Structure and Physiology of Peripheral Nerves
Emily A. Van Kouwenberg, MD
Michael Hausman, MD
Dr. Hausman or an immediate family member is a member of a speakers’ bureau or has made paid presentations on behalf of Trimed; serves as an unpaid consultant to Skeletal Dynamics; and has stock or stock options held in Checkpoint SurgicalNDI MedicalSPR Therapeutics Neither Dr. Van Kouwenberg nor any immediate family member has received anything of value from or has stock or stock options held in a commercial company or institution related directly or indirectly to the subject of this chapter.
Keywords: anatomy; peripheral nerve; physiology; structure
INTRODUCTION
Peripheral nerves comprise afferent and efferent pathways and autonomic control fibers. A complex connective tissue lattice is responsible for protecting the nerve cells and maintaining homeostasis that is crucial to normal nerve function. Peripheral nerve dysfunction can result from degenerative conditions, nerve compression, or extremity trauma. The degree of nerve recovery after these insults will determine the ultimate functionality of the affected limbs. Understanding the macroscopic structure, microscopic structure, and physiology of peripheral nerves is necessary to understand the effects of nerve injury.
MACROSCOPIC STRUCTURE OF PERIPHERAL NERVES
The central nervous system includes the brain and spinal cord, while the peripheral nervous system is composed of peripheral nerves and their spinal nerve derivatives, cranial nerves, and the peripheral autonomic nervous system. Peripheral nerves consist of motor, sensory, and postganglionic autonomic nerve fibers that serve to communicate between the spinal cord and the rest of the body. Motor axons that exit the ventral horn and sensory axons that enter the dorsal horn of the spinal cord distally form the ventral and dorsal rootlets, respectively; these combine to form mixed spinal nerves as they pass through the spinal foramen. Spinal nerves then split into dorsal and ventral rami, which contribute to plexuses and peripheral nerves that supply different areas of the body (Figure 1). For example, the ventral rami of C5 through T1 form the brachial plexus, while the dorsal rami at these levels supply the posterior neck and trunk. The motor neuron cell bodies of peripheral nerves are located in the ventral horn of the spinal cord, and the sensory neuron cell bodies are located in the dorsal root ganglia just outside of the spinal cord in the intervertebral foramina. Understanding the distinct locations of motor versus sensory cell bodies is critical to accurately diagnose and localize conditions such as a brachial plexus injury. For example, nerve avulsion from the spinal cord constitutes a postganglionic injury to the motor nerve fibers, but a preganglionic injury to the sensory nerve fibers since the connections between the sensory cell bodies in the dorsal root ganglion and the axons are not severed.
Peripheral nerves are composed of organized groups of nerve fascicles that form nerve branches to innervate end organs. Fascicles within a nerve are larger and fewer in number in more proximal locations and smaller and greater in number distally. In the proximal extremity, the nerve fibers that supply the proximal limb tend to be more superficially located within the nerve to facilitate branching to their target, compared with more distally directed fibers that are distributed over multiple fascicles.1,2 As the nerve reorganizes distally, nerve fibers with the same distal target join the same fascicles. Fascicles become superficially positioned before branching, and terminal branches are often monofascicular.2 Distally, fascicles are
cable-like and can be followed proximally for a considerable distance with consistent somatic organization. Eventually, fascicles will begin to intermingle with other fascicles proximally and will become less discrete3. The ability to follow well-defined fascicles for a substantial distance proximally is the basis for nerve transfer surgery. Historically, it was thought that fascicles frequently redistribute by branching and uniting with other fascicle branches, resulting in a constantly changing plexiform architecture.2,4 Sunderland believed that any given fascicular pattern would not persist for more than 1.5 cm.5 More recent studies, however, have shown that although nerve fascicles intermingle proximally, there is far less intermingling than previously reported by Sunderland. These more recent works have also shown that nerve fibers that innervate specific end organs typically remain together along much of the nerve course, even throughout fascicular rearrangements proximally3,6,7,8.
cable-like and can be followed proximally for a considerable distance with consistent somatic organization. Eventually, fascicles will begin to intermingle with other fascicles proximally and will become less discrete3. The ability to follow well-defined fascicles for a substantial distance proximally is the basis for nerve transfer surgery. Historically, it was thought that fascicles frequently redistribute by branching and uniting with other fascicle branches, resulting in a constantly changing plexiform architecture.2,4 Sunderland believed that any given fascicular pattern would not persist for more than 1.5 cm.5 More recent studies, however, have shown that although nerve fascicles intermingle proximally, there is far less intermingling than previously reported by Sunderland. These more recent works have also shown that nerve fibers that innervate specific end organs typically remain together along much of the nerve course, even throughout fascicular rearrangements proximally3,6,7,8.
