Study
Video (number)
Subject and sports played (number)
Measurement variables (main results)
Results
Boden et al.
Injury (29)
Injury: basketball (14), handball (7), soccer (3), football (3), cheerleading (1), gymnastics (1)
Sagittal: foot position, ankle angle, knee angle, hip angle
Less planter flexion ankle angle and larger hip flexion angle in injured athletes.
Control (27)
Control: basketball (27)
Coronal: knee angle, hip angle
Hewett et al.
Injury (23)
All basketball
Coronal: trunk angle, knee angle
Greater lateral trunk and knee abduction angle in injured female athletes.
Control (28)
Sheehan et al.
Injury (20)
Injury: basketball (10), soccer (2), football (5), handball (3)
Sagittal: trunk angle, limb angle, COM_BOSa
Greater limb angle and COM_BOS, smaller trunk angle in injured athletes.
Control (20)
Control: basketball (10), soccer (2), football (5), handball (3)
13.2.1 Sagittal View Analysis During ACL Injury
Table 13.1 shows studies (Boden et al. 2009; Sheehan et al. 2012) that used sagittal view analysis to make several important observations. Interestingly, the initial contact with the ground for injured athletes was with the hindfoot or the entire foot, whereas in controls it was with the forefoot or a combination of forefoot and midfoot (Boden et al. 2009). The ankle angle at initial contact was significantly less plantar flexed in the injured group (10.7 ± 9.6 degrees [deg]) than in the control group (22.9 ± 10.1 deg). In addition, the ankle angle of injured athletes changed only a little from the initial contact to the next four frames (120 ms post contact) of the video when compared with a greater change in the uninjured controls. Boden et al. (2009) suggested that the calf musculature may be unable to absorb the ground-reaction forces adequately, following which forces are transmitted directly to the knee in injured athletes because they land flatfooted or with initial hindfoot contact.
Although less knee flexion was observed on recordings involving ACL injury events (Ireland 2002; Krosshaug et al. 2007a), the difference in knee flexion angle between injured and control groups in 2-D video analysis was not statistically significant (Boden et al. 2009). This is a methodological limitation of 2-D video analysis and Boden et al. (2009) stated that the 2-D measurement technique is not sufficiently sensitive to distinguish small differences in joint angle, and knee valgus and rotation may simulate flexion in all subjects. However, the knee was significantly less flexed in the injured athletes at the first frame when the foot was 100 % flat on the ground (average for injured athletes was 17.6 deg and for controls was 39.3 deg), thus causing abnormal force on the knee. This is because the foot becomes completely flat on the ground quicker in injured athletes.
Previous studies investigating hip movement from the sagittal view used two different methods. Boden et al. (2009) defined the angle between a line from the superior tip of the acromioclavicular joint to the superior tip of the greater trochanter and a line from the superior tip of the greater trochanter to the midpoint of the lateral knee at the joint line to define the hip angle. On the other hand, Sheehan et al. (2012) defined the angle between the vertical and the thigh (represented as a line from the center of the knee joint to the center of hip joint) as the limb angle. At initial contact with the ground, Boden et al. (2009) showed that the hip angle was significantly more flexed in the injured athletes (50.1 ± 13.2 deg) than in controls (25.8 ± 14.7 deg), and that the limb angle in the study by Sheehan et al. (2012) was greater in the ACL-injured athletes (48 ± 12 deg) than in controls (31 ± 22 deg). Moreover, Sheehan et al. (2012) reported that the trunk angle, defined as the angle between vertical and the centerline of the trunk, was lower in the injured group (4 ± 14 deg) that in controls (16 ± 13 deg). These hip and trunk positions at initial contact with the ground are important components of the mechanism of non-contact ACL injury.
Sheehan et al. (2012) further evaluated landing by measuring the body’s center of mass (COM) to the base of support (BOS). The distance from the COM to the BOS (COM_BOS) was greater in injured athletes (1.5 ± 0.5 pix/pix) than in controls (0.7 ± 0.7 pix/pix) at initial contact. Discriminant analysis of these data showed that no controls landed with the COM_BOS greater than 1.6, and no injuries in female occurred when athletes had a COM_BOS <1.2. In the COM_BOS range of 1.2–1.6, both provocative and safe landing occurred.
