Fig. 4.1
Among the diverse disciplines and events that the sport of skydiving encompasses are formation skydiving and vertical formation skydiving (a), in which teams of skydivers build predetermined sequences of free fall formations as fast as possible. Wingsuit flying (b) utilizes garments that enhance the free fall glide ratio, and in large formation jumps (c), skydivers strive to build large free fall formations, sometimes in predetermined sequences. Freeflying and freestyle skydiving (d) are artistic events in which free fall moves are scored by judges in a manner somewhat similar to figure skating. In canopy piloting (e), also called swooping, an athlete performs a high-speed landing of a wing parachute judged by speed, distance, or accuracy. In canopy formation (f), teams of skydivers build sequences of formations with opened wing parachutes as fast as possible (Photos courtesy of Ori Kuper and Craig O’Brien)
Freeflying, freestyle skydiving, and skysurfing are artistic events in which free fall moves performed by two or more skydivers are scored by judges in a manner somewhat similar to figure skating, but with the peculiar feature that one of the team members wears a video camera and records the video to be judged, encompassing both free fall flying skills in all three dimensions and cinematography into the artistry. Judging criteria are separated in technical and presentation items. In free fall style, an athlete performs a predetermined sequence of free fall maneuvers in the least amount of time possible.
During speed skydiving (claiming the title of “fastest nonmotorized sport on earth”), an athlete strives to achieve and maintain as high free fall vertical velocity as possible, typically by diving head down. The current speed skydiving world record is 527 km/h [10]. This is to be compared with the more typical terminal vertical velocity of a skydiver falling straight down, belly-to-earth, of around 200 km/h, or circa 55 m/s.
Canopy formation is similar to formation skydiving but with opened wing parachutes – teams of skydivers build sequences of parachute formations as fast as possible. In canopy piloting, also called swooping, an athlete performs a high-speed landing of a wing parachute judged by speed, distance, or accuracy. Accuracy landing is a separate landing event using larger and slower wing parachutes for centimeter accuracy. Para-ski combines accuracy landing with giant slalom skiing, the landing pad being located on a snowy slope of between 25 and 35°. Free fall style and accuracy landings are the two oldest competitive events in skydiving.
Other events include large formation jumps (“big ways”), night jumps, and high-altitude jumps. The latter require supplemental oxygen and are sometimes referred to as HALO jumps, acronym for high-altitude low opening, and HAHO jumps, acronym for high-altitude high opening. The highest parachute jump to date was made on August 16, 1960, when Joseph Kittinger in the US Air Force jumped from 31-km (102,800 ft) altitude above New Mexico [1, 11]. Attempts to break this record are underway.
The Equipment Used: Essential and Safety Requirements
The Parachute
The technical term for a parachute is aerodynamic decelerator, meaning that it slows down motion against air. The wing parachute is commonly referred to as a ram-air parachute, in reference to it being pressurized into its wing (air-foil) shape by ram-air intake of air in the front. This was the invention of Domina Jalbert: a wing that self-inflates by ramming air. To reduce opening shock, skydiving ram-air parachutes are fitted with a reefing device, typically a rectangular piece of fabric called a “slider” that slides down the lines on grommets during the parachute opening sequence. Parachute deployment is initiated by releasing a small round parachute, called a pilot chute, that anchors into the airstream and pulls out the wing parachute. An overview of modern, state-of-the-art skydiving equipment is given in Fig. 4.2.
