Fig. 36.1
Lateral aspect of a left knee. The superficial layer (1) of the iliotibial band (ITB) has been flapped down, revealing the Kaplan fibres. The lateral superior genicular artery (5) passes through the lateral intermuscular septum (9) between the proximal (2) and supracondylar insertion (3). The retrograde insertion (4; capsulo-osseous layer) forms a sling around the posterolateral femur and inserts distally somewhat posterior to Gerdy’s tubercle (8). Lateral collateral ligament (6); fibular head (7) (Reprinted from [26], with permission from Sage Publications)
If the ITB is split along its fibres, on the anterior edge of the band which passes to Gerdy’s tubercle, the cut edge may be reflected posteriorly, and that manoeuvre reveals the deep structure of the ITB, which has been referred to as the capsule-osseous layer of the ITB [43]. There is effectively a strong link from its attachment at Gerdy’s tubercle and posterior to it (which is the area of the Segond avulsion), along the deep aspect of the ITB, to the Kaplan fibre attachments to the lateral side of the femoral metaphysis. This band is obviously tightened by tibial internal rotation. This band is functionally distinct from the superficial layer of the ITB, due to its attachments to the distal-lateral femur, and is not a distinct tissue layer which may be separated from the superficial layer of the ITB.
Removal of the ITB exposes the capsular and other deep structures. There has been much recent interest in the anterolateral ligament (ALL), which has been described by Claes et al. [6] and others [9, 16, 46], with differing interpretations. The present authors have consistently found an ALL which has a femoral attachment that is a mean of 8 mm proximal and 4 mm posterior to the lateral epicondyle [9], although it should be noted that these measurements are variable between knees, with attachments varying from proximal to posterior to the epicondyle [7]. The ALL then passes over (superficial to) the lateral (fibular) collateral ligament (LCL) and courses down to a distal attachment to the tibia, midway between the distal fibular attachment of the LCL and Gerdy’s tubercle, approximately 10 mm below the joint line. This is the area from which a bone fragment may be avulsed during ACL rupture – the Segond fracture [40]. In its distal part, the ALL passes over the anterolateral aspect of the lateral meniscus, and the ALL has proximal and distal attachments to the rim of the meniscus, leaving a tunnel through which the inferior lateral branch of the genicular artery passes [6, 9].
There has been widespread agreement regarding the distal attachment of the ALL, but the femoral attachment has been subject to debate. Vincent et al. [46] described the ALL as seen via an arthrotomy during knee replacement when, with the patella reflected laterally, a fibrous capsular band was seen, passing antero-distally from the anterior/distal aspect of the epicondyle. Claes et al. [6] also showed their ALL attaching anterior/distal to the epicondyle and described it as a capsular ligament, with no mention of it passing superficial to the LCL. The attachment described by Dodds et al. [9] has been confirmed recently [25], and there is an accumulation of evidence to support this description, although the exact point may vary from proximal to posterior to the epicondyle [7, 25, 38]. This is an important point, because, as with the ACL, the isometry with knee flexion depends principally on the femoral attachment site, and for the ALL to resist the pivot shift, it needs to be tight near to knee extension [27]. This condition is met by an attachment proximal/posterior to the lateral epicondyle, whereas an anterior/distal attachment leads to graft slackening when the knee is extended [9, 27].
The anatomy of the fibrous structures which reinforce the joint capsule remains poorly defined, and this capsular complex has received many names across the years, perhaps most commonly being called the ‘mid-third capsular ligament’ [19], and has been associated with the avulsion fracture of Segond [40]. Further studies that include transillumination may build on the work of Dodds et al. [9] to provide a more complete understanding of whether the capsular fibres are a significant structure (see biomechanics, below), but recent findings have suggested that the overlying structures may be more important for resisting the pivot shift.
A concluding observation is that some of the variation in the literature regarding the importance of the ALL appears to have originated from differing interpretations of the anatomy, such that several of the structures and layers of tissue described above may have been conflated into ‘the ALL complex’, and then it appears that what may be loosely termed ‘the ALL’ is actually a bulkier and stronger structure than the isolated ALL [7, 26].
36.3 Biomechanics
Several studies have investigated the biomechanical rationale for adding a lateral tenodesis to a modern ACL reconstruction. Samuelson et al. [39] found that an intra-articular ACL reconstruction was unable to restore normal stability to an injured knee when a combined simulated anterolateral plus ACL rupture was present. If an extra-articular tenodesis was added, using a strip of the ITB, the rotational instability was eliminated. The converse of this was a cadaver study of isolated ACL injury, which did not find a biomechanical advantage of adding an extra-articular tenodesis to the intra-articular ACL reconstruction [1]. Engebretsen et al. [10] found a load sharing between the extra- and intra-articular structures, suggesting that the load on the ACL graft would be reduced by using such a combination.