13.2.2 Coronal View Analysis During ACL Injury
Table 13.1 shows results from studies involving a coronal view analysis (Boden et al. 2009; Hewett et al. 2009). Boden et al. (2009) reported no significant difference in knee abduction angle at initial contact between injured (29.9 ± 11.0 deg) and control groups (25.7 ± 12.7 deg). Following the time course, however, significant difference in knee abduction angle between injured and control athletes appeared at the third frame after the initial contact (60 ms post contact) (Boden et al. 2009) and 100 ms post contact (Hewett et al. 2009). Meanwhile, no significant differences in hip abduction angle between injured and control athletes were found in any frame (Boden et al. 2009).
Dempsey et al. (2007) investigated how trunk position in the coronal plane changed knee loading. The mean lateral trunk angle relative to vertical was found to be higher in female than male players during ACL injury, and trended toward being greater than female controls at initial contact (Hewett et al. 2009). Hewett et al. (2009) suggested that trunk position and knee load may be mechanically linked, as lateral positioning of the trunk can create abduction load at the knee.
13.3 2-D Video Analysis for Screening ACL Injury Risk; Approach to Soccer Specific Landing
Previous video analysis studies have collected data on ACL injury events from several sports. These studies provide useful information on the multi-risk component for ACL injury and for the development of an ACL injury prevention program. Therefore, some common risk factors will be included along with the soccer specific movements. Faunø and Wulff (2006) reported that landing after jumping for a header was one of the most frequent actions that lead to ACL injury. By observing this specific action using 2-D video analysis, we will be able to identify the aspects of landing behavior that result in the greatest risk for ACL injury. This new approach has the potential to screen large number of players or actions, and help minimize ACL injuries in soccer. Thd following pilot study analyzed the landing action after players jumped for a header. We screened the variables of uninjured landing events using 2-D video analysis.
13.3.1 Data Collection
We recorded a soccer game in the Kanto Women Football League 2012 using four digital video cameras (Sony HDR-CX590V, Japan) with frame rates of 60 Hz. The cameras were located around the soccer field and video recorded in AVCHD format and high definition (1080i).
Three experts in the field of soccer biomechanics and sports medicine formed the analysis team. The analysis was organized into two stages to identify events for analysis. First, one analyst (S.I) identified single-leg landing events for one of the teams from a recorded video sequence in 2× slow motion. A total of 27 landing events were selected for further analysis. In stage 2, the other two analysts (S.S, S.K) reviewed single-leg landing events after the player jumped for a header and there was a skirmish with another player in mid-air. Criteria for inclusion of a particular event were: (1) a good quality video recording, with the camera angle approximating a sagittal view of the athlete; (2) good visibility of the foot contacting the ground; (3) an unobscured view of the athletes. Events were excluded if the athletes landed without a skirmish with another player. Twelve landing events met the study criteria for inclusion in stage 2.
13.3.2 Video Editing and Analysis
The video recordings were edited using Edius Neo (version 3.5, Grass valley llc, USA). For each event, the frame in which the foot initially contacted the ground was captured and stored in TIFF files for analysis. Image-J (National Institutes of Health, USA) was used for all analyses. For consistency, a single author (S.S) performed all measurements.
The trunk angle, limb angle, and the COM_BOS were measured in this study (Fig. 13.1). The trunk angle was defined as the angle from the vertical to the centerline of the trunk, and the limb angle was defined as the angle between the vertical line and the centerline of the thigh. A positive trunk and/or limb angle indicated that the trunk and/or limb were rotated anteriorly relative to the vertical. The COM was defined as the center of an ellipse delineating the athlete’s trunk and the BOS was defined as the point bisecting the line of contact between the shoe and the floor at initial contact. The COM_BOS (in pixel) was taken along the anterior/posterior direction and normalized by femur length (in pixel). A more detailed description of the method used has been published (Sheehan et al. 2012).
