Fig. 7.1
(a) PASSPORT arthroscopic simulator showing the leg, a webcam that tracks instrument motions, and a user interface that provides selection of the exercises and offers real-time force and time feedback during training (© AJ Loeve 2012. Reprinted with permission). (b) Close-up showing the skin covering the joint, the instruments inserted in the joint, and the arthroscopic view (© AJ Loeve 2012. Reprinted with permission)
Escoto and coworkers also developed a high-fidelity knee joint bench model with performance tracking named Knee Arthroscopy Simulator [27]. The Knee Arthroscopy Simulator is composed of modular and replaceable plastic elements using quick release clamps that uncover the intra-articular joint space. The clamps also secure the skin watertight to allow joint irrigation. The lower leg is moveable and covered by a custom-made foam that holds hard plastic bones. Instrument motion is recorded by electromagnetic motion tracking system, and modified arthroscopic instruments that contain strain gauged allow the measurement of instrument tissue interactions. The Knee Arthroscopy Simulator shows the presence of a learning curve for trainees and was evaluated for face validity [27].
7.3 Virtual Reality Simulators
From the mid-1990s, computer scientists and (orthopedic) surgeons in various institutes teamed up to develop the first arthroscopic virtual reality simulators [11, 14, 16, 17, 28]. Virtual reality simulators comprise a computer and screen which present the virtual world including the intra-articular joint space, pathology, and instruments such as cameras, probes, punches, and shavers. For the computer scientists, the advantage of working in this medical area was the fact that bone is a solid tissue, which can be considered as an undeformable object in contrary to, for example, the soft tissues located in the abdominal area. Contrary, this also poses a huge engineering challenge as surgeons are used to haptic feedback during actual arthroscopy and demand this sensation as well in their arthroscopy simulators. In the early stages, the American Academy of Orthopaedic Surgeons (AAOS) evaluated virtual reality technology as a means of learning and maintaining surgical skills and felt that it was too early to commit the substantial resources required [8, 15]. But research groups continued development focusing primarily on the knee joint [4, 29–31] by application of volume rendering techniques [17], object deformation modeling techniques for collision detection [11, 20, 28], and computer graphics techniques to guide a trainer through exercises [29, 32, 33]. Also, Lu and coworkers have worked on algorithms that mimic tissue cutting [34].
The challenge of incorporating haptic feedback into the virtual environment has led to two different development strategies for virtual reality simulators. The first was started by Megali and coworkers who focused on the advantages of having a computer with specific software [29, 32]. The computer program offers unsupervised guidance for a trainee through different steps of a curriculum, which can be built of imaged-based instructions, videos, digital questionnaires, and basic navigation in a virtual environment. Assessment of performance is feasible using metrics that need to be defined anyway to build the interactive virtual environment, e.g., task time and path length. Within this strategy, specific basic skills that require high levels of haptic feedback (e.g., cutting) are less present or completely absent.
This strategy has been implemented in the SIMENDO ArthroscopyTM (Simendo, Rotterdam, The Netherlands, www.simendo.eu), a simulator that solely focuses on training of eye-hand coordination. The focus on eye-coordination training is prominently expressed in their exercises “4 Boxes” and “6 Boxes,” which take place in an entirely virtual world that does not represent a human joint and focuses solely on correct camera orientation (Fig. 7.2a). Target users are residents that have no arthroscopic experience. The simulator can also be connected to the Internet, where training progress is document and can be viewed by supervising surgeons.