Biomechanically, the principal reason to use a lateral extra-articular procedure is to have the best chance to control abnormal tibial internal rotation laxity, which is an important component of the pivot shift [5]; reviews of the results of ACL reconstruction typically report 15 % of cases having residual ‘pivot-glide’ laxity [12]. The reason for going to the lateral aspect of the knee is that, when the knee is intact, the axis of tibial internal-external rotation is close to the centre of the knee: Kaneda et al. [24] showed that, although the axis moved across the range of knee flexion, on average it was at the tip of the medial tibial intercondylar spine. This means that neither of the cruciate ligaments has sufficient moment arm to control tibial internal-external rotation effectively, and there have been differing conclusions as to whether isolated ACL injury has a significant effect of tibial rotation. In a real injury, the lateral compartment becomes far more mobile than the medial, and so the axis of rotation shifts medially, sometimes beyond the medial border of the knee [4] (Fig. 36.2). This movement of the axis of rotation means that the lateral aspect of the ACL-injured knee moves anteriorly far more than normal in an anterior draw test, giving rise to the descriptive term ‘anterolateral rotatory instability’ (ALRI), even though the magnitude of the rotation may not be much more than normal.


Fig. 36.2
(a) The axis of rotation in an ACL intact knee is located at the medial intercondylar spine. (b) The axis shifts medially in an ACL-deficient knee, causing a more mobile lateral tibial plateau (Reprinted from [2], with permission from Elsevier)
A corollary of the large moment arm of the lateral extra-articular structures about the axis of tibial internal-external rotation is that they may act as restraints with relatively low tensions. Recent work on the ALL [25, 50] reported that it had a mean tensile strength of only 50–175 N, and so it may not act as a significant restraint in isolation. Conversely, past work on the use of strips of the ITB as an ACL graft [34] showed that it has much greater strength and stiffness, as expected from its large cross-sectional area.
It was noted above that it is important for the lateral extra-articular tenodesis to be relatively tight (i.e. elongated) when the knee is near to extension, in order to restrain the pivot-shift instability. In general, graft isometry is controlled mainly by the femoral attachment, with posterior points causing tightening in extension, and anterior points causing slackening [49]. The transition between these behaviours lies close to the lateral epicondyle, so that the LCL, for example, slackens slowly with knee flexion [42]. The proximal/posterior attachment of the ALL identified by Dodds et al. [9] was found to cause the ALL to be tight from 0 to 60° knee flexion and then to lead to slackening. Similar findings came from the more complete study by Kittl et al. [27], who also examined several lateral extra-articular tenodeses. In particular, they showed that grafts passed deep to the LCL, which is not an anatomical path, could be attached proximally/posteriorly to the femur and have consistent behaviour with slow tightening of approximately 3 mm as the knee extended, the LCL proximal attachment acting like a pulley to control isometry (Fig. 36.3). That appears to be a good surgical method to adopt, because the graft elongation behaviour was insensitive to the site of bone attachment.


Fig. 36.3
Isometry measurements, expressed as % length change during the range of 0–90° knee flexion, for lateral extra-articular reconstructions. Total strain range (TSR) values were plotted onto the femur. Low values indicate near-isometry and high values show larger length changes. Reconstruction guided deep to the LCL and fixed proximal/posterior to the lateral femoral epicondyle showed favourable graft behaviour (Reprinted from [27], with permission from Sage Publications)
A key biomechanical question is ‘which structures are the primary restraints to tibial internal rotation?’ In order to answer this, it is necessary to perform what is known as a ‘cutting study’, in which the movement being restrained – in this case tibial internal rotation – is repeated exactly, while the possible restraining structures are cut sequentially. This is an ideal application for a robot, which records the path of motion of the native knee and can repeat it and then measure the drop in load caused by cutting each structure. This method has been used to show that the primary restraint to tibial internal rotation was the ITB, with varying contributions from the deep and superficial layers as the knee flexed, but with a combined restraint of approximately 75 % of the total torque imposed [26] (Fig. 36.4). The ACL was a significant restraint only in full knee extension. When all of the structures which have been identified as the ALL and related capsule were examined, their total contribution to resisting internal rotation was approximately 10 % and not statistically significant. Other robotic studies [35, 37] came to a different conclusion, but had either removed the ITB prior to starting their measurements or did not distinguish between the ALL and the capsulo-osseous fibres of the ITB inserting at the same tibial attachment site.