Although major peripheral nerves are generally well protected, there are anatomic sites where they are vulnerable to injury, including sites where they pass through narrow channels, run across bony structures, or travel at sharp angles. Compressive and tensile forces sustained in various positions and with various motions can impact blood flow to the nerve, axonal transport, and conduction of action potentials. Biomechanical properties of peripheral nerves allow them to tolerate these forces to an extent.9 With increased tensile stress during range of motion, nerves are able to lengthen as a result of viscoelastic properties. Nerves are also able to glide during excursion toward the site of elongation to relieve some of the tension.9 With normal range of motion, the brachial plexus has a total excursion of about 50 mm with shoulder motion. The median and ulnar nerves have about 7.3 and 9.8 mm of excursion, respectively, at the elbow and 14.5 and 13.8 mm of excursion, respectively, at the wrist.1,10 Mobility is more restricted at sites of nerve branching and vessel penetration.11
MICROSCOPIC STRUCTURE OF PERIPHERAL NERVES
The neuron is the smallest functional unit of nerves and is composed of a cell body with cytoplasmic extensions, including multiple dendritic processes to receive information (afferent) and a single axonal process to transmit information (efferent)12 (Figure 2). Inside of the cell body, otherwise known as the soma, is the nucleus, neurofilaments, mitochondria, lysosomes, and Nissl bodies surrounded by cytoplasm.12,13 The cell bodies of peripheral nerves are sometimes located within the central nervous system.14 The axon originates from the soma at a site called the axon hillock and travels distally as a peripheral extension.1,11 Each axon has a thin outer layer called the axolemma, which is the cell membrane that encloses the axoplasm and extends from the cell body along the entire length of the axon.11 The axoplasm acts as a conduit for transport of substances from the cell body to the distal nerve terminals, and vice versa.11 Within the axon there are many structures supporting its function, including mitochondria, endoplasmic reticulum, lysosomes, vesicles, microtubules, and neurofilaments11 (Figure 3). Schwann cells wrap around the axons and lay down myelin along the axolemma.11
Axons with their associated Schwann cells form nerve fibers. Myelinated nerve fibers are composed of a series of adjacent Schwann cells that surround and wrap around a single axon, covering it with multiple compact layers of plasma membrane to form a myelin sheath.9,15 The segments of axon covered by Schwann cells and myelin are called internodes, whereas the small segments of axon between Schwann cells are called nodes of Ranvier9,11 (Figures 4 and 5). Nodes of Ranvier are about 1 µm in length and occur about every 1 mm along the length of the axon.4 Axons can form branches at the nodes of Ranvier, allowing a single cell body to innervate multiple end organs.14 The myelin sheath extends along the length of the nerve fiber, beginning just after the axonal hillock and terminating 1 to 2 µm from the end of the axon.11 Unmyelinated nerve fibers contain several axons that are surrounded as a group by a single layer of Schwann cells, without the formation of multiple, compacted plasma membrane layers seen in myelinated nerve fibers9,15 (Figure 6). In both myelinated and unmyelinated nerve fibers, Schwann cells are surrounded by an associated basal lamina.4 Myelinated nerve fibers tend to be larger than unmyelinated nerve fibers, with myelin typically found in those greater than 2 µm and absent in those smaller than 2 µm; myelinated nerve fibers usually range 2 to 14 µm while unmyelinated fibers range 0.2 to 3 µm in size.9,11,16
Most peripheral nerves contain both myelinated and unmyelinated nerve fibers.3,14 Nerve fibers can be classified by their size and function. The largest myelinated nerve fibers are classified as group A fibers and have the fastest conduction velocity. Myelinated autonomic and preganglionic fibers are group B fibers, and thin nonmyelinated fibers are group C fibers.1,13
Nerves comprise many nerve fibers arranged and held into groups by connective tissues, including the endoneurium, perineurium, and epineurium (Figures 7 and 8). These connective tissues provide nerves with protection, framework, and compartmentalization to maintain specialized environments.4,15 The connective tissue surrounding each individual nerve fiber is called the endoneurium, which encases longitudinally oriented type I and type II collagen fibers, fluid, and cells such as fibroblasts, macrophages, and mast cells along with the nerve fiber.9 In larger nerve fibers, the endoneurium can be divided into two layers, including a thin inner layer that covers Schwann cells and comes into close contact with the nodes of Ranvier and an outer layer that does not approach the nodes of Ranvier.11