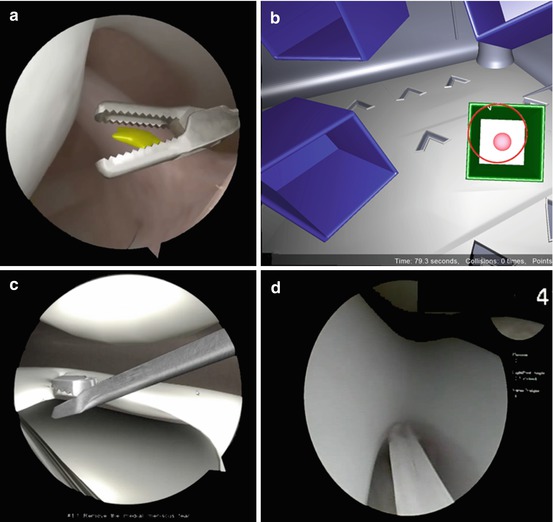
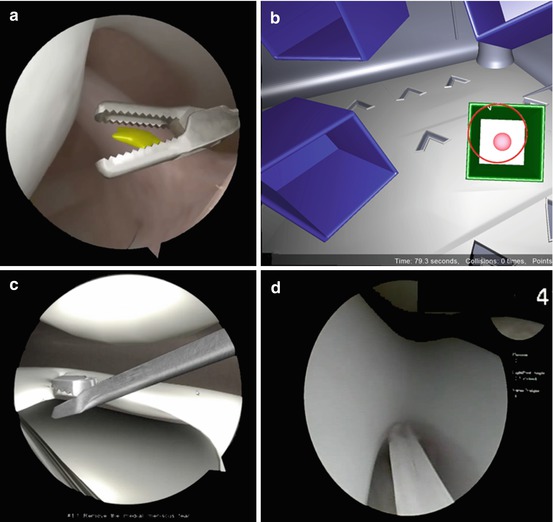
Fig. 7.2
(a) Screenshot of Virtamed ArthroS knee joint showing the ACL ligament and a grasper. (b) Screenshot from the virtual world of the Boxes exercises of the SIMENDO ArthroscopyTM. The ball in a box needs to be touched with the camera tip (© Simendo 2014. Reprinted with permission from www.simendo.eu). (c) Screenshot of ARTHRO MentorTM showing the lateral compartment and a punch. (d) Screenshot of ArthroSimTM showing the notch and the ACL of the virtual knee
Another initiative that has embraced this strategy is the joint project between the Royal College of Surgeons of England and Primal Pictures, which focuses on a “cognitive” online trainer for arthroscopy leaving out the “haptic” part. The concept of the “cognitive” trainer called VATMAS was built on earlier work on the VE-KATS simulator [20]. Initial results show that it is possible to reproduce arthroscopic optics and a probe that can be independently manipulated, with indication of contact with hard and soft surfaces. As laid out, such a simulated environment can take the trainee through a series of tutorials using real arthroscopic images or videos, while providing automated feedback. Hurmusiadis and coworkers in their study showed that this type of simulator is a simple and cheap interface to address the cognitive (“non-haptic”) components of arthroscopic knee surgery [35].
The second development strategy has been initiated by McCarthy and coworkers [6, 13], since they acknowledged that apart from realistic graphics, a key feature is the feeling of touch. It is for example important to know if the anatomic structure at the end of the probe is soft or hard. Their Sheffield Knee Arthroscopy Training System (SKATS) was initially designed as a cost-effective PC based knee arthroscopy simulator consisting of a hollow plastic leg, replica surgical instruments, and a monitor displaying the internal view of the knee joint [6, 13]. Evaluation of the SKATS simulator demonstrated severe acceptability issues due to the lack of realistic haptic feedback [36]. The SKATS simulator was updated by adding passive haptics in the form of a more realistic leg containing solid femur and tibia bones that gave resistance when navigating through the virtual environment [37]. The validation results obtained with this mixed reality environment provided construct, predictive, and face validity for navigation and triangulation training.
