Sex and Gender Differences in Clinical and Preclinical Studies
Laura L. Tosi, MD
Austin P. Gillies, BA
Rolanda A. Willacy, BA
Barbara D. Boyan, PhD
Dr. Tosi or an immediate family member serves as a board member, owner, officer, or committee member of American Orthopaedic Association, Osteogenesis Imperfecta Foundation, and US Bone & Joint Initiative. Dr. Boyan or an immediate family member is a member of a speakers’ bureau or has made paid presentations on behalf of TitanSpine, Inc.; serves as a paid consultant to or is an employee of National Institutes of Health (NIAMS & NICHD), Spineology Inc., and Titan Spine, LLP; serves as an unpaid consultant to Institut Straumann AG, and Musculoskeletal Transplant Foundation; has stock or stock options held in SpherIngenics, and Titan Spine; has received research or institutional support from Institut Straumann AG, Musculoskeletal Transplant Foundation, and Titan Spine LLC; has received nonincome support (such as equipment or services), commercially derived honoraria, or other non-research-related funding (such as paid travel) from LifeNet, Musculoskeletal Transplant Foundation, and Titan Spine, LLP; and serves as a board member, owner, officer, or committee member of Commonwealth Center for Advanced Logistics Systems, Commonwealth Center for Advanced Manufacturing, Virginia Academy of Science, Engineering, and Medicine, and Virginia Bio. Neither of the following authors nor any immediate family member has received anything of value from or has stock or stock options held in a commercial company or institution related directly or indirectly to the subject of this chapter: Mr. Gillies and Ms. Willacy.
Keywords: bone loss; inflammation; musculoskeletal development; osteoarthritis; osteoporosis; peak bone mass; sarcopenia; sex differences; sexual dimorphism
INTRODUCTION
The US National Institutes of Health (NIH) define “sex” as biological differences between females and males, including chromosomes, sex organs, and hormonal profile. “Gender” is defined as socially constructed as well as enacted roles and behaviors within a historical and cultural context.1 Musculoskeletal health is one of the areas of medicine in which differences between men and women—in morphological, physiological, and behavioral characteristics—are most evident. Although there is a higher incidence of acute traumatic injuries among men, women tend to be disproportionately more disabled by musculoskeletal conditions. Examples of conditions leading to disability with higher incidence in the female population, as compared with their male counterpart, include anterior cruciate ligament (ACL) injuries and spinal deformities during adolescence, stress fractures during adulthood, osteoarthritis, rotator cuff tears, reduced bone density, and osteoporotic fragility fractures. However, the study of the impact of sex on these differences has mostly focused on epidemiological aspects, and the biological basis of sexual dimorphism has only recently begun to be elucidated.2
For most of the last century, researchers believed that in higher vertebrates genetic sex determination dictated the sex of the gonads and that hormonal secretions produced by the gonads then determined the sexual phenotype of the rest of the body as well as behavior. Over the past two decades, genome-wide analyses of gene expression have provided a much better understanding of the genes located on the sex chromosomes, their evolution, and their
function. This research has not only confirmed that there are extensive phenotypic differences between the sexes, but has also revealed that there is an equally extensive amount of divergence in gene expression. Moreover, the inherent inequality of the amount of genetic material in the X and Y chromosomes in the two sexes has effects throughout the body, not just on the gonads. Thus, although males and females share a common genome, genes that differ in expression between the sexes, or sex-biased genes, lead to variation in phenotypic traits.3
function. This research has not only confirmed that there are extensive phenotypic differences between the sexes, but has also revealed that there is an equally extensive amount of divergence in gene expression. Moreover, the inherent inequality of the amount of genetic material in the X and Y chromosomes in the two sexes has effects throughout the body, not just on the gonads. Thus, although males and females share a common genome, genes that differ in expression between the sexes, or sex-biased genes, lead to variation in phenotypic traits.3
Despite these discoveries, progress in the recognition of sex differences has been slow. Before the 1990s, biomedical research was centered on the male model, and this belief was grounded in the ideology that male biology (excluding the reproductive system) was representative of the species. It was not until 1985 that the US Public Health Service noted that the lack of knowledge of female biology compromised the health of women. And it was not until 1993 that Congress passed the NIH Revitalization Act mandating that women and minorities be included in all clinical research. Additionally, this Act required that data from phase III clinical trials be analyzed by sex.