Nerve fibers are bundled into fascicles, which are bound by a relatively thin but dense connective tissue sheath called the perineurium. This sheath includes up to 15 concentric layers of flattened polygonal cells surrounded by basal laminas, with collagen and elastic fibers arranged in varying orientations between the perineurial cells. The perineurium can be subdivided into internal, intermediate, and external zones.4,9,14 The internal zone is typically comprised of a single layer of tightly interconnected perineurial cells called the perilemma, while the intermediate and external zones are comprised of multiple cell layers.9 The tight cellular junctions of the internal zone contribute to the blood-nerve barrier, resulting in diffusion properties that preserve intrafascicular pressures and protect the nerve fibers against infection.3,11,17 The external zone is not easily distinguished from the epineurium and contains type I and type II collagen fibers and elastic fibers arranged in longitudinal, oblique, and circumferential orientations between the cell layers; these fibers contribute to the biomechanical properties of the perineurium.9,14 Collagen in the perineurium has a wavy arrangement similar to the epineurium, but a relatively smaller period of the waves (6 vs 39 µm), possibly due to smaller fibril diameter (60 vs 80 nm).4,18,19 The perineurium functions to provide tensile strength to the nerve and is thicker at sites more vulnerable to strain, such as across joints or at nerve bifurcations.9 The perineurium is under a degree of longitudinal tension at baseline, which is why nerve ends retract after transection.4,20 As fascicles reach terminal nerve branches, the surrounding perineurium transitions into a single layer that is continuous with muscle spindle and end organ capsules.11
Fascicles are held together to form a nerve trunk by epineurium, which is the outermost layer of connective tissue. While the perineurium provides tensile strength and elasticity to tolerate stretch, the epineurium acts as a shock-absorber to provide protection from external compression.2 The epineurium is formed by longitudinally oriented bundles of type I and type III collagen fibers, elastic fibers, and adipose tissue, and contains abundant fibroblasts and scattered mast cells.9,11 The adipose tissue helps to protect the nerve from compression and is often reduced in diabetics; this potentially explains the increased vulnerability to compression injuries seen in diabetic patients.4 The fibroblasts within the epineurium are responsible for fibrosis after nerve injury, which can limit mobility and thereby increase the risk for additional injury by stretch or compression forces.4 The epineurium can be divided into epifascicular and interfascicular components. The epifascicular epineurium is the outer nerve sheath and is surrounded by a layer of loose areolar tissue called the mesoneurium, which loosely attaches to adjacent soft tissues and allows movement of peripheral nerves along their courses.3,11,13,15,18 This tissue is
sometimes confused with adipose tissue, which has led to the popular misconception that fat is a good environment for a damaged nerve. In actuality, fat can scar to the epineurium, and the mesoneurium is more complex with a tenosynovial-like gliding property that is lacking in fat. The interfascicular epineurium allows gliding between the fascicles and contains collagen fibers in wavy arrangements to accommodate stretch.9,15,18 Nerves composed of many smaller fascicles tend to have more total epineurium than those composed of larger and fewer fascicles and are therefore better protected from compression forces.2,17 The epineurium tends to be proportionally thicker in larger nerves and where nerves cross joints.4,13
sometimes confused with adipose tissue, which has led to the popular misconception that fat is a good environment for a damaged nerve. In actuality, fat can scar to the epineurium, and the mesoneurium is more complex with a tenosynovial-like gliding property that is lacking in fat. The interfascicular epineurium allows gliding between the fascicles and contains collagen fibers in wavy arrangements to accommodate stretch.9,15,18 Nerves composed of many smaller fascicles tend to have more total epineurium than those composed of larger and fewer fascicles and are therefore better protected from compression forces.2,17 The epineurium tends to be proportionally thicker in larger nerves and where nerves cross joints.4,13
The connective tissue layers of peripheral nerves are continuous with the central nervous system meninges: the epineurium is continuous with the dura mater, the outer perineurium with the arachnoid mater, and the inner perineurium with the pia mater.9,17 The endoneurial space is continuous with the subarachnoid space, with communication between the endoneurial fluid and cerebrospinal fluid.9 Nerve roots lack both epineurium and perineurium and have a less substantial endoneurium, resulting in greater susceptibility to injury from compression or traction.2 Understanding this regional variation of structure along the course of the nerve can help the surgeon make an accurate assessment of the degree of damage and, hence, the prognosis for recovery.
