Fig. 2.1
Renderings of bone models of the femur, tibia, and fibula show how the varus laxity of the knee is determined. The other six laxities are determined in a similar way. In general, the laxity in a particular anatomic direction is the relative displacement (either translation or rotation) of the tibia on the femur under an applied load (either force or torque) from the relative position of the tibia on the femur under no applied load (i.e., the neutral or resting position) [7, 17, 18]
These seven laxities in the native knee have been reported by numerous studies [3, 13, 17–28]. Each study differs in the experimental setup used and the loads applied. While these differences lead to differences in the magnitude of the measured laxities, these studies generally agree that the laxities of the knee are negligible in extension andincrease with knee flexion (Fig. 2.2).


Fig. 2.2
Renderings of bone models of the femur, tibia, and fibula show the general pattern of the distraction laxity. In general, the V-V and I-E laxities follow this same general pattern where the laxity is negligible at 0° of flexion and is much greater at 90° of flexion
To provide some quantitative results, the following paragraphs present a representative set of the seven laxities measured in vitro in native knees using a robotic load application system [17, 18]. The V-V, I-E, A-P, and D laxities were determined under applied loads of ±5 Nm [20], ±3 Nm [3], ±45 N [21], and 100 N [29], respectively. These loads were selected to just engage the soft tissue restraints [3, 20, 21].
In V-V, the knee has negligible laxity at 0° of flexion where the varus laxity (mean ± standard deviation) is 0.7° ± 0.3°, and the valgus laxity (mean ± standard deviation) is 0.4° ± 0.2° (Fig. 2.3a). Both V-V laxities increase with knee flexion, but the varus laxity increases more (five times greater on average at 90° of flexion than that at 0° of flexion) than the valgus laxity (three times greater on average at 90° of flexion than that at 0° of flexion).


Fig. 2.3
The column graphs show the laxities of the native knee (columns are the means and error bars are the standard deviations) at 0°, 45°, and 90° of flexion in (a) V-V, (b) I-E, (c) A-P, and (d) D. An asterisk indicates that the laxity at either 45° or 90° of flexion is greater than that at 0° of flexion [17]
In I-E, the knee is also the least lax at 0° of flexion (Fig. 2.3b). At 0° of flexion, the internal rotation laxity (mean ± standard deviation) is 4.6° ± 1.4°, and the external rotation laxity (mean ± standard deviation) is 4.4° ± 1.7°. Both I-E laxities increase from 0° to 45° of flexion (three times greater on average at 45° of flexion than that at 0° of flexion) and stay relatively constant and symmetric from 45° of flexion to 90° of flexion.
In A-P, the knee is also least lax at 0° of flexion (Fig. 2.3c). At 0° of flexion, the anterior translation laxity (mean ± standard deviation) is 2.1 ± 0.5 mm, and the posterior translation laxity (mean ± standard deviation) is 2.4 ± 1.2 mm. Both A-P laxities are greatest around 45° of flexion where the anterior translation laxity is on average three times greater than that at 0° of flexion, but the posterior translation laxity is not significantly greater than that at 0° of flexion.
In distraction, the knee is also least lax at 0° of flexion (Fig. 2.3d). At 0° of flexion, the distraction laxity (mean ± standard deviation) is 0.6 ± 0.1 mm. Similar to the I-E laxities, the distraction laxity increases from 0° to 45° of flexion (three times greater on average at 45° of flexion than that at 0° of flexion) and then remains fairly constant throughout the rest of flexion.
2.3 Using the Soft Tissue Balance of the Native Knee to Guide Balancing During TKA
If the preferred functional outcome after TKA is restoration of native biomechanics, then the soft tissue balance of the native knee is a reasonable choice to guide balancing during TKA. As previously mentioned, the native knee is inherently stable and has adequate mobility to enable individuals to perform a wide range of activities without pain, stiffness, or feelings of instability. Additionally, the laxities of the knee can be measured pre-, intra-, and postoperatively which allows the laxities of the native knee to be translated into clinical practice. There are two complexities that surgeons should be aware of when applying the previously described laxities in clinical practice. The first complexity is that at the time of surgery, the patient’s soft tissue balance has changed from native due to the degenerative changes present in the joint [30–33]. Degradation of the articular surfaces increases the laxities of the knee by bringing together the origin and insertion of the soft tissue restraints (i.e., pseudo-ligamentous laxity) [34]. Growth of osteophytes often re-tensions the soft tissue restraints which in turn reduces the laxities of the knee [35]. Remodeling of the soft tissue restraints (e.g., either lengthening or contracture) may also change the laxities of the knee [30].
