4.4 Pediatric fractures
To access the videos, please follow the URL link
1 Introduction and epidemiology
Injuries of the musculoskeletal system are common in children and usually related to the normal activities of childhood, including play and sports. Musculoskeletal injuries are the second most common cause (after head injuries) of permanent disability in childhood. One in seven children seen in the emergency department presents with a fracture. We must be aware that young children are sustaining more severe injuries because of new, high-velocity sports and sport equipment (eg, rollerblades, skateboards, high-tech bicycles). Mann and Rajimaira [1] reviewed 2,650 long-bone fractures in children, 30% of which involved the physis. Up to 50% of physeal injuries occur in the distal radius. The second most commonly injured area is the distal humerus, of which about 50% of fractures are treated operatively [2]. The overall incidence of fractures depends greatly on the activities of a child. Children participating in sports are more likely to sustain diaphyseal fractures and injuries to the physis.
All orthopedic, trauma, and pediatric surgeons must have a basic knowledge of children′s fractures.
A child is not just a small adult. The main differences lie in the physical properties of the skeleton and its ability to grow.
The aim in this age group must be to provide, from the beginning, treatment that is effective, definitive, and appropriate to childhood injury. Repeated reduction maneuvers and anesthesia are to be avoided. Children, after injury, should be able to return to full activity without undue pain or long-term problems.
2 Growth and development
Immature bone is resistant to mechanical forces and can respond quickly to injury. Provided the essential zones of the growth plate are not directly involved, growth of the immature skeleton has a positive influence on fracture repair. Two different regulatory systems exist for growth:
Longitudinal growth (length) is regulated by the epiphyseal system.
Circumferential growth (diameter and thickness) is regulated by the periosteal/endosteal system.
Both systems follow the rules established by Roux in 1895 [3]. While disturbances in circumferential growth are rarely encountered, longitudinal overgrowth or premature growth arrest may be observed after practically any injury. Injury can sometimes stimulate extra growth but this is generally temporary and rarely causes significant deformity. Transient growth arrest occurs after most physeal injuries, leading to the formation of growth arrest lines, which are visible on x-ray. Permanent growth arrest is rare but serious.
In general, it may be assumed that:
Pediatric fractures tend to heal rapidly and reliably.
Malunions remodel adequately in most instances, depending on the age of the child, degree of displacement, and location of the fracture (segment).
Apart from physeal injuries with growth arrest, only failures of treatment lead to permanent angulation of the immature skeleton. This applies mainly to diaphyseal fractures.
2.1 Regulation of epiphyseal growth
The physis (growth plate) is the primary center responsible for the longitudinal growth of bones. It can be divided into two sections: the epiphyseal zone where proliferation predominates and the metaphyseal zone without proliferation [4]. In the epiphyseal zone the proportion of the matrix surpasses that of the cellular elements, while the contrary is the case for the metaphyseal zone [5]. According to Trueta and Morgan [6], the growth plate can be divided into five zones:
Bone matrix
Proliferation
Mature and hypertrophic cartilage
Mineralized cartilage
Cell degeneration and bone formation
The physis is surrounded by the perichondrium, which is responsible for the circumferential growth of the cartilaginous zone, and therefore of the epiphyseal/metaphyseal end of a bone. The blood supply in this zone is of major importance. The epiphysis, metaphysis, and perichondrium are supplied by three separate nutrient arteries.
The metaphyseal area of the physis shows little resistance to bending and shear force because of its high cellular ratio. Consequently, injury usually occurs through this part of the growth plate while the epiphyseal portion of the growth plate remains intact. Thus, growth disturbance is rare. Injuries through the metaphyseal area of the physis produce type I and type II Salter-Harris fractures, which are discussed in more detail later in this chapter.
Growth disturbance following such injuries is rare and the etiology of this serious complication is not fully understood, but is probably related to severe crushing accompanying the fracture as well as undulations in some physes.
Another important function of the physis of long bones is the modeling of articular surfaces through modified growth of the articular cartilage. Any injury to the epiphysis must be considered as an articular injury.
2.2 Growth and remodeling in the diaphysis
The diaphysis gains its rigidity by tubular calcification in the middle of the immature shaft. Du Hamel [7] described the circumferential growth of the diaphysis by periosteal apposition accompanied by endosteal resorption. The thickness and size of the ossified tubes is directly related to the mechanical stress, which seems to influence the remodeling process. Toward the neighboring joints, the tubular structure widens and the metaphysis broadens through periosteal appositional growth.