Fig. 36.4
Contributions of the anterolateral structures in controlling a 5 Nm internal rotation torque at 0°, 30°, 60°, and 90° knee flexion. Solid columns are data with ACL intact knees (n = 8); cross-hatched columns indicate results from the ACL-deficient knee (n = 8). Together the superficial fibres (sITT) and the deep fibres (dcITT) of the iliotibial band provide the primary restraint to internal rotation at 30–90° flexion. Conversely, the anterolateral ligament (ALL) and the anterolateral capsule (Cap) show a non-significant contribution in restraining internal rotation. Medial collateral ligament (MCL; n = 4); posteromedial capsule (PMC; n = 4) (Reprinted from [26], with permissions from Sage Publications)
It should be noted that the method described above is measuring the load required to create a given tibial displacement and thus allows the restraining load in each structure to be found. The structure carrying most of the load is the primary restraint. That method is not the same as in clinical examination, when the examiner usually imposes a standard load and measures the change of displacement, or laxity. In the latter design, the primary restraint may be ruptured, but the knee may remain close to normal laxity under the relatively small forces imposed by hand, and the result will be cutting sequence dependent. Thus, if all structures other than the ALL have been cut, there will be a large increase in laxity when it is finally cut, but that does not mean that it carried much load when acting in concert with the other structures, particularly the overlying ITB, pre-injury.
36.4 Surgical Procedures
Historical descriptions of extra-articular surgical treatment for ACL insufficiency included various techniques that involved the use of the ITB. The idea behind these reconstructions is to reduce the anterior subluxation of the lateral tibial plateau. Therefore, a strip of the ITB was passed deep to the LCL and fixed on the lateral femur. This tethered the ITB posterior to the flexion-extension axis in order to control the anterolateral rotatory instability. This reduction of the anterior subluxation of the lateral tibial plateau has been shown in an experiment, when inserting a K-wire into the femur anterior to the ‘Kaplan fibres’ (i.e. femoral attachments of the capsulo-osseous ITB) kept the ITB posterior throughout the range of motion. Conversely, when the K-wire was inserted posterior to the Kaplan fibres, the subluxation was still present [15] (Fig. 36.5).


Fig. 36.5
(a) When a K-wire was inserted anterior to the Kaplan fibres, the iliotibial band (ITB) reduced the pivot-shift phenomenon, as it provided a posterior vector throughout the range of motion. (b) Conversely, when inserting it posterior to the Kaplan fibres, the reduced anterior force vector of the ITB could not reduce the subluxation, even at 80° flexion (Reprinted from [15], with permission from Springer)
Isolated lateral extra-articular procedures have largely been left behind due to poor patient outcomes and residual laxity in anterior translation, since the intra-articular ACL deficiency was unaddressed. For a while they were combined with intra-articular ACL reconstructions, but in time due to the success of intra-articular ACL reconstruction, the added extra-articular procedure was abandoned by most surgeons as it was thought superfluous and a source of morbidity. There have, however, been more recent attempts to combine intra-articular ACL reconstruction with anterolateral procedures. Although results have been mixed, some studies suggest a positive effect on knee rotational stability and patient-related outcomes [3, 41, 45, 48].
A recent biomechanical comparison of anterolateral procedures combined with ACL reconstruction compared modern modifications of the ITB- based MacIntosh and Lemaire procedures with an anatomic ALL reconstruction using a hamstrings autograft [20]. A combined anterolateral and intra-articular ACL lesion was created in the knees to simulate the combined injured state that is likely to be present in a knee with a significant anterolateral rotatory laxity [31, 44]. A subsequent ACL reconstruction using an AM-portal technique and a patellar tendon graft placed via the centre of the tibial ACL attachment and the AM-bundle position in the femoral attachment was unable to restore the intact knee biomechanics, leaving residual laxity for anterior translation and internal rotation. An ALL reconstruction, based on the anatomic findings of Dodds et al. [9], was added as a conjunct procedure, but only showed a minor additional effect on knee laxity. When instead combining the ACL reconstruction with either a modified MacIntosh or a Lemaire procedure, both utilising a mid-strip of the ITB, residual laxities from ACL reconstruction alone were normalised. In the following section we will therefore elaborate on these two techniques.