A major step forward was taken in 2001 when the Institute of Medicine Committee on Understanding the Biology of Sex and Gender Differences published the report titled “Exploring the biological contributions to human health: Does sex matter?”, which stated that “every cell has a sex” and that research should account for sex differences from “womb to tomb.”4 Researchers then began to realize that every organ in the body, not just in the reproductive system, responds differently on the basis of sex due to chromosomal differences and to sex-specific hormonal regulation. A male cell is not the same as a female cell, and sex chromosome-linked genes can be expressed in the somatic cells as well as germline. However, as noted above, it was primarily the explosion of genomic data that has underscored the importance of this report.
In 2014, the NIH issued a mandate stating that all funded preclinical studies had to include both females and males (animals, tissues, or cells).5 The rationale behind this mandate was the idea that the failure to consider sex and sex-related differences in cellular biology and pathology played a role in the lack of reproducibility in biomedical studies and in the literature.
Two years later, an initiative implemented by the NIH included sex as a biological variable (SABV).1 This policy was designed to improve the reproducibility of studies by requiring that sex be factored into the design, analysis, and reporting of human and vertebrate animal research. It was also designed to increase the attention that was given to the role of sex in cell physiology, biologic function, and disease symptoms and manifestations.
Since the issuance of these recommendations and guidelines, the inclusion of “sex” in research analyses has led to groundbreaking discoveries in areas such as medication dosing and also to a greater understanding of diseases such as multiple sclerosis (MS), Parkinson disease, schizophrenia, and stroke, underscoring the importance of sex as a biological variable.1 The inclusion of “gender” is far more nuanced. In the past, many authors have used the term incorrectly, likely because of their discomfort in using the term “sex.” However, this is no longer acceptable and, as more individuals begin to acknowledge that they have chosen to assume a gender role that is different from the sex they were assigned at birth, it is clear that additional research is needed to ensure that their health needs are also met.
This chapter will explore the wide heterogeneity of musculoskeletal growth, development, and pathological traits due to sex differences and the opportunities to improve healthcare through recognition of sex as a biological variable.
SEXUAL DIMORPHISM IN THE DEVELOPMENT OF THE MUSCULOSKELETAL SYSTEM
Men and women have fundamentally different strategies for building mechanically functional bone. Jepsen and colleagues have demonstrated that, on average, women build more slender bones (narrow relative to length) for their body size, with less cortical area and only slightly more cortical bone mineral density (BMD), compared with the more robust bones of men.6 Differences in the way these traits covary with sex is consistent for both Caucasian and African-American cohorts. As discussed below, female femurs are not just a more slender version of male femurs; their differences have far-reaching clinical implications for developing sex-specific diagnostic and treatment strategies to reduce fragility fractures.
Bone structural integrity is a function of a multitude of complex and interrelated traits of the bone matrix, microstructure, macroscopic bone morphology, and bone density.7 Research data support the concept that bone traits coadapt to functional loading in a complex multivariate manner and that these complex traits differ between the sexes and vary with age. In both sexes, elegant coadaptation for a myriad of traits—at all of bone’s hierarchical levels of organization—functions to maintain adequate biomechanical properties in the face of ever-changing, and sometimes competing, physiological and mechanical demands. Evidence of sex-specific genetic effects on a number of fragility-related traits, taken together with physiological differences between men and women throughout life, indicate that important differences exist in the genetic regulation of bone formation. Historically, male mice have been used to study these traits, but Jepsen et al. have called this practice into question as females were shown to build stronger and more robust bones than their male counterparts in a study of inbred mice.6 This also raises the issue of whether murine models are appropriate for modeling human sexual dimorphism in the musculoskeletal system.
