CHAPTER 3 Somatosympathetic reflex mechanisms
Introduction
Healthcare professions whose patient care includes manual therapy, such as chiropractic, osteopathy, and massage, are most commonly recognized for their beneficial effects on the musculoskeletal system. All use some form of manipulation or mobilization of joints, muscles, and other soft tissues. The osteopathic and chiropractic professions in particular have diagnostic algorithms for determining dysfunctional areas of the musculoskeletal system to manipulate or mobilize. These algorithms are remarkably similar. In osteopathic medicine, the acronym TART denotes four signs and symptoms that identify a somatic dysfunction (Kuchera & Kappler 2003): abnormal tissue Texture, positional Asymmetry of hard or soft tissue landmarks, Restricted motion between jointed segments, and tissue Tenderness. Criteria used by chiropractors have been adopted by the Health Care Financing Administration and are embodied in the acronym PART (Sportelli & Tarola 2005): Pain and tenderness, segmental or regional Asymmetry or misalignment, abnormal Range of motion, change in soft tissue Tone. Changes in the biomechanical and chemical environment of the tissues are thought to underlie these signs and symptoms. The practitioner’s goal in manipulating, mobilizing, or massaging these areas is to restore normal motion and normalize the physiology of the neuromusculoskeletal system.
Disturbances in somatic tissues may have consequences that extend beyond the neuromusculoskeletal system and involve visceral function. Many clinicians who practice manual therapy consider the signs and symptoms elicited by TART and PART procedures as representing a combination of altered sensory signaling from the local tissues and altered central neural processing of those signals (Korr 1978, Pickar 2002). All of the chapters in this book demonstrate in some way the biological roles played by the autonomic nervous system, including control of visceral function, homeostatic regulation, promotion of healing, and contributions to the feeling of well-being. Evidence from both clinical experience and experimental studies indicate that mechanical treatment of the musculoskeletal system can indeed provide benefits beyond the neuromusculoskeletal system (Haldeman 1978, Masarsky & Todres-Masarsky 2001).
Stimulation of somatic afferents innervating limbs
Substantial evidence demonstrates that somatic afferents from non-paraspinal tissues can reflexly affect the sympathetic nervous system. Neural recordings from white rami of the sympathetic chain reveal three reflex components of efferent sympathetic discharge in response to electrical stimulation of a peripheral nerve in anesthetized cats and rats (Sato & Schmidt 1971). The A-reflex, so-called because it is elicited by stimulation of Aβ and Aδ primary somatic afferents, has two components: early and late. The early A-reflex has a short latency (25–50 ms) and arises from stimulation of low-threshold Aβ fibers. The early component is integrated segmentally requiring only an intact spinal cord. The late A-reflex has a long latency (80–120 ms) and arises from stimulation of low-threshold Aβ and high-threshold Aδ fibers. The late component requires medullary integration in the brainstem (Sato et al. 1969). If an experimental preparation is lightly anesthetized, a very late A-reflex can be recorded which requires an intact nervous system rostral to the pons (Sato 1972). The fibers mediating this very late component are unknown. The third reflex component, the C-reflex, was first described by Fedina et al. (1966), and Schmidt and Weller (1970) coined the name. The C-reflex has a long latency (250–500 ms) and arises from stimulation of the smallest-diameter unmyelinated primary afferents, the C fibers. By electrically stimulating a peripheral nerve, Schmidt and Weller (1970) identified the stimulation parameters necessary to consistently evoke the C-reflex.
The A- and C- somatosympathetic reflexes have been recorded in efferent sympathetic nerves in the cat and the rat. For example, electrical stimulation of a peripheral nerve evokes early and late A-reflexes in cardiac, renal, splanchnic, and adrenal sympathetic nerves (Araki et al. 1981, Coote & Downman 1966, Iwamura et al. 1969, Katunsky & Khayutin 1968, Kirchner et al. 1971, Miyamoto & Alanis 1970, Sato et al. 1969). In addition, electrical stimulation at strengths that recruit unmyelinated somatic afferents elicits the C-reflex in cardiac, renal, splanchnic, and adrenal sympathetic nerves (Araki et al. 1981, Fedina et al. 1966, Kimura et al. 1996, Nosaka et al. 1980, Sato et al. 1985). These reflex discharges were often evident only after cardiac- and respiratory-related bursts of sympathetic nerve activity were eliminated by baroreceptor denervation.