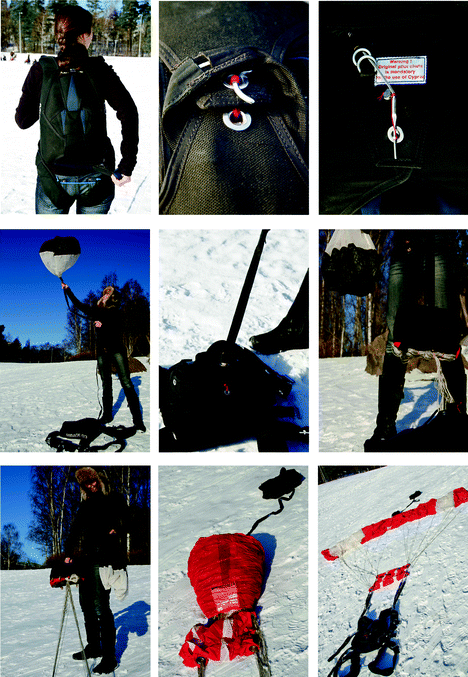
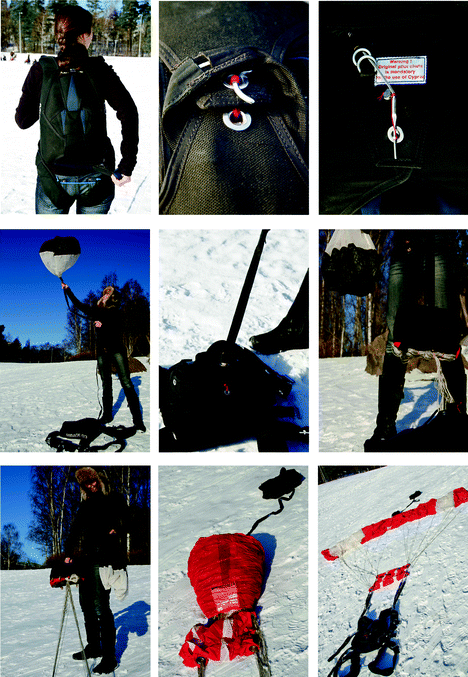
Fig. 4.2
Overview of skydiving equipment. Top row from left: A standard piggyback skydiving harness. It contains a main and a reserve parachute, packed in separate containers closed by metal closing pins. Should the main parachute malfunction, it is manually disconnected with a “cutaway” handle, and the reserve deployed by means of the reserve rip cord. This equipment is fitted with an automatic activation device, installed inside the reserve container, which activates the reserve at a preset altitude and vertical velocity, if the skydiver has lost altitude awareness or become incapacitated. In a pouch at the bottom of the main container is a small round parachute, called a pilot chute. Middle row from left: Main parachute deployment is initiated by manually releasing the pilot chute into the airstream, where it acts like an anchor and pulls out the deployment bag with the main parachute from its container. The lines are stowed with rubber bands on the deployment bag. Bottom row from left: The lines are stretched and the wing parachute emerges from the deployment bag. To reduce the opening shock, skydiving ram-air parachutes are fitted with a device that slows down the inflation. Typically, this is a rectangular piece of fabric called a “slider” that catches air like a small sail and slides down the lines on grommets during the parachute opening sequence (Photos courtesy of Linda Persson)
Today, high-performance skydiving main parachutes are regularly flown and landed at speeds exceeding 100 km/h and require a bit of a runway to land safely, that is, a large open level field. During extreme high-speed landing approaches, they can achieve sink rates nearing a skydiver in free fall terminal vertical velocity. In skydiving vernacular, both round drag parachutes and rectangular ram-air wing parachutes are called “canopies,” hence the names for the events canopy piloting and canopy formation.
The Reserve Parachute
Skydivers leave the aircraft carrying two packed separate parachutes, a main parachute and a reserve parachute. These are almost independent of each other, meaning that the risk of a double malfunction, that both should fail on the same jump, is very small. The philosophy of the skydiving reserve parachute is built upon two heights: one being the lowest required altitude for the reserve parachute to deploy and the other being the lowest required altitude for the main to deploy. Added, these two form the lowest required altitude for main parachute activation. Current Swedish regulations set this imaginary glass floor at 700 m (higher for several specific types of jumps) [12]. At a sink rate of 55 m/s, typical for a skydiver falling straight down, belly-to-earth, this equals to 13 s before impact. If the used reserve parachute model and configuration has a lowest required altitude to deploy of circa 300 m, the altitude margin from the lowest required altitude for main parachute activation is 700–300 = 400 m, equal to 7 s. Visual altimeters are usually worn on the wrist or chest strap and audible altimeters inside the helmet.
Since independence of the two parachute systems is paramount, activating the reserve in response to a main malfunction entails pulling two handles: First, the skydiver disconnects (“cuts away” in skydiving vernacular, though there is no cutting involved) the failed primary system and then activates the secondary. If this is performed in reversed order, the reserve canopy may entangle with the malfunctioned main canopy. A very dangerous situation arises if fabric or lines become tangled around the athlete. A few years ago in Sweden, a student skydiver practicing free fall flight techniques on his own, in accordance with the training program (it was his second free fall jump from 1,500 m), flipped over on his back when he pulled the main parachute, and it entangled with his foot. He disconnected the main parachute from the harness but it was still wrapped around his foot, and when he pulled the reserve parachute, it entangled with the entangled main parachute. He died on impact some seconds later.
