on the humerus, the external rotator tendons are seemingly fused together, in particular those of the supra- and infraspinatus. However, if the coracohumeral ligament and connective tissue that overhang the two tendons near their insertion are removed, the front edge of the infraspinatus is more easily highlighted and the boundary between the two muscles becomes more apparent. The front edge of the infraspinatus is slightly more prominent than that of the adjacent rear supraspinatus. This is because the front of the infraspinatus partially covers the portion of the posterolateral supraspinatus.


Fig. 12
Rotator cuff tendons. (a, b) Anterior views. (c, d) Lateral views; (e) posterior view; the scapular spine was removed. sbst subscapularis tendon, sst supraspinatus tendon, ist infraspinatus tendon, tmt teres minor tendon, lhbt long head biceps tendon, ant anterior, post posterior
If the infraspinatus is removed, leaving the capsule below intact, we note that the greater tuberosity is constituted by three distinct areas (the higher, middle, and lower) [47]. Mochizuki et al. [48] observed that the insertion of the infraspinatus occupies about half of the higher and the whole of the middle area. The anteriormost region of the humeral insertion of the infraspinatus almost reaches the anterior margin of the highest part of the greater tuberosity. Because the infraspinatus fits laterally, it can be argued that it can also play an important role in abduction. These new acquisitions concerning insertion of the infraspinatus suggest that the frequent atrophy of the infraspinatus muscle, visible in MRI scans on the occasion of apparent isolated lesions of the supraspinatus, is not attributable to lesions of the suprascapular nerve (due to traction caused by supraspinatus retraction) [49] but to a direct involvement of the infraspinatus itself in the lesion.
Upon removing the supraspinatus, it can be noted that it fits onto the highest antero-medial part of the greater tuberosity.
The footprint of the supraspinatus is triangular in shape, with the longer side toward the articular surface; it is wider at the front and narrower at the back. The supraspinatus also fits onto the lesser tuberosity in 21 % of cases. In these cases, the anteriormost part of the tendon covers the top of the bicipital groove.
Mochizuki et al. [48] measured the maximum length (from medial to lateral) and width (front to rear) of the footprints of the supra- and infraspinatus. That of the supraspinatus is triangular in shape. The maximum length was 6.9 ± 1.4 mm. Instead, the maximum width of the medial margin was 12.6 ± 2.0 mm, while the lateral measured 1.3 ± 1.4 mm. The footprint of the infraspinatus is trapezoidal, wider laterally and medially. Its maximum length was 10.2 ± 1.6 mm. The maximum medial width was 20 ± 6.2 mm; the lateral was 32.7 ± 3.4 mm.
Previous studies assumed that the footprint of the supra- and infraspinatus were longer [50–52] probably because the joint capsule was included in the measurements. The tendon of the supraspinatus is composed of two portions: the anterior half is long and thick, the posterior short and thin. Itoi et al. [53, 54] arbitrarily divided the tendon into three portions (anterior, middle, and posterior) and observed that the anterior third is significantly stronger and tougher than the other two portions. However, the anterior portion, which fits onto the greater tuberosity for an extension corresponding to only 40 % of the tendon insertion, bears proportionately higher mechanical stress, which makes it more vulnerable and predisposed to rupture [55, 56]. In view of these findings, during the repair of the supraspinatus tendon, considerable attention should be paid to reinsertion of the anterior portion.
The so-called critical zone of the supraspinatus tendon is that at about an inch from the insertion of the middle third of the tendon [57]. Nakajima et al. [58] performed a histological and biomechanical study of tendons of the supraspinatus and identified four independent structural subunits. The “real tendon” extends from the myo-tendinous junction to about two inches from the insertion on the greater tuberosity. It consists of parallel collagen dossiers oriented along the stress axis. The “ fibrocartilage” extends from the tendon to the greater tuberosity and is mainly composed of intertwined collagen fibers. The “rotator cable” extends from the coracohumeral ligament to the infraspinatus, lying between the surface layer and the depth of the true tendon. The “capsule” is composed of thin collagenous sheets, each consisting of fibers with the same orientation. The combination of these subunits provides the supraspinatus with dispersive load and compression stress resistance properties [59].