The elicitation of somatosympathetic re- flexes by electrically stimulating a peripheral nerve convincingly demonstrates the presence of neural circuitry by which somatic afferent input can affect efferent sympathetic output. However, electrical stimulation of a peripheral nerve is probably non-physiological, in that somatic afferents are activated synchronously and with discharge patterns that are probably unnatural. A number of studies have used relatively natural stimuli to determine the influence of somatic inputs on the sympathetic nervous system. These studies confirm that somatic afferents can reflexly affect the sympathetic nervous system. Somatosympathetic reflexes elicited by relatively natural stimuli to peripheral muscles and joints are presented below. Although stimulation of the skin can also elicit somatosympathetic reflexes (see Sato et al. 1997 for a review), these reflexes are not described here.
Contraction of skeletal muscle activates the sympathetic nervous system via a reflex whose afferent arm consists of small-diameter thinly myelinated (Aδ or group III) and unmyelinated (C or group IV) fibers (Kaufman & Forster 1996). For example, group III afferents discharge rapidly and vigorously to maintained and intermittent tetanic contractions as well as to twitch contractions (Hayward et al. 1991, Kaufman et al. 1983, Mense & Stahnke 1983, Paintal 1960). These types of muscle contraction have been shown to reflexly increase renal, adrenal, cardiac, and hindlimb sympathetic nerve discharge (SND) in both anesthetized and decerebrate cats (Gelsema et al. 1985, Hill et al. 1996, Matsukawa et al. 1990, McMahon & McWilliam 1992, Victor et al. 1989, Vissing et al. 1994). In addition to muscle contraction, muscle stretch also increases renal SND (Wilson et al. 1994). These somatosympathetic reflexes elicited by small-diameter afferents in skeletal muscle increase blood pressure, heart rate, and ventilation, and are thought to contribute to cardiovascular and respiratory adjustments during exercise (Coote et al. 1971, McCloskey & Mitchell 1972).
Somatosensory input from synovial joints also contributes to sympathetic nerve regulation via a reflex whose afferent arm is comprised of three types of sensory fibers innervating the joint capsule: Aβ, Aδ, and C-fibers (Langford & Schmidt 1983). Sato et al. (1985) recorded from the inferior cardiac nerve while applying passive movements to the knee joint of the cat. Joint movements in the normal working range of the knee typically did not influence activity in the cardiac sympathetic nerve unless the joint was inflamed. In the uninflamed joint, cardiac sympathetic nerve activity increased when the knee was rotated beyond the physiological range. These results indicate that high-threshold Aδ and C fibers from the knee joint directly affect cardiac sympathetic nerve outflow. However, Sato et al. (1983) showed that A-reflexes could be elicited when electrical stimulation of the articular nerve to the knee was at a strength that primarily activated Aβ fibers. Thus low-threshold input from synovial joints may provide input to sympathetic preganglionic neurons and influence somatosympathetic reflexes by summation of their subthreshold inputs.
Stimulation of afferents innervating axial/paraspinal tissues
In 1984, Sato and Swenson (1984) were the first to investigate vertebrosympathetic reflexes. They studied the effect of vertebral movement (mechanosensory stimulation) on adrenal and lumbar SND. Figure 3.1 is a schematic of their preparation. In chloralose/urethane-anesthetized rats, thoracic and lumbar vertebrae were isolated by surgical exposure and by removing their attached muscles. Lateral bending moments were induced between T11 and T12 or L3 and L4. Forces ranged between 0.5 and 3.0 kg. With removal of the musculature, somatic input was probably derived from facet joint tissues, intervertebral discs and/or intervertebral ligaments. Nerves to the kidney and adrenal gland were exposed and whole nerve recordings obtained. SND was quantified by threshold crossings of the electrical discharge. Crossings were counted as im-pulses and placed into 5-second bins.
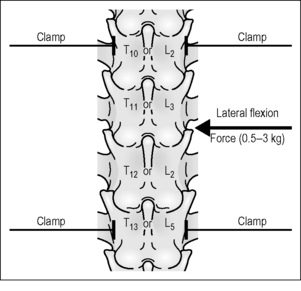
Figure 3.1 Schematic of the experimental approach used by Sato and Swenson (1984) to mechanically evoke vertebrosympathetic reflexes in the chloralose-anesthetized rat.