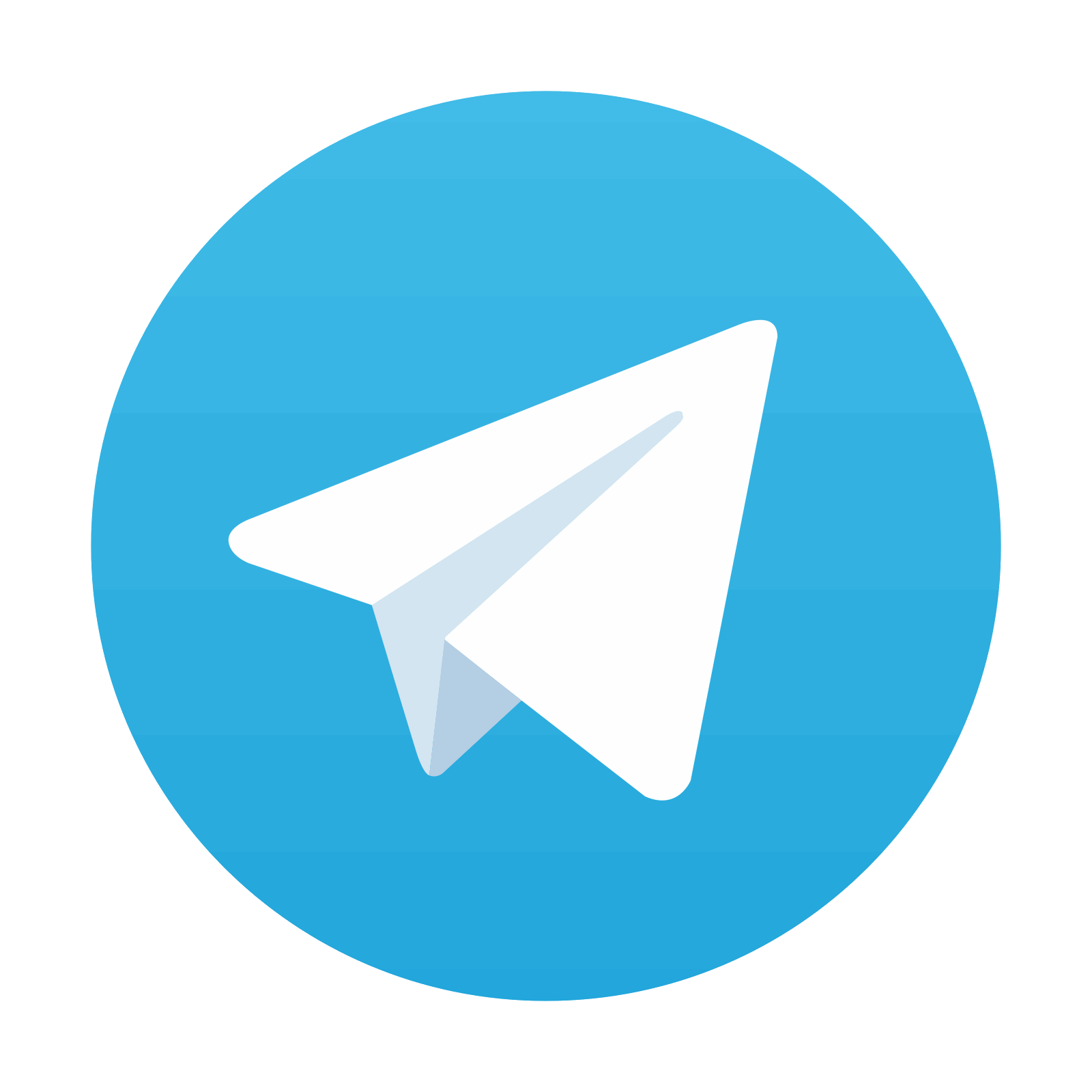
Stay updated, free articles. Join our Telegram channel

Full access? Get Clinical Tree
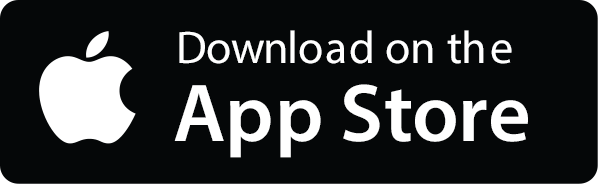
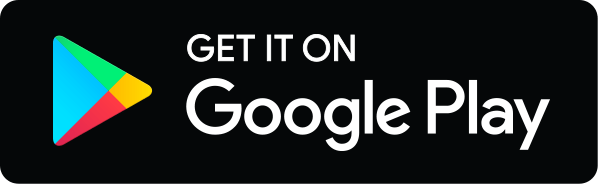