Automatic Reserve Activation Devices
Responding to a persistent problem of no-pull (no parachute activation) and low-pull (too low parachute activation) fatalities, the industry developed a new generation of automatic reserve activation devices (AAD) that came out on the market in the 1990s [13]. The reliability of these led to widespread acceptance and use, reducing the number of no-/low-pull fatalities markedly. For the year 2009, at least 38 lives saved by AADs were reported to the IPC, and 10 of the reported 2009 fatalities (16 % of 62) might have been avoided by the use of an AAD [9].
Wing Loading
Various types of garments that enhance the jumper’s free fall glide ratio (ratio of the horizontal to vertical distance traveled), such as the wingsuit (Fig. 4.3), are widely used today in sport parachuting. The wingsuit follows the same concept of the wing parachute, using layers of fabric between arms and body and between legs. During the fall, the air is pressurized into the ram-air wing shape cells. Some wingsuit designs give the parachutist a maximum glide ratio of >3:1 [14], similar to a high-performance skydiving main parachute. The most important factor to determine the glide ratio is the athlete himself, with his ability to control and navigate the wingsuit. A wingsuit, a very small skydiving main parachute, a large skydiving main parachute, a skydiving reserve parachute, and a BASE parachute can all be considered a continuum of variations of Domina Jalbert’s ram-air fabric wing. What differs between them is the ratio of total suspended weight to wing platform area of the wing. This measure, called wing loading, is an important determinant of airspeed. Wingsuits have high wing loadings and consequently fly at high airspeeds and are as yet not intended for landing. BASE parachutes have low wing loadings and consequently fly at low airspeeds and are very soft to land. Highly loaded skydiving main parachutes can be flown at speeds approaching wingsuits.
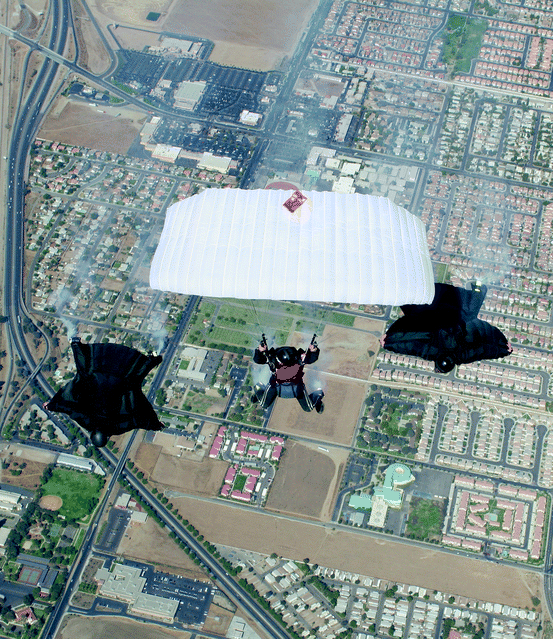
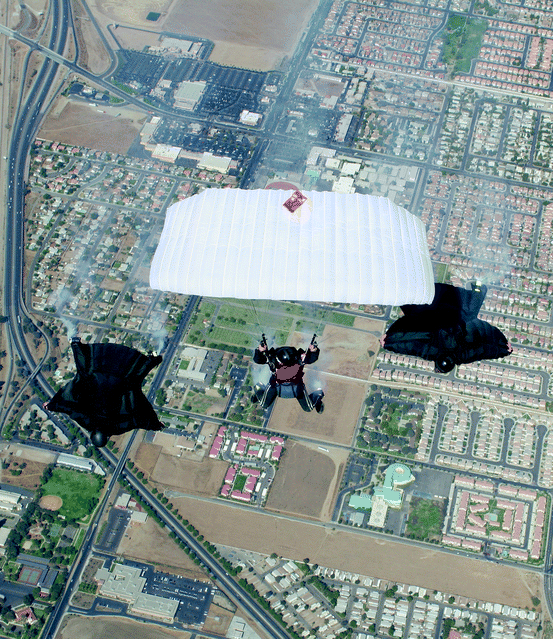
Fig. 4.3
A skydiver flying a wing parachute flanked by two skydivers flying wingsuits. The wing parachute and wingsuits alike are pressurized into their wing shapes by ram-air intake of air (Photo courtesy of Craig O’Brien)
Supplemental Oxygen
The regular exit altitude in skydiving, routinely using unpressurized aircraft without supplemental oxygen, is 4,000 m (13,000 ft). This is higher than the 3,000-m (10,000 ft) altitude at which hypobaric hypoxia (oxygen deficiency caused by low air pressure) is believed to be of physiologic importance. The rationale for this practice is an assumption that the relatively brief durations (usually less than half an hour) of hypobaric exposure do not cause hypoxic incapacitation to a significant degree [11]. In-aircraft supplemental oxygen is used when the exit is at higher altitudes. During the large formation world record jumps over Thailand in 2004 and 2006, over 400 skydivers jumped out of multiple C-130 Hercules aircraft at >7,300 m (>24,000 ft). The in-aircraft oxygen system used for these jumps were individual oxygen hoses fed into the skydiving helmets, without oxygen breathing masks (H. Berggren, 2011, Thailand 2004 big way hog hojd, Personal Communication; J. Hansson, 2011, Skydiving_chapter_of_Adventure_and_Extreme_Sports_Injuries2 Kommentar LUL5, Personal Communication; S.Mörtberg, 2011, world team 2006, Personal Communication) [15, 16].