Vertebral movement was short in duration, lasting approximately 30 s. Lateral bending of the thoracic and lumbar spinal column reduced mean renal SND (Fig. 3.2, left panel). In the adrenal nerve, bending initially reduced mean SND, which was followed by a longer-lasting increase (Fig. 3.2, right panel). All decreases were 25–40% of baseline and appeared to attenuate rapidly. Maximal responses were found with 2.0 kg loads (19.6 N). A depressor response accompanied the changes in SND; both arterial blood pressure and heart rate decreased. This raised the possibility that the baroreflex had mediated the changes in SND. This possibility was assessed by cutting the carotid sinus and vagus nerves. Neither the decreases in renal nor adrenal SND were affected, but the denervation abolished the late increase in adrenal SND, indicating that the latter was baroreflex mediated as a result of baroreceptor unloading during the decrease in blood pressure (Fig. 3.2, both panels). Sato and Swenson further demonstrated that the decreased adrenal and renal SND was mediated reflexly. Cutting the lower thoracic and upper lumbar dorsal roots abolished the decreases, whereas cutting the dorsal roots at lower lumbar levels had no effect. Whereas the overall vertebrosympathetic response was inhibitory, the segmental portion of the reflex appeared excitatory because cutting the spinal cord between the upper two cervical vertebrae transformed the decreased SND into a 40% increase. The descending inhibitory supraspinal input could have been tonic, or initiated by the lateral bending itself.
In this study, the 0.5–3 kg applied loads were considered innocuous. However, this may need to be reconsidered. Loads in this range are 1.7–10 times greater than a rat’s body weight (assuming an average adult weight of 300 g), and it is not known whether the resulting strains approach the injury or failure limits of paraspinal tissues. In a previous study of the rabbit lumbar spine, axial loads five times body weight were considered noxious (Avramov et al. 1992).
Nearly 10 years after Sato and Swenson’s study, Budgell et al. (1997b) continued investigating vertebrosympathetic reflexes in Sato’s laboratory. In contrast to the previous study of mechanosensory effects, they investigated chemosensory effects in the form of a noxious algesic chemical, namely capsaicin. Stimulation was applied to the interspinous tissues of the thoracic and lumbar vertebral column, and adrenal nerve activity was recorded. In urethane-anesthetized rats, 20 μL of physiological saline or capsaicin (10 mM) was injected in the midline, at the cephalad margin of the caudal spinous process from T8 to T13 and from L3 to S1. Threshold crossings of adrenal nerve activity were counted as impulses and placed into 5-second bins.
Saline injected into either the thoracic or the lumbar interspinous tissues had no effect, whereas capsaicin injection increased adrenal SND by ∼︀80% (Fig. 3.3). Upper cervical spinal cord transection did not affect capsaicin-induced excitation in the thoracic spine and slightly reduced the excitation (to ∼︀50%) in the lumbar spine. The responses were considered reflexive in origin, based on adrenal nerve responses to electrical stimulation of the L2 medial branch of the dorsal ramus. This nerve innervates midline tissues in the rat vertebral column (Budgell et al. 1997a). Different somatic sensory fibers were recruited as stimulating parameters were increased. Adrenal nerve responses were considered reflexive because repetitive electrical stimulation of the L2 medial branch of the dorsal ramus evoked a C wave in the adrenal nerve (Fig. 3.4). The excitatory response persisted after spinalization similar to that after capsaicin; however, the C-fiber latency was shortened after spinalization, for reasons not discussed in the study. Thus, as in the mechanical studies of Sato and Swenson, noxious chemosensory stimulation produced an excitatory vertebrosympathetic reflex in the adrenal nerve. This response was thought to be mediated segmentally and, unlike the response to mechanical stimulation, may have little or no supraspinal component, but further work is necessary to confirm this owing to the change in latencies after spinalization.
