Fig. 25.1
Skate anatomy
The exterior of skates was traditionally leather, but gradually has been substituted with synthetic materials. Graphite and polypropylene materials have been added for strength and protection of the boot with flex points added to allow proper ankle joint plantarflexion in the skating motion . The toe cap is always rigid for toe protection.
The next part of a skate is the skate blade housing. This portion of the skate is riveted or screwed onto the boot itself. The attachment of the blade housing to the boot can be a point of biomechanical input. This housing can be moved medial to lateral, or anterior to posterior on the boot. Its standard position is to hold the blade centrally under the heel and then to continue forward under the second metatarsal head and further forward through the second digit. The blade housing can also act as an attachment site for heel lifts and wedges as they are sandwiched between the housing and boot. The portion of the rivet inside the boot can be a potential source of irritation.
The last anatomical portion of a skate is the narrow skate blade itself. This portion of the skate is traditionally made of stainless steel and is a necessity given the surface of the activity but can be adjusted in many ways for specific biomechanical effect. It is rockered front to back and can be varied for desired performance. The rocker acts as a balance point with as little as 1 in. contacting the ice. A longer radius of curvature allows for more blade to contact the ice and thus can improve balance and speed. A shorter radius of curvature increases the ease of turning and improves maneuverability. The bottom surface of the blade is hollow ground to create a medial and lateral edge or bite angle, Fig. 25.2. This curvature of hollow can be altered at the time of sharpening to get a desired bite into the ice. The technology in skate blades is ever changing. One newer technology is disposable titanium blades that can be purchased with varying degrees of rocker and with varying curvatures of hollow. These blades stay sharper longer than traditional stainless steel blades and can be easily removed and replaced when worn out or damaged. An even more recent advancement in blade manufacturing is the ability to buy blades with varying bite angles. These blades are presently being tested to prove their expected capability to increase speed and turning ability.


Fig. 25.2
Frontal plane blade-ice contact; surface is hollow ground along its length
Goalie Skates
Goaltender skates are similar to player skates, with some obvious differences specific to the requirements of the position, Fig. 25.3. The boot itself does not go as high up the lower leg as traditional skate boots. This is primarily due to the need for increased ankle mobility in this position. Also, the skate blade housing encompasses the entire lower portion of the boot to create a plastic housing of protection for the foot. The blade has a longer radius of curvature to help improve balance, as well as a wider width to avoid breakage. All the same adjustments that can done in a player’s hockey skate can also be done on a goalie skate.


Fig. 25.3
Goalie skates
In-Line Skates
Two major components differentiate in-line skates from traditional hockey skates. The most obvious is that instead of a blade, wheels are attached due to the difference in sport surface. Just like skate blades can be changed for rocker and material, so can the types and sizes of wheels be altered. The second major structural difference is the ventilation systems used to accommodate heat transfer as most in-line skating is done in a warmer environment than traditional ice skating, Fig. 25.4.


Fig. 25.4
In-line skates
Figure Skates
The design of figure skates as we know them today has changed very little in over a century. The biggest changes, like those seen in hockey skates, are with construction materials. Figure skating boots are designed to provide the foot and ankle with the stability necessary to perform difficult jumps and spins; however, this rigidity brings with it a myriad of lower extremity problems and injuries. The figure skate blades vary in that there are “picks” at the anterior end of the blade to help with function and maneuverability, and the radius of curvature is longer than that of a traditional hockey skate blade.
Since 1990 the actual art of skating figures, also known as compulsories, has been eliminated from the sport , and has been replaced with increased emphasis on free skating, which includes jumps, spins, lifts, and throws [1]. The stiffness of skate boots has long been linked to lower extremity injuries in figure skating, and the increased amount of time spent practicing jumps may result in a greater frequency and degree of severity of these injuries [2]. Skaters may perform 50–100 jumps per day, 6–7 days a week, and the force generated from a typical skating jump amounts to eight to ten times the skater’s body weight [3]. Because the design of figure skates allows for very little flexion at the ankle, skaters land on their heels, and since the hardness of the ice surface offers almost no shock absorption, most of the force is transferred to the knees, hips, and spine. Most figure skating injuries involve the lower extremity, and many are directly related to the skating boot [4].
Figure skating is the only jumping sport that confines the movement of the ankle joint and calf muscles by the use of rigid boot support [5]. The skate boot is designed with a high heel and inflexible ankle portion that limits ankle plantar flexion, which decreases effectiveness in jump takeoff and restricts the ability of the ankle to cushion the landing [5]. The force absorbed by the knee extensor apparatus contributes to occurrence of patellofemoral pain and various overuse injuries. In a study by Dubravic-Simunjak et al., 42.8% of female and 45.5% of male singles skaters reported overuse syndromes, the most frequent injuries in females being stress fractures and jumper’s knee in males.