2.3 Growth disturbances
If the growth plate is open, there are two possible patterns of growth disturbance. In one situation, bone growth is stimulated; in the other, the activity of the physis is reduced by partial or total premature closure. While the latter situation is permanent, growth stimulation is temporary and occurs only during the repair phase of an injury or a bone/articular infection to the adjacent diaphyseal or metaphyseal bone [8].
Careful attention must be given to all periepiphyseal injuries, not just to physeal fractures.
Growth arrest can occur after any physeal injury and in exceptional circumstances can follow simple metaphyseal fractures. The risk of growth disturbance must be communicated to parents. Secondary injury through treatment that is too vigorous should be avoided.
2.4 Potential to remodel
In the upper extremity, longitudinal growth mainly occurs at the proximal humerus and distal radius/ulna (the shoulder and wrist, respectively). The proximal and distal growth centers of the upper humerus and distal radius and ulna account for most growth in the extremity. Thus, the remodeling potential in these areas is considerable. Fractures around the elbow, especially the distal humerus, however, have much less remodeling potential. The proximal ulna has no real growth plate, it is an apophysis and not responsible for longitudinal growth. This is the reason why Monteggia lesions with fracture or bowing of the proximal ulna have a low remodelling capacity.
The opposite is true of the lower limb. Most growth occurs around the knee (at the distal femur and proximal tibia) and this is where the greatest degree of remodeling will take place. However, the extent of remodeling is less than observed in the upper limb. A fracture of the distal femoral or proximal tibial epiphysis is always related to high-energy trauma with a major risk of growth disturbance.
2.4.1 Factors affecting remodeling potential
Four major factors have a bearing on the potential for angular remodeling:
Skeletal age
Individual potential of a specific growth plate (eg, proximal humerus > distal humerus)
Proximity to the joint
Orientation to the axis of the joint
2.4.2 Remodeling of specific fractures
The capacity of a child′s fracture to remodel after malunion depends on multiple factors, including the age of the child, site of the injury, direction of the deformity in relation to the nearby joints, and the integrity of the growth plates. Rotational deformity will not remodel and tolerance of this deformity will depend upon the degree of compensatory rotation available at either the hip or shoulder. General guidelines for remodeling of specific fractures are shown in Table 4.4-1 .
3 Clinical examination of the injured child
3.1 Case history
The aim must be to perform a focused clinical examination without causing pain. This includes obtaining a history, with the help of the parents, and an examination of all vital parameters according to the advanced trauma life support (ATLS) protocol, when appropriate. The history should carefully establish the mechanism of injury. Nonaccidental injury should be excluded with the help of the case history. Unnecessary or painful manipulations should be avoided and pain relief should be provided before radiological examination.
3.2 Polytrauma
The algorithms for initial treatment should follow ATLS guidelines and are similar to those of the adult (see chapter 4.1). Long-bone stabilization facilitates nursing and rehabilitation but does not affect lung function in children, and so early total care is often appropriate. Thus, early or immediate stabilization of all major long-bone fractures, the unstable pelvis, and the spine has high priority. External fixation allows rapid stabilization in children with polytrauma. A child′s cardiovascular system can compensate for blood loss for a long time, followed by a sudden circulatory collapse that may be difficult to manage.
After successful resuscitation, the severely injured child should be transferred as rapidly as possible to a specialized center.
Spinal column injuries are infrequent in children and represent only about 3% of all pediatric injuries. However, postmortem studies in children younger than 16 years who died of high-energy trauma have shown that the incidence of spinal fractures is about 12% [1]. The upper cervical spine is the most commonly injured vertebral zone. Pain, torticollis, limitation of motion, and muscle spasm should raise suspicion of an injury to the cervical region. Flexion forces tend to produce more severe spinal cord injuries than extension forces in the cervical region.
In the traumatized child, the pelvic ring is assessed after the spine has been protected. Most pediatric pelvic fractures are stable. Adequate x-rays should be obtained and computed tomographic (CT) scans may be needed to complete the evaluation.
Acetabular fractures represent approximately 6% of all pelvic fractures. It is important to recognize fractures of the triradiate cartilage, as this can produce a central growth arrest resulting in acetabular dysplasia with lateral subluxation of the femoral head. This usually occurs in children younger than 8 years, an age in which such injuries are hard to recognize.
3.3 Limb injuries
To prevent pain, manual examination should be systematic and gentle.
An alert child will usually indicate where it hurts. Direct questions may warn the child of a forthcoming (painful) examination, causing distress and making further examination impossible. Sometimes, examination may have to be restricted to simple observation plus the absolutely essential peripheral neurovascular examination.