< div class='tao-gold-member'>
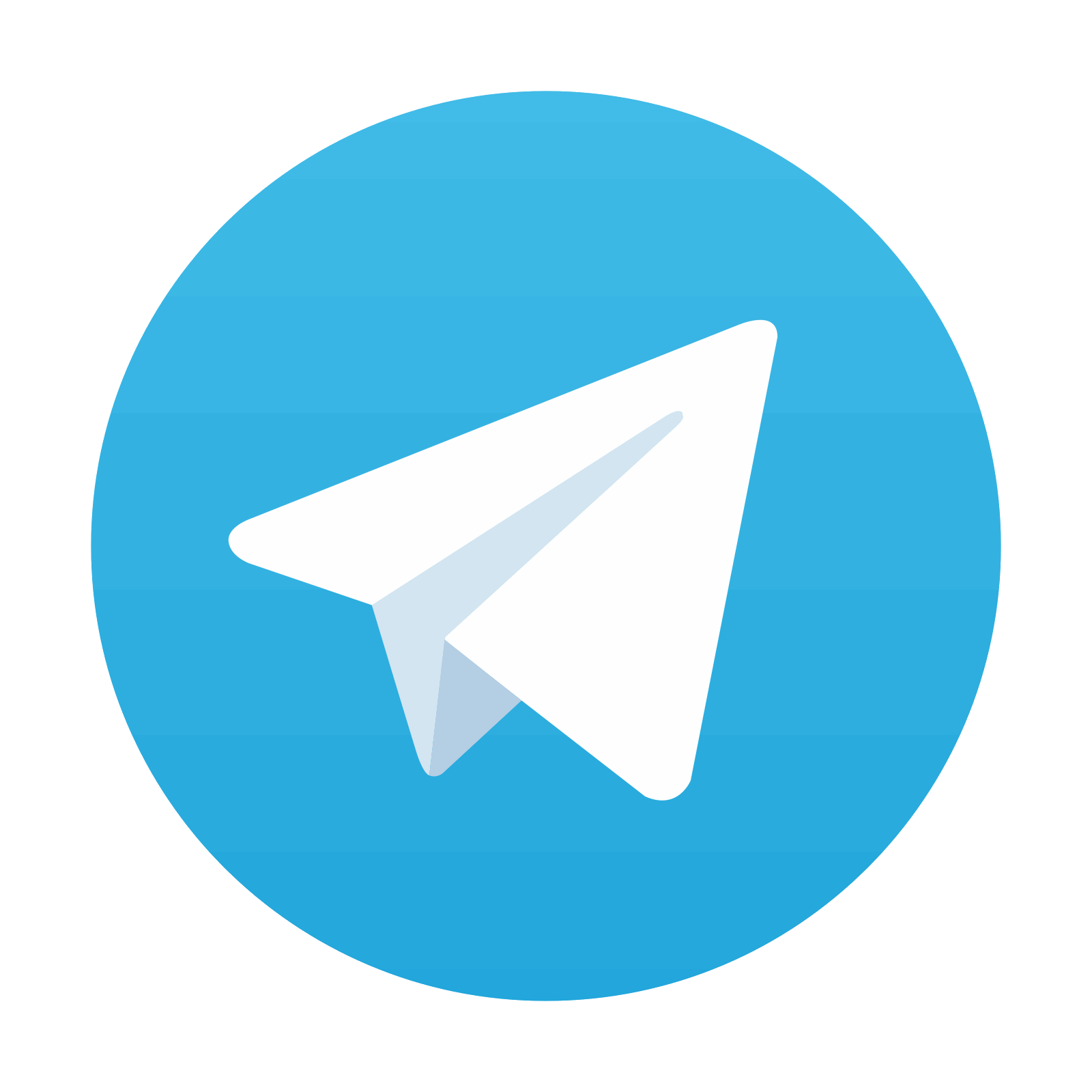
Stay updated, free articles. Join our Telegram channel

Full access? Get Clinical Tree
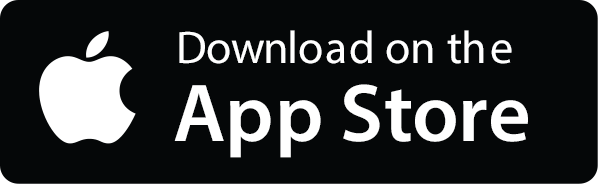
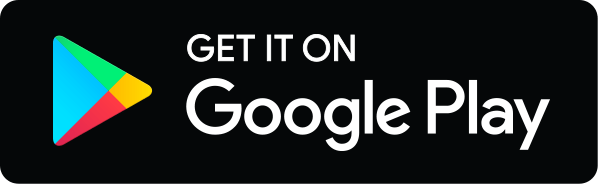