SEXUAL DIMORPHISM IN THE DEVELOPMENT OF PEAK BONE MASS
Peak bone mass (PBM), the maximum amount of bone accrued at the end of the growth period, is an important predictor of future risk of osteoporosis and fracture. Phenotypic sex differences are found in bone diameter and cortical index (a ratio of cortical thickness over bone diameter) at a very early age and continue throughout most of life. Sexual dimorphism in summed cortical thickness and bone length, however, is not evident until shortly after the pubertal growth spurt. The greatest gain in bone mass occurs during the rapid bone growth period associated with puberty8 (Figure 1). During skeletal growth, males display increased periosteal apposition, bone diameter, and cortical thickness. However, females at puberty display inhibited periosteal apposition and increased cortical thickness through the formation of endosteal bone formation, which decreases the endosteal perimeter9 (Figure 2).
Lifestyle choices affect 20% to 40% of adult PBM.10 However, genomic investigations of BMD in young individuals (ie, prior to attaining PBM) indicate that between a third and a half of the variations in bone growth and mineral accretion are influenced by common genetic variants. Liu et al failed to find a gene by sex interaction effect on BMD in adults11; however, a recent genome-wide association study (GWAS) in children participating in the Bone Mineral Density in Childhood Study (BMDCS) found two unexpected results: sex specificity of two loci and large effect size.12 Moreover, the signals identified in this study do not reside on the X chromosome, adding to the mounting evidence for autosomal sex-specific effects.
This study is particularly interesting because the effect sizes reported for the GWAS associations were higher (sometimes
by an order of magnitude) than those reported in larger adult meta-analyses.13 The authors noted that while this could be an effect of the “winner’s curse” or Beavis effect (statistical terms that refer to the possible overestimation of effect size of a quantitative trait locus as the result of small sample size), it is plausible that genetic variation exerts a stronger influence in children, in whom cumulative exposure to environmental factors is lower than in adults.14 They suggested that this age-specificity may explain why they could not find strong evidence of replication for many of the pediatric signals in the publicly available datasets for BMD in adults (which also are not stratified by sex).
by an order of magnitude) than those reported in larger adult meta-analyses.13 The authors noted that while this could be an effect of the “winner’s curse” or Beavis effect (statistical terms that refer to the possible overestimation of effect size of a quantitative trait locus as the result of small sample size), it is plausible that genetic variation exerts a stronger influence in children, in whom cumulative exposure to environmental factors is lower than in adults.14 They suggested that this age-specificity may explain why they could not find strong evidence of replication for many of the pediatric signals in the publicly available datasets for BMD in adults (which also are not stratified by sex).
EXERCISE
Numerous articles have sought to analyze lifestyle factors that might optimize PBM and strength and reduce the risk of osteoporosis or low bone mass later in life. Perhaps the greatest interest has been in the area of exercise. During periods of growth, increased body weight and muscle strength and longitudinal bone growth lead to increased loads placed on the skeletal structure. As a result of the high levels of bone modeling and remodeling that occur, it has been suggested that these periods of growth are ideal to positively affect bone mass. Observational studies have reported higher areal bone mineral content (aBMC) and areal bone mineral density (aBMD) in physically active children compared with sedentary children.15
In the early 1990s, observational studies began to report higher bone density among physically active children. Unfortunately, subsequent randomized trials were less convincing than observational studies. There are several explanations for this, and they are typically not consistent among studies. Results from observational studies, in which children or young adults with high activity levels were likely to have been more active throughout their lifetime, indicate that increased bone density may have been a reflection of cumulative effects of activity on bone, whereas in randomized trials exercise is for shorter time periods. Inconsistencies among exercise trials may be explained in part by sex and maturity differences in the bone response to loading.
Unfortunately, the majority of trials do not report results by sex and have either been completed in single sex groups or have combined both sexes in the analyses. However, a recent report by McVeigh et al underscores the influence of organized sports activity on PBM as well as highlights the challenge that fewer females are likely to achieve “consistent sport participation.”16 Future studies need to report separate results for women and men, and the data need to be analyzed in the context of estrogen-driven bone packing in females during puberty and extending into the reproductive years, as well as bone responsiveness.