Injury and Fatality Rates and Specific Types of Injury
Fatalities
International fatality statistics are collected by the IPC and published as an annual report (Table 4.1). The 2009 number was one fatality per 88,000 jumps [9]. Skydiving in Sweden during the years 1994–2003 suffered nine fatalities in 1.1 million jumps, or one fatality per 125,000 jumps [17], a risk slightly less than maternal deaths per live birth in Sweden during the same period (Mödradödlighet (dnr 32346/2011), 2011, Personal Communication) [18]. A review of postmortem autopsy records in 22 fatalities in Swedish skydiving 1964–2003 showed massive injuries to the central nervous, cardiovascular, respiratory, musculoskeletal, and urinary systems and to the liver, spleen, pancreas, and skin. In many cases, more severe injuries were found in body parts having hit the ground first. One skydiver in this group who fell into a lake with no parachute inflated died with severe lacerations of the central nervous system [17]. Main causes of fatal skydiving incidents reported to the IPC for 2009 (40 countries, 62 fatalities) were “fast canopies” (e.g., fast wing parachutes) – 12 (19 %), “landing errors” – 11 (18 %), “no/low main pull” – 7 (11 %), tandem – 7 (11 %), and “canopy collisions” (e.g., midair wing parachute collisions) – 6 (10 %). The IPC concluded that 44 (71 %) of the 62 fatalities in 2009 happened with the jumper having at least one good parachute on his or her back, and that 49 (79 %) of the 62 fatalities may have been caused by human error [9]. The introduction of high-performance wing parachutes in the 1990s has rendered it the epithet “A Decade of Landing Deaths” [19]. Between 1986 and 2001, 507 people in the United States died from injuries sustained in skydiving, with an increase in landing fatalities with open parachute and fully functional gear, but without change of overall fatality rate [20]. Thus, perhaps counterintuitive to a non-skydiver, it may be concluded that the fatality risk in a skydive is not exclusively related to whether the parachute will open or not but also to the parachutist’s piloting skills of a perfectly functioning parachute. When flying and landing a highly loaded skydiving main parachute, the margin of error is small.
Table 4.1
The international fatality rate reported to the International Parachuting Commission of the Fédération Aéronautique Internationale 1989–2009 [9]
Year and number of countries | Number | Number | Number | Jumps | Jumpers per fatality | |
---|---|---|---|---|---|---|
Jumpers | Jumps | Fatalities | Per jumpers | Per fatality | ||
1989 – 34 countries | 340,715 | 5,564,137 | 97 | 16 | 57,362 | 3,513 |
1990 – 32 countries | 316,994 | 5,189,991 | 70 | 16 | 74,143 | 4,528 |
1991 – 35 countries | 245,162 | 4,848,025 | 74 | 20 | 65,514 | 3,313 |
1992 – 35 countries | 300,586 | 4,591,980 | 59 | 15 | 77,830 | 5,095 |
1993 – 38 countries | 370,679 | 5,267,754 | 101 | 14 | 52,156 | 3,670 |
1994 – 40 countries | 285,253 | 5,064,125 | 70 | 18 | 72,345 | 4,075 |
1995 – 38 countries | 322,322 | 5,562,691 | 64 | 17 | 86,917 | 5,036 |
1996 – 40 countries | 323,300 | 6,013,691 | 76 | 19 | 79,128 | 4,254 |
1997 – 37 countries | 404,198 | 6,843,299 | 78 | 17 | 87,735 | 5,182 |
1998 – 33 countries | 332,603 | 5,596,753 | 72 | 17 | 77,733 | 4,619 |
1999 – 26 countries | 335,867 | 5,594,191 | 60 | 17 | 93,237 | 5,598 |
2000 – 27 countries | 355,405 | 5,750,464 | 63 | 16
![]() Stay updated, free articles. Join our Telegram channel![]() Full access? Get Clinical Tree![]() ![]() ![]() |