A second complexity is that soft tissue balance in the native knee has wide variability in terms of the laxities [18, 36]. This is likely caused by the variability in both the mechanical properties of the soft tissue restraints [37–40] and shapes of the articular surfaces [41]. This variability demonstrates that average laxities reported in the literature are unlikely to represent the native laxities of an individual patient. Hence, striving to achieve the same soft tissue balance in all patients during TKA is unlikely to restore the native soft tissue balance for a particular patient. It is important to note that the laxities do not vary widely at 0° of flexion [18], and therefore the average values at 0° of flexion are reasonable estimates of the native laxities of a particular patient at 0° of flexion.
The contralateral knee should be considered when determining how to approach these complexities. If the patient’s contralateral knee has no to mild degenerative changes, then the laxities of the contralateral knee may be used to define the native soft tissue balance for that patient because side-to-side differences in the laxities are negligible [42]. However, if the contralateral knee also has moderate to severe degenerative changes, then the surgeon must assume that the previously reported average laxities at 0° of flexion are native for the patient.The soft tissue balance can then be checked in a relative sense based on the expected increases in the laxities with flexion relative to those at 0° of flexion (Fig. 2.3).
2.4 Potential Consequences of Not Restoring Native Soft Tissue Balance
Although complexities exist with striving to restore the native soft tissue balance, surgeons should be aware that there are at least three potential consequences for not striving to restore native soft tissue balance. The first consequence is that overly tight soft tissue restraints compared to native may cause abnormal kinematics. For example, studies have reported that patients following gap-balanced TKA exhibit abnormal kinematics in flexion [43, 44]. The goal of gap balancing in terms of soft tissue balance is to tighten the knee in flexion so that the laxities in flexion match those in extension [1, 45–47]. If the ideal balance is achieved in a gap-balanced TKA, then the knee will be overly tight in flexion especially in the lateral compartment [17]. One possible explanation for these abnormal kinematics is that the overly tight soft tissue restraints in flexion especially in the lateral compartment may limit the posterior translation of the femur on the tibia. This translation is present in the native knee and is necessary for achieving deep flexion [48–51]. This consequence is supported by a recent clinical study that showed patients preferred a more lax knee after TKA [52].
A second consequence is that soft tissue releases will frequently be required [53–57]. Soft tissue releases have several detrimental effects on the knee. First, soft tissue releases increase multiple laxities [58, 59]. Therefore, a release performed to achieve the desired change in one laxity may increase other laxities that do not need to be changed. Second, it is difficult to control the amount of release performed, and hence under- and over-release of the soft tissue restraints are possible [56, 60, 61].
The third consequence is that non-native tensions in the soft tissue restraints may feel abnormal to the patient because the soft tissue restraints are innervated with both proprioceptors and mechanoreceptors [27, 62–66]. Hence, even subtle changes in the soft tissue balance may be perceived as pain, stiffness, or instability by the patient [27, 67].
2.5 Summary
The soft tissues are “balanced” in the native knee because the joint is inherently stable and has adequate mobility for individuals to perform a wide range of activities. As such, restoration of the native soft tissue balance is a reasonable goal for soft tissue balancing in TKA to best restore native biomechanics and provide the patient with a knee that feels as close to normal as possible. Failing to restore native soft tissue balance may lead to instability, stiffness, limited motion, pain, or an abnormal feeling knee.
The benefits of striving to restore native soft tissue balance are evident in the positive clinical outcomes following kinematically aligned TKA. In kinematically aligned TKA, the components are aligned to best restore the native articular surfaces, and the surgeon strives to restore the native soft tissue balance without soft tissue releases [68]. A recent cadaveric study showed that kinematically aligned TKA does closely restore native soft tissue balance as judged by the laxities [69]. Patients after kinematically aligned TKA have better pain relief, have higher functional scores, achieve greater flexion, have more normal kinematics, and are three times more likely to report that their knee feels normal than patients after mechanically aligned TKA, in which surgeons most often strive to achieve balanced gaps [70–73].
