Fig. 8.1
Causes of need for long-term care. The proportions of the top four causes in the Ministry National Livelihood Survey for 2011. The proportion of persons for whom care became necessary because of locomotorium-related causes including fall/fracture, joint disease, and spinal cord injury ranked first at 23 %
Such circumstances are the background for the unique proposal in 2007 by the Japanese Orthopaedic Association of the concept of locomotive syndrome (hereafter referred to as “locomo”), which is based on the idea that locomotorium disorders inhibit healthy longevity [2]. The definition of this concept was revised in 2014 as a condition of decreased locomotive function due to locomotorium disorder [3]. The literature describes that osteoporosis, sarcopenia, fracture, osteoarthritis, spinal osteoarthritis, and neuropathy are the main diseases underlying locomo (Fig. 8.2). These locomotorium disorders are obviously the main factors that inhibit healthy longevity. Of them, sarcopenia is a relatively new disease concept [4].
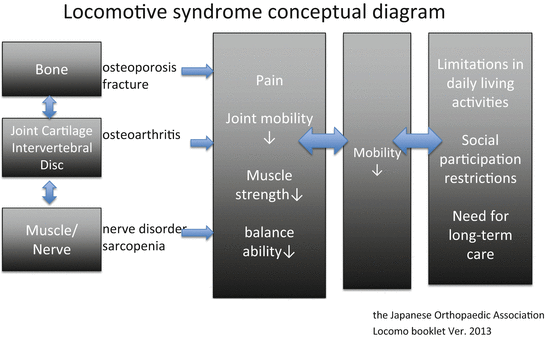
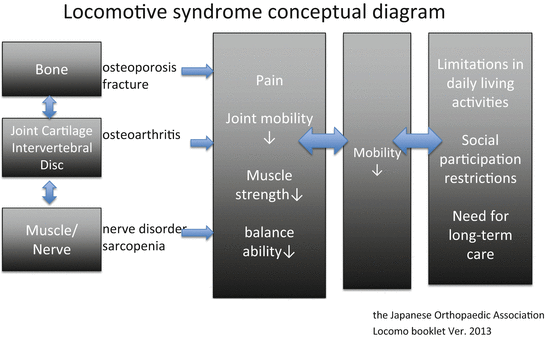
Fig. 8.2
Locomotive syndrome conceptual diagram. According to a brochure on locomotive syndrome prepared in 2013 by the Japanese Orthopaedic Association. This diagram shows the underlying disease to which bone, articular cartilage/intervertebral disc, and muscle/nerve tissues are related, the symptoms that are caused by the disease, and their effects on mobility and correlation with activity
Among the above-described causes of need for support or care, fall/fracture accounted for 12 % and is thought to result from sarcopenia (factor of fall) and concurrent osteoporosis (factor of fracture). The main reason that osteoporosis and sarcopenia are addressed in this chapter seems to be the serious condition that the concurrent occurrence of these two diseases leads to.
The science of osteoporosis , including definition, diagnosis, prophylaxis, and treatment, has dramatically advanced over the past 20 years and boasts of drug treatment with strong evidence. It is considered a skeletal disease that is characterized by decreased bone strength and likely to increase the risk of fractures [5]. A cohort study of community residents aged ≥ 40 years showed that the prevalence of osteoporosis by transcervical bone density was 12 % in men and 27 % in women and that the estimated number of patients is 10.7 million [6, 7]. Decreased bone strength alone does not produce any symptoms, nor does it directly decrease locomotive function. In the Japanese diagnostic criteria, osteoporosis is diagnosed when the patient has a history of fragility fracture due to a slight external force. In the absence of a history of fracture, osteoporosis is diagnosed when bone density is <70 % of the young adult mean (YAM) [8].
On the other hand, in the science of sarcopenia , the definition and diagnostic criteria have just been established and there is little evidence for prophylaxis and treatment other than exercise and nutrition. Sarcopenia is thought to lead to health problems such as movement disorder, increased risk for fall/fracture, decreased ability to perform activities of daily living, increased disability, loss of independence, and an increased risk of death [9–12].