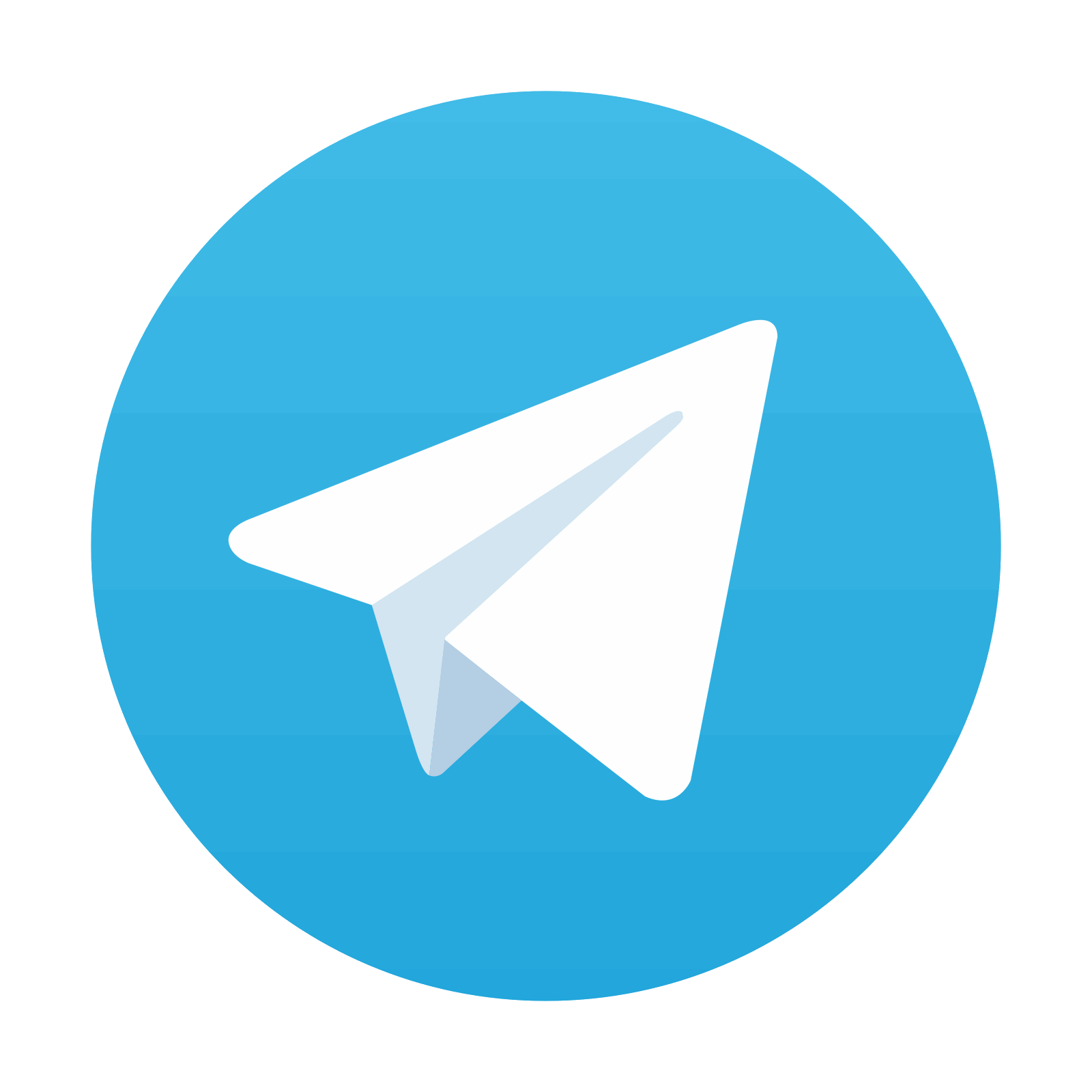
Stay updated, free articles. Join our Telegram channel

Full access? Get Clinical Tree
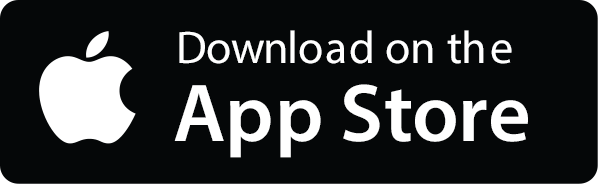
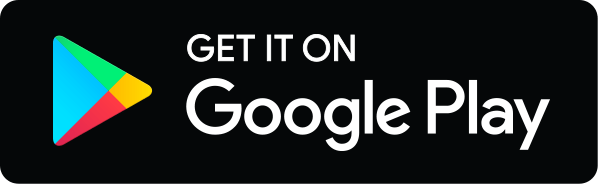