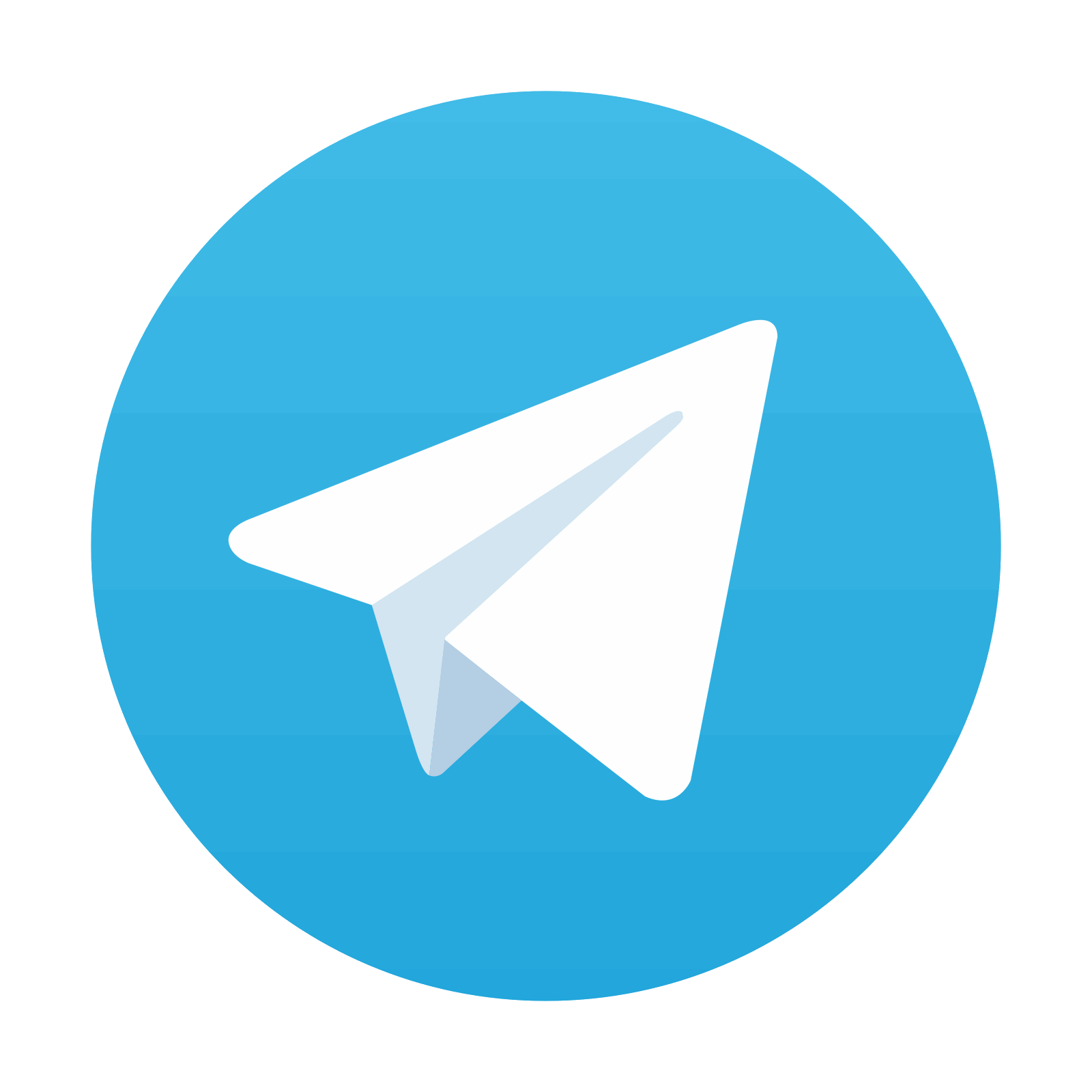
Stay updated, free articles. Join our Telegram channel

Full access? Get Clinical Tree
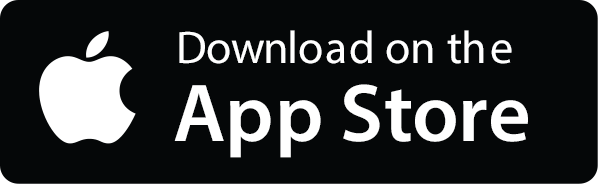
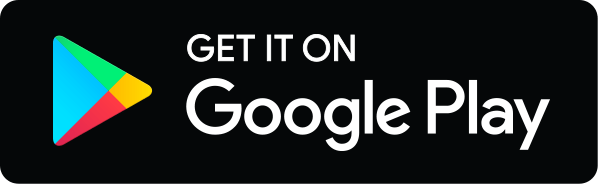