A consensus report published in 2010 by the European Geriatric Medicine Society and related organizations (European Working Group on Sarcopenia in Older People [hereafter referred to as “EWGSOP”]) [13] defines sarcopenia as “a syndrome characterised by progressive and generalised loss of skeletal muscle mass and strength with a risk of adverse outcomes such as physical disability, poor quality of life, and death.”[14] Sarcopenia was initially diagnosed by muscle mass alone [15] and changes in diagnostic procedures occurred subsequently. EWGSOP and the Asian Working Group for Sarcopenia [16] proposed a diagnostic algorithm using gait speed and grip strength for screening and muscle mass for diagnosis. In addition, the International Sarcopenia Consensus Conference Working Group [17] proposed a diagnostic algorithm that uses gait speed alone for screening and muscle mass for diagnosis. As is seen from the above description, although the diagnostic procedure has not yet been internationally standardized, muscle mass is the criterion used for the final diagnosis of sarcopenia and the most important factor in any procedure. In our study of sarcopenia as described below, it was diagnosed using muscle mass.
Only a limited number of studies have examined the correlation between osteoporosis and sarcopenia; [18] thus, this article addresses the question.
8.2 Relationship Between Bone Mass and Muscle Mass
8.2.1 Bone Mass and Muscle Mass Database
The human body is composed of oxygen, carbon, hydrogen, and other atoms at the atomic level; water, fat, protein, and other molecules at the molecular level; and muscle, fat, bones, blood, and other tissues at the tissue level. The body composition at the tissue level continues to change gradually even during adulthood, although not as greatly as during the growth period [19]. Bone, muscle, and fat mass all decrease in old age, and these decreases are expected to increase the risk of fall/fracture in the following manner: the decrease in muscle mass is likely to cause falls, which in turn are likely to cause fractures because of a decrease in impact absorption due to the reduced fat mass and a decrease in bone strength due to the reduced bone mass. However, only a small number of studies have been conducted on these changes and most were cross sectional. Hence, much of the details remain unknown. Thus, we used the database of bone mass and muscle mass in our hospital to study the correlation between the two parameters.
Bone density measurements have long been taken using dual energy X-ray absorptiometry (DXA) to diagnose osteoporosis. The typical sites at which bone density is measured by DXA are the lumbar spine, proximal femur, and distal radius. The actual principle of measurement by DXA is that the accurate calculation of fat and lean masses is always performed internally and automatically for the purpose of obtaining an accurate bone mass from body composition. However, DXA devices that are used to measure bone mass at these sites do not show the lean mass value since it is unnecessary for the diagnosis of osteoporosis.
On the other hand, DXA devices that are used to measure the whole-body bone mass show both the whole-body bone mass and the regional fat and lean masses as the body composition. In other words, in this measurement mode, muscle mass, which can be calculated from lean mass, can be obtained in addition to bone mass.
To measure bone density, we have been using the entire body in addition to the lumbar spine and proximal femur since the 1990s in routine examinations of outpatients and inpatients of the departments of orthopedic surgery, internal medicine, rehabilitation, and gerontology of our hospital who are suspected to have osteoporosis. This patient database contains the bone mass values at the lumbar spine and proximal femur and of the whole body, and the muscle mass values calculated from lean mass as described later, and it can be used as a bone and muscle mass database.
The data used in this investigation represented that obtained from 2800 patients (655 men, 2118 women; age range, 20–102 years) who underwent body composition measurements by DXA between June 2002 and January 2009. The mean values for age, body height, body weight, bone mass, muscle mass (appendicular lean mass and the corresponding skeletal muscle index [SMI], a value corrected for body height), and fat mass for this database are shown in Table 8.1. Appendicular lean mass is the sum of arm lean mass and leg lean mass. SMI is a value obtained by correcting appendicular lean mass for body size as described later and is considered an international standard parameter of muscle mass.
Table 8.1
Values for the parameters in the bone and muscle mass database
Sex | Age (year) | Height (cm) | Body weight (kg) | SMI (kg/m2) | Total bone mineral content (g) | Total fat mass (g) | Appendicular lean mass (g) | Arm lean mass (g) | Leg lean mass (g) | |
---|---|---|---|---|---|---|---|---|---|---|
Men: 655 patients | Average | 71.1 | 152.4 | 51.7 | 6.081 | 1746 | 14,196 | 14,068 | 3487 | 10,759 |
Women: 2118 patients | SD | 12.1 | 9.1 | 11.5 | 0.976 | 531 | 7428 | 3609 | 1027 | 2413 |
Range | 20–102 | 125–186 | 24–136 | 1.608–10.586 | 469–4531 | 876–59,991 | 3280–30,353 | 769–8998 | 2512–25,637 |
Furthermore, Table 8.2 and Fig. 8.3 show variations in mean values for bone, muscle, and fat mass by age group. The data shown seem to show a tendency of these three tissue mass types to decrease with age in the investigated patient group. However, SMI, which is obtained by correcting for body height and considered to reflect muscle mass more accurately, did not necessarily show a tendency to decrease linearly with age (Fig. 8.4).