References
1.
Sikorski JM. Alignment in total knee replacement. J Bone Joint Surg Br. 2008;90(9):1121–7. doi:10.1302/0301-620X.90B9.20793.CrossRefPubMed
2.
Heesterbeek PJ, Haffner N, Wymenga AB, Stifter J, Ritschl P. Patient-related factors influence stiffness of the soft tissue complex during intraoperative gap balancing in cruciate-retaining total knee arthroplasty. Knee Surg Sports Traumatol Arthrosc. 2015:1–9. doi:10.1007/s00167-015-3694-5.
3.
Blankevoort L, Huiskes R, de Lange A. The envelope of passive knee joint motion. J Biomech. 1988;21(9):705–20. doi: 10.1016/0021-9290(88)90280-1.
4.
Schipplein OD, Andriacchi TP. Interaction between active and passive knee stabilizers during level walking. J Orthop Res. 1991;9(1):113–9. doi: 10.1002/jor.1100090114.
5.
Dyrby CO, Andriacchi TP. Secondary motions of the knee during weight bearing and non-weight bearing activities. J Orthop Res. 2004;22(4):794–800. doi:10.1016/j.orthres.2003.11.003.CrossRefPubMed
6.
Wilson DR, Feikes JD, O’Connor JJ. Ligaments and articular contact guide passive knee flexion. J Biomech. 1998;31(12):1127–36. doi: 10.1016/S0021-9290(98)00119-5.
7.
Halewood C, Amis AA. Clinically relevant biomechanics of the knee capsule and ligaments. Knee Surg Sports Traumatol Arthrosc. 2015;23(10):2789–96. doi:10.1007/s00167-015-3594-8.CrossRefPubMed
8.
Davidson BS, Judd DL, Thomas AC, Mizner RL, Eckhoff DG, Stevens-Lapsley JE. Muscle activation and coactivation during five-time-sit-to-stand movement in patients undergoing total knee arthroplasty. J Electromyogr Kinesiol. 2013;23(6):1485–93. doi:10.1016/j.jelekin.2013.06.008.CrossRefPubMed
9.
Meier W, Mizner RL, Marcus RL, Dibble LE, Peters C, Lastayo PC. Total knee arthroplasty: muscle impairments, functional limitations, and recommended rehabilitation approaches. J Orthop Sports Phys Ther. 2008;38(5):246–56. doi:10.2519/jospt.2008.2715.CrossRefPubMed
10.
Thomas AC, Judd DL, Davidson BS, Eckhoff DG, Stevens-Lapsley JE. Quadriceps/hamstrings co-activation increases early after total knee arthroplasty. Knee. 2014;21(6):1115–9. doi:10.1016/j.knee.2014.08.001.CrossRefPubMed
11.
Petterson SC, Mizner RL, Stevens JE, Raisis L, Bodenstab A, Newcomb W, Snyder-Mackler L. Improved function from progressive strengthening interventions after total knee arthroplasty: a randomized clinical trial with an imbedded prospective cohort. Arthritis Rheum. 2009;61(2):174–83. doi:10.1002/art.24167.CrossRefPubMed
12.