Any suspicion of a possible fracture should be followed up by an x-ray examination.
4 Imaging in children
X-ray evaluation of each suspected lesion must include at least two views taken at 90° to each other. Both projections should include the joint above and the joint below the suspected fracture area. Unlike adult practice, if the first x-ray clearly shows a grossly displaced fracture that will require formal treatment under anesthesia, a second view may not be required, thereby preventing further painful positioning. In this situation a clear examination of the adjacent joints under image intensification is mandatory.
When the diagnosis is unclear, comparison views of the other side are a last resort and not generally recommended to protect children from undue radiation. All emergency departments should have an atlas of normal appearances and variants; consideration should be given to repeated x-ray after an interval or referral to a specialist in children′s fractures. Suspected fractures should be immobilized for 5–7 days and then reexamined with further x-rays as indicated. Follow-up x-rays are also indicated in fractures with a high incidence of secondary displacement, such as the lateral humeral condyle.
Other diagnostic tools, such as ultrasound, CT scan, or magnetic resonance imaging (MRI) should be used appropriately and after consultation with a radiologist. A CT scan results in a relatively high dose of radiation and must be used with caution in children, particularly those younger than 2 years as there may be a significant risk to the thyroid and thymus glands. However, CT scanning is most helpful in evaluating the head, spine, and pelvis. The risks and benefits for each individual patient must be considered. Ultrasonography is increasingly used for the diagnosis of intraarticular injuries in the younger child with a large mass of cartilage, for example the lateral humeral condyle. An MRI gives excellent images but will require anesthesia in smaller children.
5 Classification of children′s fractures
Articular and periarticular fractures in children are injuries that inevitably involve the physis.
Both the treatment and prognosis for physeal injuries depend on the type of injury.
5.1 Physeal injuries—Salter-Harris classification
The most frequently used classification of physeal injuries is that of Salter-Harris [14], which describes five different types. It fails, however, to differentiate injuries to the zone of Ranvier at the periphery of the physis. These may be due to ligamentous avulsion or open abrasive trauma. Rang [15] proposed that these be included retrospectively in the Salter-Harris classification as type VI ( Table 4.4-2 ).
5.2 AO Pediatric Comprehensive Classification of Long-Bone Fractures
Because the Salter-Harris classification only describes fractures of the epiphyseal zone, the Pediatric Expert Group of AO introduced a special classification that is appropriate to immature bones and describes all the bone segments of the long bones.
This is built upon the classification of adult fractures in its specific, essential features and also integrates classifications such as that by Salter-Harris and other well-known systems ( Fig 4.4-1 ).

This system was validated and published as the AO Pediatric Comprehensive Classification of Long-Bone Fractures, and essentially comprises the elements that follow [16, 17].
5.2.1 Bone and segment
The bone is coded in the same order as in the AO/OTA Fracture and Dislocation Classification for adults:
Humerus
Radius/Ulna
Femur
Tibia/Fibula
The segments within the bones also follow a similar coding scheme:
Proximal
Diaphyseal
Distal
However, their identification differs from adults. For pediatric long-bone fractures, the metaphysis is identified by a square whose sides have the same length as the widest part of the physis in question. For paired bones (radius/ulna and tibia/fibula), both bones must be included in the square. Consequently, the three segments ( Fig 4.4-2 ) can be defined as:

Segment 1 | Proximal epiphysis and metaphysis |
Segment 2 | Diaphysis |
Segment 3 | Distal metaphysis and epiphysis |
Pediatric malleolar fractures are rare and have a different morphology than those of adults. These are placed in segment 3.
5.2.2 Fracture type
The original severity code (A-B-C) used in adults [1] is replaced by a different classification of fractures:
E | Epiphysis |
M | Metaphysis |
D | Diaphysis |
This terminology is accepted worldwide and is relevant to pediatric fractures.
Using the E-M-D code makes it possible to identify intraarticular and extraarticular fractures without ambiguity, since epiphyseal fractures are intraarticular fractures by definition. Metaphyseal fractures are identified by the position of the square (the center of the fracture lines must be located in the square) with one side over the physis.
5.2.3 Child code
Specific pediatric features (also called child patterns) are noted in a child code. For easier recognition, this code is preceded by a forward slash “/” within the entire classification code ( Fig 4.4-1 ). Relevant child patterns are specific to one of the fracture types, E, M, or D, and hence grouped accordingly ( Tables 4.4-3 – 5 ).