Not only does the design of figure skate boots cause injuries, but poor fit can also lead to deformities of the foot. In an article for the US Figure Skating website, Linda Tremain reports that up to 57% of skaters have bunions, while 31% have enlarged navicular bones, likely related to uncorrected pronation problems of the boot and blade or the foot itself . She also found that excess heel slippage has led to the development of Haglund’s deformities in 49% of skaters, and hammertoes in 18%. Current research and trials are under way to design an articulated boot that may decrease the magnitude of landing forces by allowing more sagittal plane mobility while still providing the stability required to execute difficult jumps and spins [6].
Speed Skates
In contrast to the unchanged design of figure skates, speed skates have undergone radical changes in the past decade, which have brought about tremendous improvements in both world record and personal best times.
Conventional speed skates featured a low boot with a long, thin blade that was fixed to the boot. A skater would push off until the leg was fully extended, at which point the ankle would naturally want to plantar flex and continue the push. However, this would cause the toe of a conventional skate to dig into the ice, called “toeing off,” which hinders the gliding motion [7]. To prevent the tip of the blade from scratching the ice, speed skaters had to use a technique where plantar flexion was largely suppressed during push-off. This limits the efficiency of the stroke because the ankle plantar flexors are prevented from contributing to the push, which also restricts the work done by the calf muscles and knee extensors and causes the skater to lose contact with the ice before the knee is completely extended [8]. Speed skaters often suffered from pain in the tibialis anterior due to this forced suppression on plantar flexion [7].
The clap skate was introduced in 1997 which features a hinge under the ball of the foot that allows the ankle to plantar flex at the end of push off while the blade continues to make contact with the ice. Skating velocity increased by 5% and mean power output improved by 10% due to an increase in both stroke frequency and work per stroke [9]. Surprisingly, this improvement is not simply due to the ability of the ankle to extend at push-off, but rather the difference in the center of rotation between the foot and the ice surface [10]. With conventional speed skates, the foot is turned into a long lever because it must rotate around the tip of the skate blade, which is located approximately 10 cm in front of the toes [10]. This extreme frontal location of the center of rotation creates an ineffective push-off and makes it difficult to set the foot into motion. The hinge of a clap skate allows the foot to rotate around the ball of the foot, which greatly enhances the effectiveness of plantar flexion in the final phase of push-off, leading to increased gross efficiency and mechanical power output [9].
The hinge must be located under the ball of the foot to achieve optimal performance; however, the exact position varies from skater to skater depending upon his or her build and skating technique [10]. Determining the most advantageous location for the hinge is still a question of feel.
Understanding basic skate boot construction along with an understanding of the biomechanics of the sport can assist in making functional interventions in both performance and comfort .
Biomechanics
All the various forms of skating along with the associated footwear are somewhat different. However, the basics of motion, push, and glide are similar as is the performance surface. So for discussion purposes regarding biomechanics we will discuss the biomechanics of power skating.
Power skating in hockey involves skating forward and backward and with multiple directional changes as the game evolves. It is this ever-changing movement pattern that makes this activity difficult to study from a biomechanical standpoint. It is forward acceleration and striding, however, that are the most consistent and studied aspects of power skating and a commonality that is seen with other forms of skating. The understanding of foot and lower extremity balance on top of a narrow balance point, the skate blade, will allow a practitioner to assist in both improved performance and overuse injury patterns.
In order to better understand the biomechanics of power skating and the clinical injury perspectives that may arise, it is first helpful to compare power skating with the more commonly understood biomechanics of walking. Both walking and skating are biphasic movement patterns that consist of periods of single- and double-limb support. By comparison, it is the support phase of walking that becomes the skating glide. One aspect of skating that makes it unique in the support phase is that the friction on the performance surface is much less than that seen in most walking activities. As a result there are decreased posterior linear shear forces with touchdown due to decreased friction and decreased anterior linear shear forces in the late midstance to propulsion stage. This low-friction surface will necessarily impart a need to abduct the foot by external hip rotation at propulsion [11]. The center of gravity therefore does not progress in a linear sinusoidal path over the foot as seen in walking , but rather the skater and his or her center of gravity move in an opposite direction to the weight-bearing skate.
The acceleration in power skating is divided into two unique stride patterns, the first three strides and the fourth stride, known as the typical skate cut [12].
The first stride pattern usually involves the first three strides. It lasts approximately 1.75 s, involves continual positive acceleration, and has a negligible or nonexistent glide phase [13]. It is during this stride pattern that the skater often appears to be “running” on his or her skates.
The second stride pattern often begins on the fourth stride and is considered the typical skate cut [12]. This stride pattern consists of periods of positive and negative acceleration and involves three phases. It starts with a glide during single-limb support which imparts negative acceleration [14]. It continues with propulsion during single-limb support which is accomplished by external rotation of the thigh and the initial extension movements of the hip and knee [15]. This stride pattern concludes with propulsion during double-limb support. During this phase the second limb acts as a balance point to complete propulsion through full knee extension, hyperextension of hip, and plantar flexion of the ankle.