HORMONES AND PEAK BONE MASS
Estrogens and androgens act as promoters of accrual of bone mass during puberty, and low levels of these hormones play a role in the initiation of the pubertal growth spurt. Sex hormones indirectly regulate this period of skeletal growth via growth hormone (GH) and insulinlike growth factor I (IGF-I).17 The result of differential initiation of puberty in boys in girls can be seen as a greater length of time for prepubertal appendicular growth in boys, which contributes to height differences between sexes. In girls, elevated levels of estrogen limit the expansion of periosteal bone, but stimulate endocortical
apposition. During puberty, boys experience significant effects of both estrogens and androgens, which stimulate periosteal bone expansion and higher rates of cortical bone growth.18 At the end of puberty, estrogen contributes to the closing of epiphyseal plates, slowing longitudinal growth. Thus, there is a differential rate in longitudinal bone growth between the sexes. These hormonal changes can be observed as complex multivariate interactions between the hormone milieu found in the bone environment and mechanical loading.
apposition. During puberty, boys experience significant effects of both estrogens and androgens, which stimulate periosteal bone expansion and higher rates of cortical bone growth.18 At the end of puberty, estrogen contributes to the closing of epiphyseal plates, slowing longitudinal growth. Thus, there is a differential rate in longitudinal bone growth between the sexes. These hormonal changes can be observed as complex multivariate interactions between the hormone milieu found in the bone environment and mechanical loading.
Estrogen is produced by the action of aromatase on testosterone.19 Therefore, the pathways for synthesis of estrogen and androgens are closely related to each other, which causes some difficulty when attempting to study the individual impact of hormones on bone homeostasis. The role of estrogen receptors (ERs) and androgen receptors (ARs) in the response of cells to these hormones has been widely studied through the use of animal models with the use of germline or tissue-specific knockouts. Although these models do not fully explain the phenotypes of sex hormone deficiency, much has been learned concerning their mechanism of action. In females, increased estrogen serum levels at puberty have a number of effects on bone cell activity. Estrogen acts via estrogen receptor alpha (ERα) to inhibit periosteal apposition, endosteal bone resorption, and chondrocyte remodeling, which result in epiphyseal closure and attenuated periosteal expansion. In contrast, in males at puberty, estrogen stimulates periosteal expansion via ERα and androgen acts via the androgen receptor (AR) to increase trabecular bone formation.18
Sex hormones bind to nuclear hormone receptors to act upon target cells. In addition, many steroid hormones, including estrogen can act on target cells via membrane-associated receptors. Compared with the sex steroid receptors ER and AR, less is known about the role of progesterone and the progesterone receptor (PR) in bone cell metabolism. Similar to the action of estrogen and testosterone, the biological effects of progesterone are mediated by interaction with membrane-associated receptors as well as intracellular receptors. Selective inhibition of PR signaling in mesenchymal stem cells (MSCs) and particularly in osteoprogenitor cells but not mature osteoblasts, significantly affects pathways involving immunomodulation, bone remodeling, extracellular signaling, and matrix interactions.19 Most importantly, these effects are only seen in males. These findings suggest that PR signaling in MSCs may intrinsically regulate sex differences in health and disease conditions.
The pubertal period is routinely the most active time for bone changes, and the size, shape, and mass of long bones are arguably the most outwardly identifiable phenotypic sex differences in the skeleton. Some of these differences can be seen as early as two years of age. By the age of two years, boys already show significantly greater bone diameter than girls, and girls possess a concomitantly greater proportion of cortical bone than boys.8 These differences are magnified during puberty, when the pubertal hormone surge elicits different responses from the growing bones in girls and boys. Both boys and girls are experiencing an earlier onset of puberty and a connected acceleration in bone maturation than several decades ago.
Using data from the FELS Study, the world’s largest and longest running study of human and growth development, Duren et al. have recently demonstrated sex-specific secular changes in skeletal maturation, and that individual processes underlying the bone age phenotype may be differentially affected by this secular trend.20 Specifically they found that over the past century, the age at epiphyseal fusion initiation and the age at epiphyseal fusion completion have gradually decreased by as much as 6.7 and 6.8 months in males and 9.8 and 9.7 months in females, respectively.21 This is important for clinical management, including treatment timing, of orthopaedic patients, because children today exhibit a different pattern of maturation than children on whom original maturity assessments were based, including both the FELS Method or the Greulich-Pyle Atlas.22
INFLUENCE OF SEXUAL DIMORPHISM ON THE DEVELOPMENT OF MUSCULOSKELETAL CONDITIONS DURING CHILDHOOD, ADOLESCENCE, AND YOUNG ADULTHOOD
PREMATURE OSTEOARTHRITIS
Femoroacetabular impingement (FAI) and developmental dysplasia of the hip (DDH) are the leading causes of premature osteoarthritis of the hip joint. Females are uniquely predisposed to DDH, and there is frequently a history of left-sided disease and breech presentation. In DDH, a shallow acetabulum causes an increase in mechanical stress on the cartilage matrix, with failure of the acetabular labrum that progresses to degeneration and osteoarthritis.