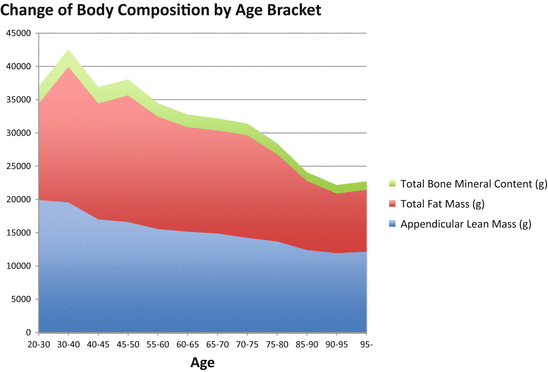
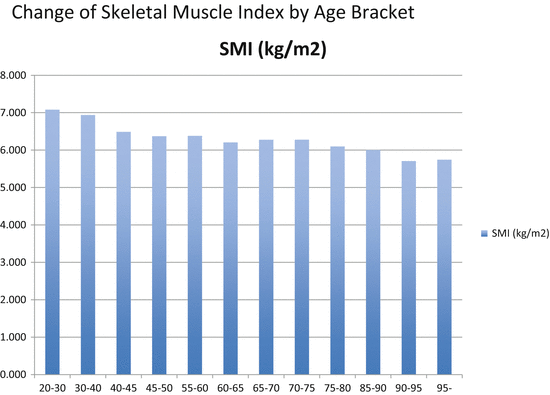
Table 8.2
Mean bone, muscle, and fat mass values by age bracket
Age (year) | n | Total bone mineral content (g) | Total fat mass (g) | Appendicular lean mass (g) |
---|---|---|---|---|
20–30 | 5 | 2510 | 14,499 | 19,901 |
30–40 | 31 | 2628 | 20,356 | 19,548 |
40–45 | 29 | 2457 | 17,393 | 17,008 |
45–50 | 123 | 2399 | 19,034 | 16,601 |
55–60 | 212 | 2044 | 16,857 | 15,546 |
60–65 | 356 | 1930 | 15,678 | 15,150 |
65–70 | 379 | 1829 | 15,465 | 14,872 |
70–75 | 482 | 1758 | 15,418 | 14,213 |
75–80 | 422 | 1642 | 13,110 | 13,668 |
85–90 | 202 | 1319 | 10,367 | 12,384 |
90–95 | 105 | 1262 | 8967 | 11,917 |
95– | 30 | 1283 | 9279 | 12,148 |
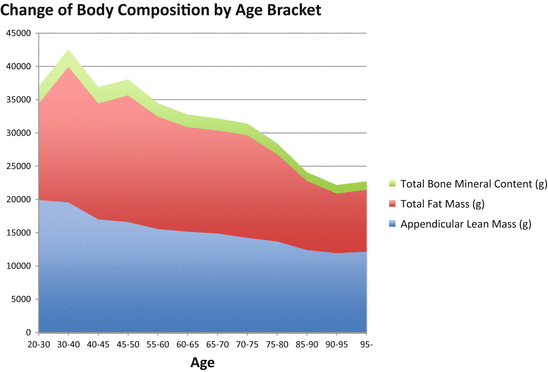
Fig. 8.3
Change of body composition by age bracket. Data of 2800 patients including outpatients and inpatients in the bone and muscle mass database in whom bone and muscle mass were measured by dosimetry X-ray absorptiometry for suspected osteoporosis. This figure shows the distribution of body composition (bone, fat, and fat-free mass) by age bracket in this patient group
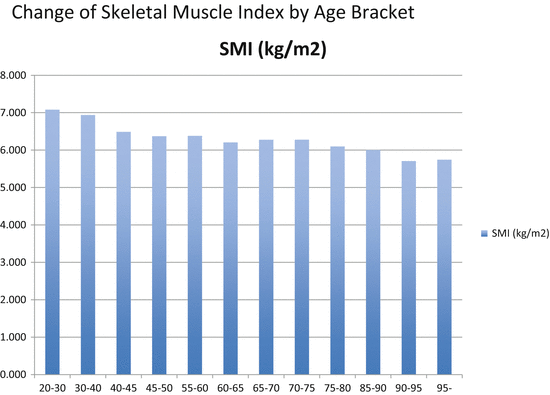
Fig. 8.4
Change of skeletal muscle index by age bracket. This figure shows the distribution of skeletal muscle index (SMI) by age bracket in 2800 patients in the bone and muscle mass database. SMI is an international standard indicator used to assess muscle mass and is calculated by the following formula: SMI = appendicular lean mass (kg)/height (m2). Appendicular lean mass is the sum of arm lean mass and leg lean mass
8.2.2 Measurement of Bone Mass and Diagnosis of Osteoporosis
Bone mass was measured by DXA (DPX-NT; GE Medical Systems Lunar, Madison, WI, USA) at three sites, i.e., with the anteroposterior views of the lumbar spine and the proximal femur and as the whole-body bone mass. In all three sites, two-dimensional bone density is calculated in grams per squared centimeters. In patients with a hip fracture, the measurement was performed at the unaffected proximal femur as soon as the patient was admitted to the hospital (within 3 days). Of the three sites, the lumbar spine and the proximal femur are most commonly used for the diagnosis of osteoporosis. However, as a patient ages, bone growth and sclerosis occur more often in the lumbar spine because of spinal osteoarthritis, making the lumbar spine less likely to reflect the accurate bone density of the vertebral body. Thus, the proximal femur was selected as the osteoporosis diagnostic site in this study. In the current diagnosis of osteoporosis, a history of fragility fractures is considered in addition to bone density as described above. There are also two proposals for the normal value of bone density: “less than the YAM – 2.5 SD” by the World Health Organization and “less than the YAM – 30 %” by the Japanese Society for Bone and Mineral Research and the Japan Osteoporosis Society. The latter was selected in this study. In summary, osteoporosis is diagnosed when the transcervical bone density is <30 % of the YAM, osteopenia is diagnosed when the density is ≥70 % and <80 % of the YAM, and osteoporosis is diagnosed when both osteopenia and a fragility fracture are present [8].