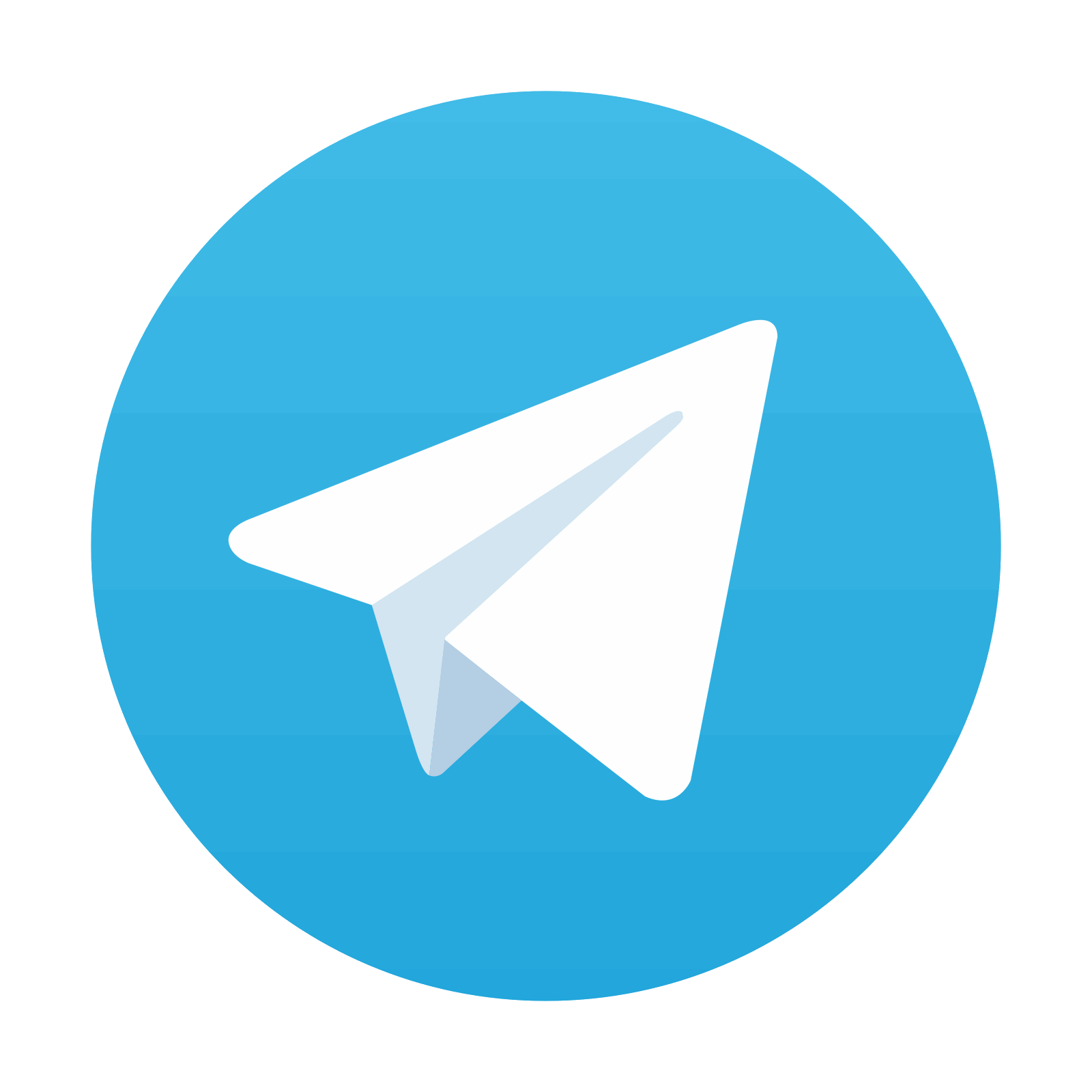
Stay updated, free articles. Join our Telegram channel

Full access? Get Clinical Tree
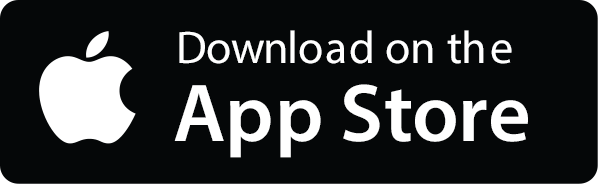
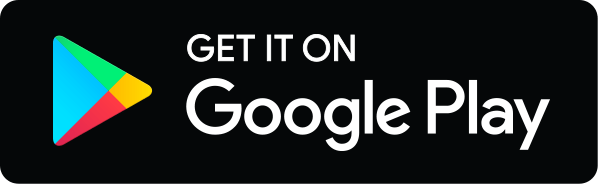