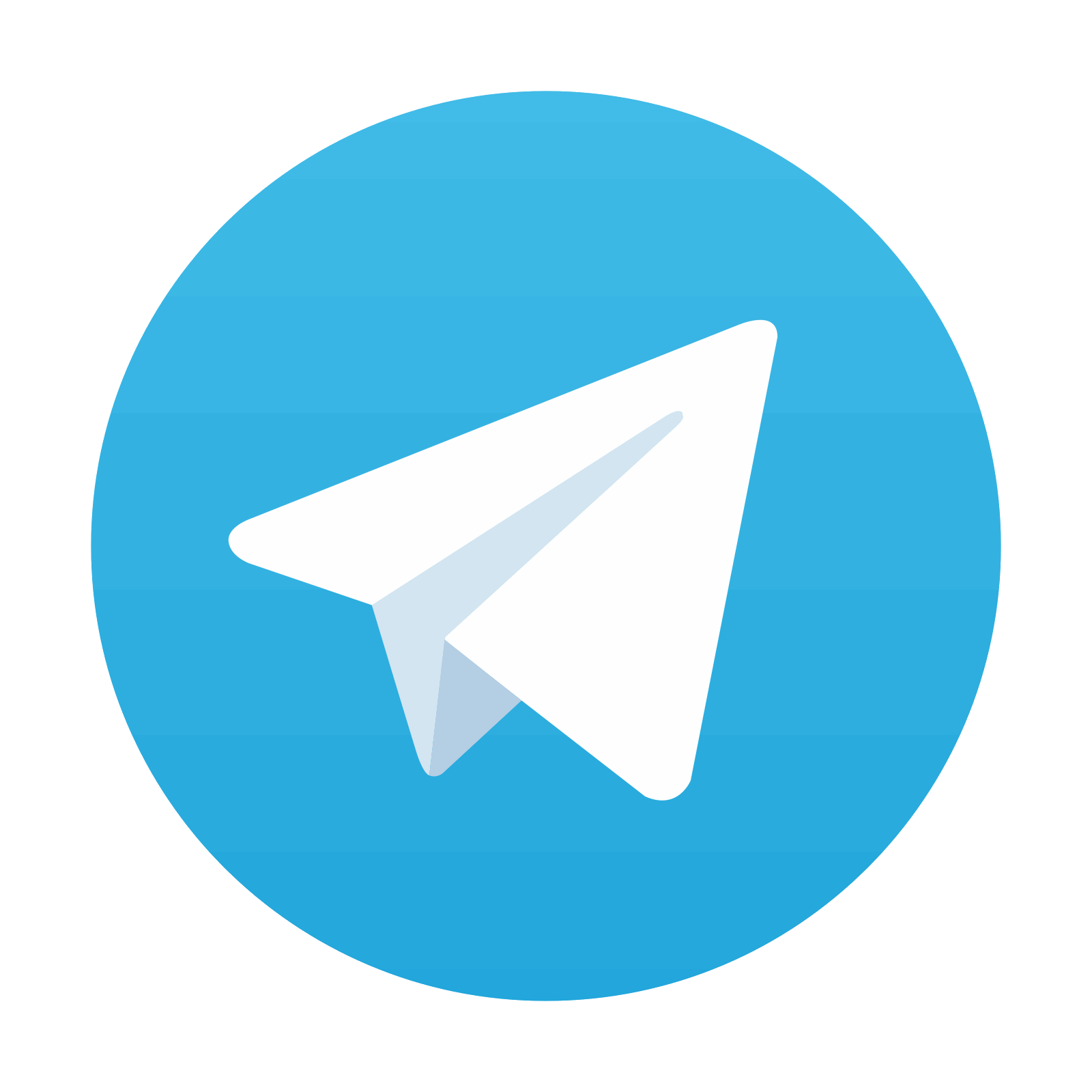
Stay updated, free articles. Join our Telegram channel

Full access? Get Clinical Tree
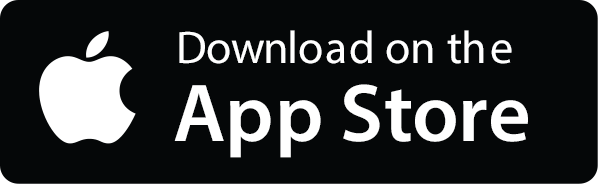
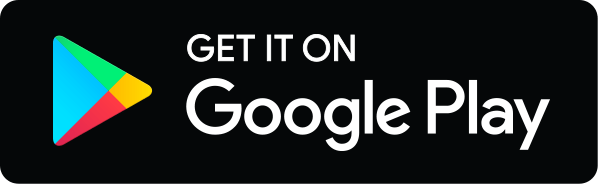