This strategy of combining virtual reality with passive haptic feedback using anatomic bench models or with active haptic devices has been applied in all other commercially available arthroscopic virtual reality simulators. These are shortly discussed. The ArthroSimTM Arthroscopy Simulator has been developed by the AAOS Virtual Reality Task Force in collaboration between the ABOS, the Arthroscopy Association of North America (AANA), and Touch of Life Technologies (ToLTech, Colorado, USA, www.toltech.net) (Fig. 7.2d). The ARTHRO MentorTM (Simbionix, Cleveland, Ohio USA, www.simbionix.com) and the Virtamed ArthroS (Virtamed AG, Zurich, Switzerland, www.virtamed.com) are the two other high-fidelity virtual reality simulators (Fig. 7.2b, c). They all offer a knee and a shoulder module, a wide variety of pathologies, and basic skills to be trained, including the identification of anatomical structures, navigation skills, triangulation and depth perception and instrument handling skills. Where ArthroSimTM solely focuses on diagnostics, the other two also offer training of therapeutic interventions such as screw placement, shaving, and cutting. All three types of simulators make optimal use of the computer graphics by offering video tutorials and 3D visualization of the instruments relative to the anatomic structures and performance assessment with objective metrics derived from the virtual environment. Individual studies indicate face and construct validity for the ArthroSimTM, ARTHRO MentorTM, and the Virtamed ArthroS [19, 38, 39].
7.4 Discussion and Future Perspective
The main conclusion is that currently simulators are available to train basic arthroscopic skills in the knee and shoulder joint in a highly standardized and repetitive manner. Simulators to train specific skills in other joints are virtually absent, although the “model with separate sensors”-concept can be easily applied in combination anatomic bench models of the wrist, hip, ankle, and elbow joint.
Training environments in which anatomic bench models are used allow for normal everyday life sensory feedback. This implies that the relevant human senses (vision and proprioception, and to a lesser extend sound and smell) can be used by the trainee to acquire feedback on their performance in a natural manner. A limitation is that the models are usually not sufficiently realistic for more complex skills training. Additionally, the combination with separate sensors often requires automated data processing software which is not readily available when purchasing the sensors. That is why anatomic bench models with sensors as of yet are not widely integrated in the training curricula. The work done by Tuijthof and Escoto and coworkers [10, 27] indicate that engineers are aware that improvements are needed. The challenge is to keep these models affordable, which is a big asset.
Virtual reality arthroscopy simulators offer highly realistic arthroscopic images, can be used repeatedly without consumable parts, and allow self-directed learning by practicing a large variety of different pathologies and exercises. Additionally, trainees can progress at their own pace and at a time of their choice without the need for a senior surgeon to be present [7]. Limitations of virtual reality are that they lack realistic tissue behavior [40], and they are expensive. A future perspective of the value of virtual reality simulators is that that they could be used for preparation of individual complex patient cases by loading their CT- or MRI- scans into the simulator. As to this date none of the simulators offers this option, but this approach can contribute to life-long learning strategies and involve more experienced surgeons in simulator training.
There is little published research on the current commercially available simulators and there has not been any evidence of the ability of simulator-based training to improve arthroscopic performance in the operating theatre [41–43]. One major drawback of all available simulators is that none allows repetitive training of portal placement, which has been indicated as one of the important skills residents should possess before they continue training in the operating room [9, 44].
Further crossover studies are needed with longitudinal follow up of trainees undergoing virtual reality simulation-based training to fully understand the benefits to patients [41]. It is accepted though that simulator training can shorten the time it takes for trainees to acquire basic skills in theatre and this has universal advantages, for trainees, trainers, institutions, and, most importantly, patients. Simulation-based training can cause a “right-shift” along the learning curve for more efficient training with real-world improvements [45–47]. In conclusion, for the training of basic skills, except portal placement, different types of simulators are available. The challenge is to implement this into training curricula.