Athwal KK, Hunt NC, Davies AJ, Deehan DJ, Amis AA. Clinical biomechanics of instability related to total knee arthroplasty. Clin Biomech (Bristol, Avon). 2014;29(2):119–28. doi:10.1016/j.clinbiomech.2013.11.004.CrossRef
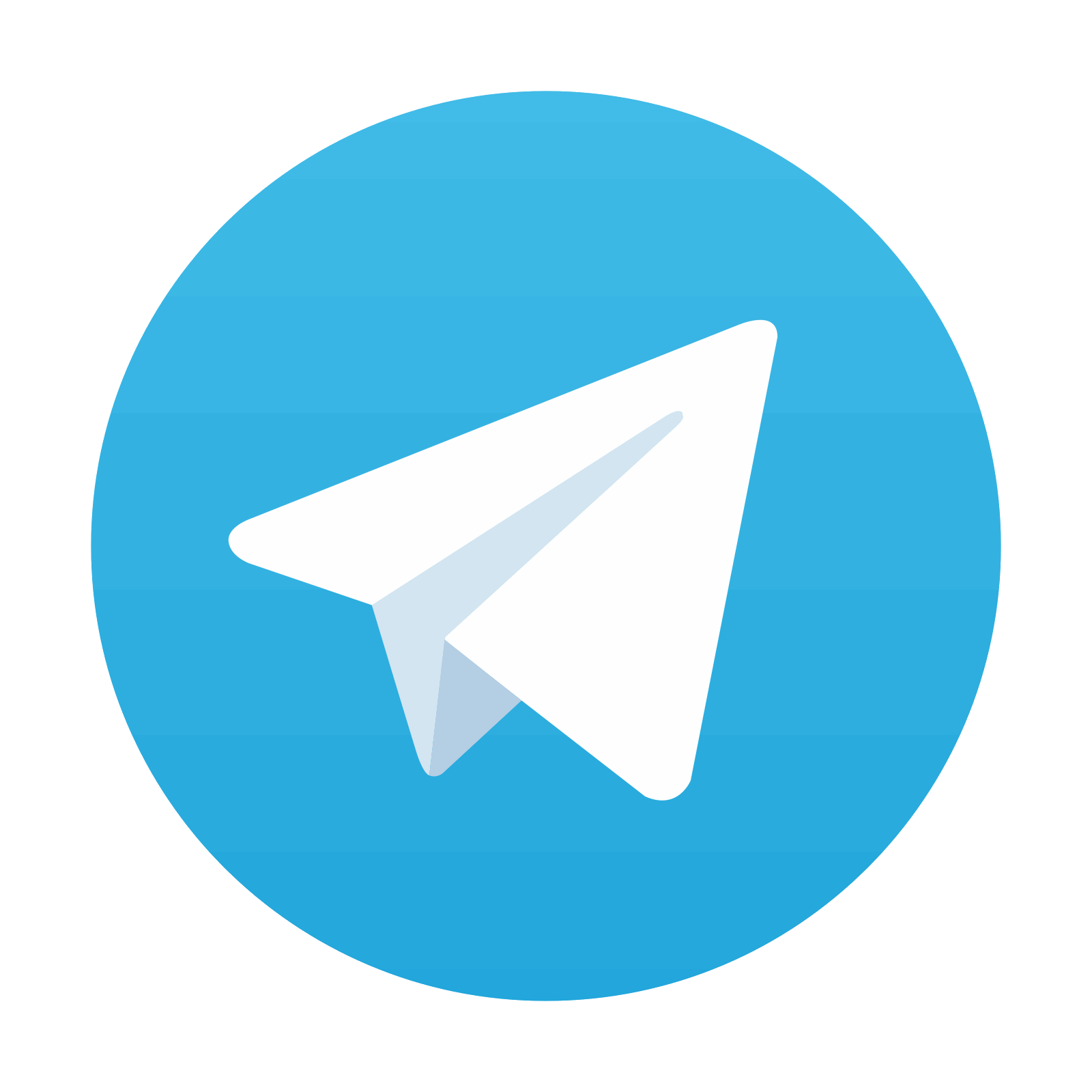
Stay updated, free articles. Join our Telegram channel

Full access? Get Clinical Tree
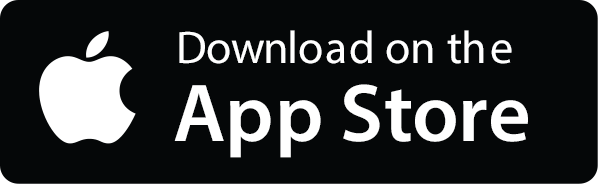
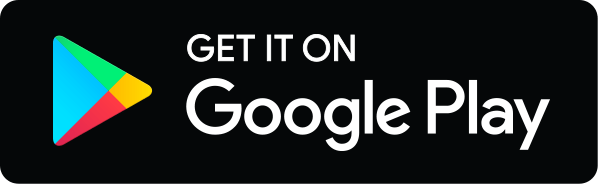