8.2.3 Measurement of Muscle Mass and Diagnosis of Sarcopenia
In the measurement of muscle mass, body composition and bone mass are measured using the same DXA device. Body composition, including bone mineral content, fat mass, and lean mass, was measured separately for each part of the body. Muscle mass was calculated from lean mass according to the method by Baumgartner et al. The lean masses of the arms and legs were nearly equal to the skeletal muscle mass and are known as the appendicular lean mass. Since appendicular lean mass is affected by body size and ethnicity, SMI as described below is often used to correct the effect.
SMI was calculated by the following formula:
Sarcopenia was defined according to only the skeletal muscle mass index (SMI) in this database, using the criteria for the Japanese based on the report by Sanada et al. [20]. The value of Japanese criterion was an SMI below 5.46 kg/m2 in women and below 6.87 kg/m2 in men.

As mentioned above, EWGSOP’ paper suggested an algorithm for sarcopenia case finding in older individuals based on measurements of gait speed , grip strength , and muscle mass [13].
However, as is obvious from their algorithm, muscle mass has the largest effect on the diagnosis. Thus, the diagnosis of sarcopenia by muscle mass alone is thought to be the most basic approach with the greatest significance.
8.2.4 Relationship Between Bone Mass and Muscle Mass: Proximal Femur Bone Density and SMI
The abovementioned investigation of bone and muscle mass based on our database showed a strong positive correlation between total bone mineral content and appendicular lean mass, which are uncorrected bone and muscle mass, respectively (Table 8.3 and Fig. 8.5). In other words, bone and muscle mass seem to be nearly in direct proportion from a cross-sectional aspect.
Table 8.3
Pearson’s correlation coefficient
Age | Height | Body weight | SMI | Total bone mineral content | Total fat mass | Appendicular lean mass | ||
---|---|---|---|---|---|---|---|---|
Age | r | −0.459 | −0.429 | −0.236 | −0.55 | −0.33 | −0.422 | |
p | .000 | .000 | .000 | .000 | .000 | .000 | ||
Height | r | 0.633 | 0.233 | 0.733 | 0.25 | 0.712 | ||
p | .000 | .000 | .000 | .000 | .000 | |||
Body weight | r | 0.538 | 0.768 | 0.789 | 0.737 | |||
p | .000 | .000 | .000 | .000 | ||||
SMI | r
![]() Stay updated, free articles. Join our Telegram channel![]() Full access? Get Clinical Tree![]() ![]() ![]() |