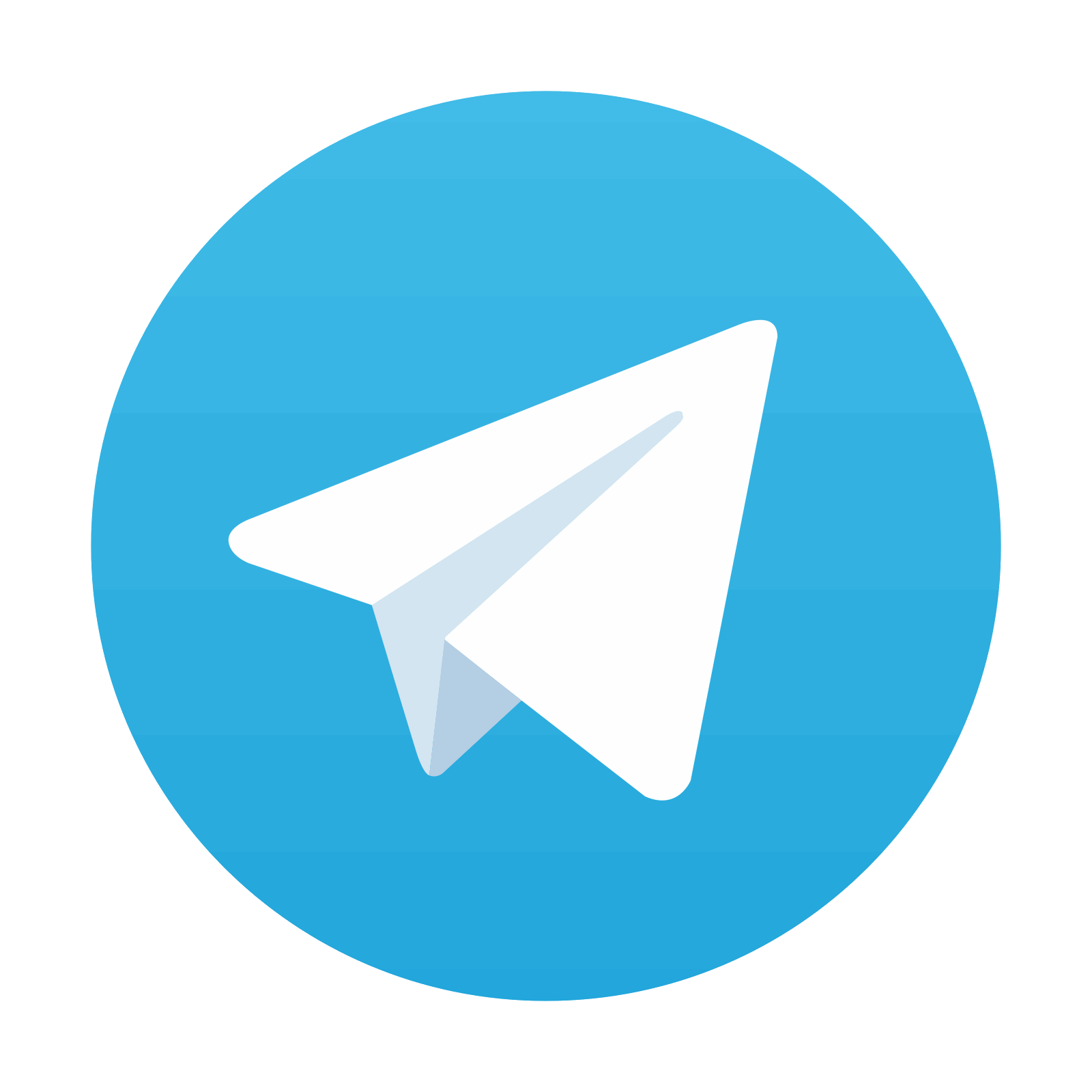
Stay updated, free articles. Join our Telegram channel

Full access? Get Clinical Tree
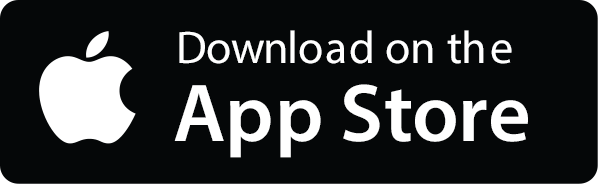
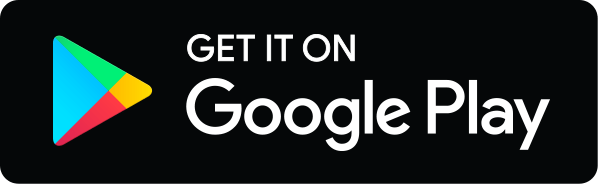