In the nondysplastic hip, repetitive abnormal contact of the femoral head-neck junction and the anterior rim of the acetabular labrum during dynamic hip motion results in damage to both the joint cartilage and the labrum. The morphology and pattern of disease is very distinct and results in variable patterns of joint injury. Asphericity of the head and loss of offset at the head-neck junction, combined with focal or global overcoverage of the acetabulum, results in repetitive collisions and microtrauma to the chondrolabral complex with terminal hip range of motion.
Even though these abnormalities affect both genders, FAI is more common in males and more likely to be bilateral. In addition, several authors have noted that the hip morphology is more similar between the two sides in this non-DDH group. This suggests that adolescent dysplasia may be caused by malformation of the entire pelvis, which is different from infant DDH. The etiology of FAI remains undefined, but sex- and sport-specific differences in prevalence and morphology suggest adaptive changes in the immature musculoskeletal system in response to mechanical stresses. In addition, recent studies
have demonstrated that there are demographic differences between patients diagnosed with hip dysplasia in infancy versus adolescence/adulthood, and there is a growing consensus that these represent distinct forms of dysplasia. In both, there is a familial tendency toward hip disease with a higher incidence of arthroplasty in the adolescence/adulthood dysplasia group’s family members and higher frequency of infantile dysplasia in the DDH group’s family members.23
have demonstrated that there are demographic differences between patients diagnosed with hip dysplasia in infancy versus adolescence/adulthood, and there is a growing consensus that these represent distinct forms of dysplasia. In both, there is a familial tendency toward hip disease with a higher incidence of arthroplasty in the adolescence/adulthood dysplasia group’s family members and higher frequency of infantile dysplasia in the DDH group’s family members.23
ANTERIOR CRUCIATE LIGAMENT INJURIES
ACL injuries of the knee are common in women, with an incidence of two to eight times higher than in men, in sports such as basketball, soccer, and handball. Women more commonly exhibit an anteriorly rotated pelvis, contralateral pelvic drop, internal hip rotation and adduction, knee valgus, tibia external rotation, and foot pronation. The sex-based bony architecture differences in osseous morphology such as plateau dimensions and femoral condyle geometry, hip version, and length of the femur, as compared with the pelvic width, notch width index (NWI), and tibial slope, have all been associated with increased risk of functional knee instability and ACL injury. However, no causal relationship has been proven to this point. Recent studies have investigated proximal and distal anatomical differences in the kinetic chain, including restricted hip range of motion and its impact on the compensatory stresses and risk of ACL injury. The recognition of variable anatomy and, perhaps more importantly, the understanding of the etiology of these differences is of paramount importance in injury prevention and rehabilitation.24 Females land with a more upright and erect posture than males, and this subjects the knees to forces that lead to anterior tibial translation. Also in females, greater external rotation may predispose them to increased rates of ACL injury because of increased laxity of the knee. Differences have also been reported in the functional adaptation of females to sports biomechanics notably, jumping and landing, which may be learned behaviors.
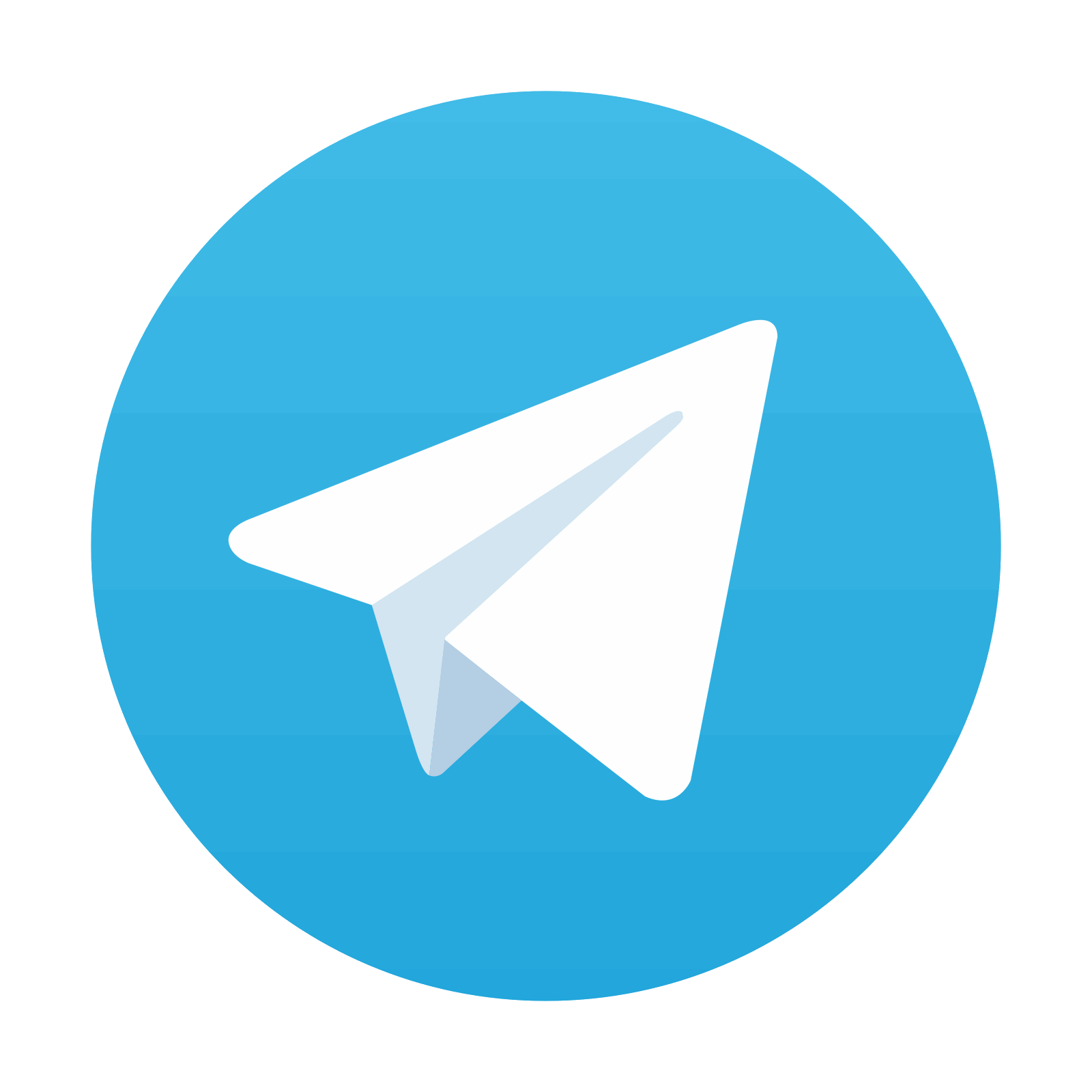
Stay updated, free articles. Join our Telegram channel

Full access? Get Clinical Tree
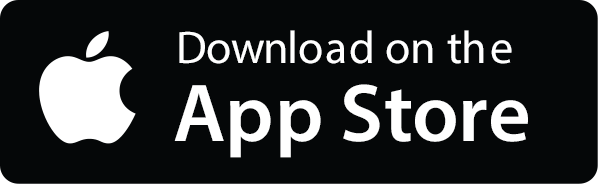
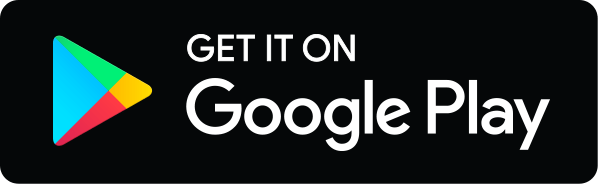