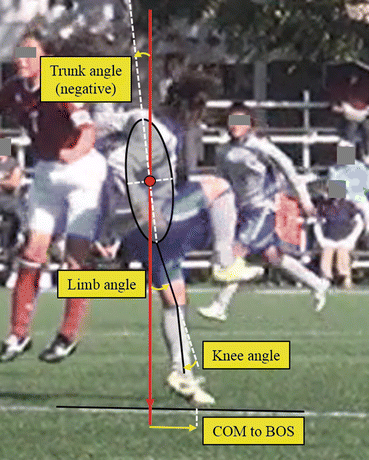
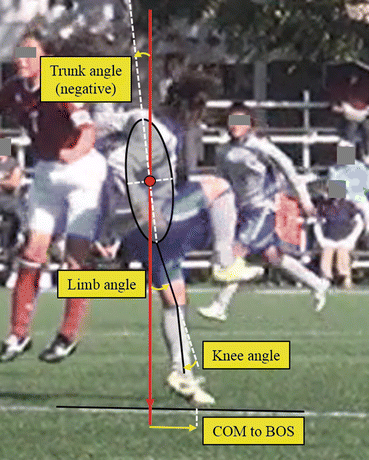
Fig. 13.1
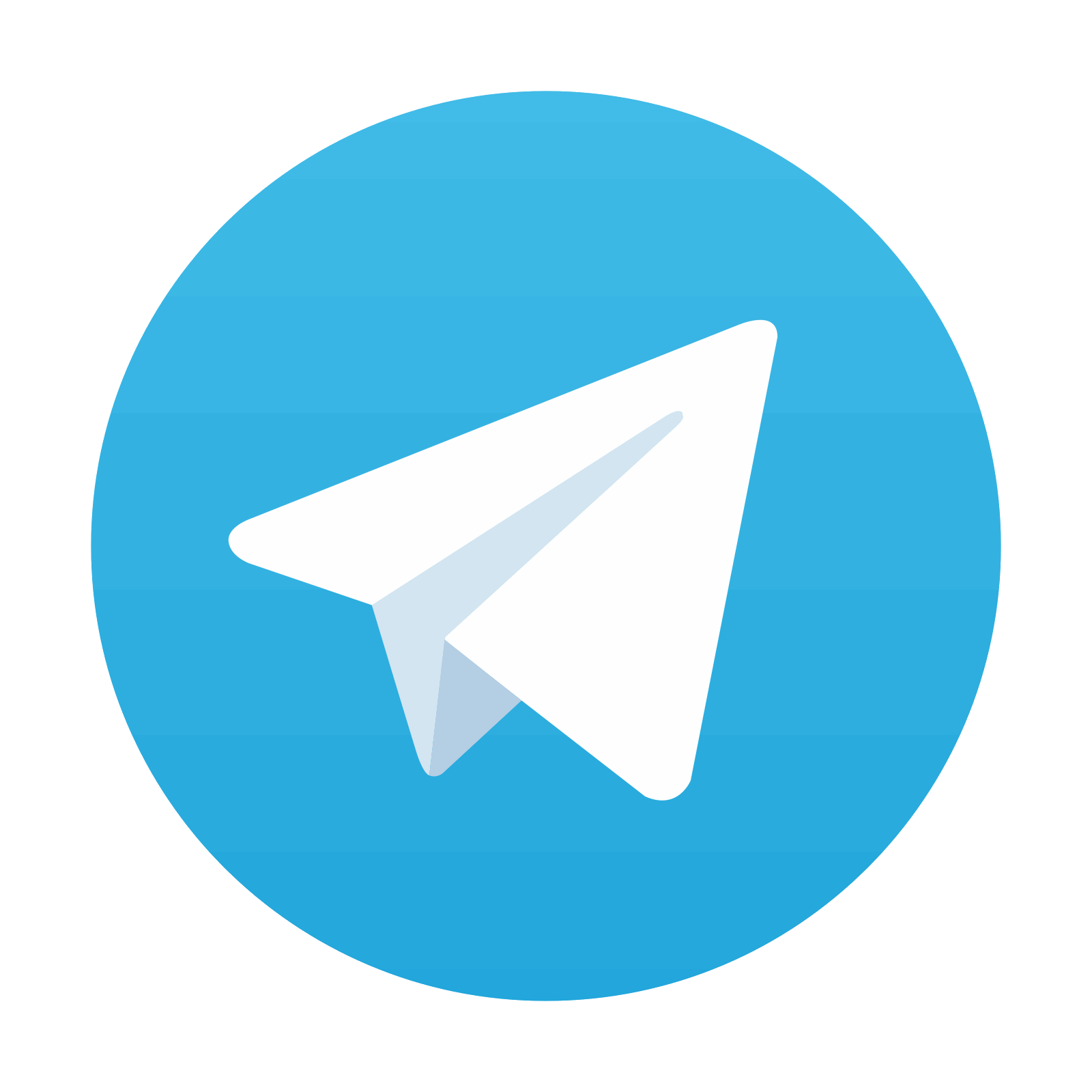
Analysis of landing on the ground after jumping for a header
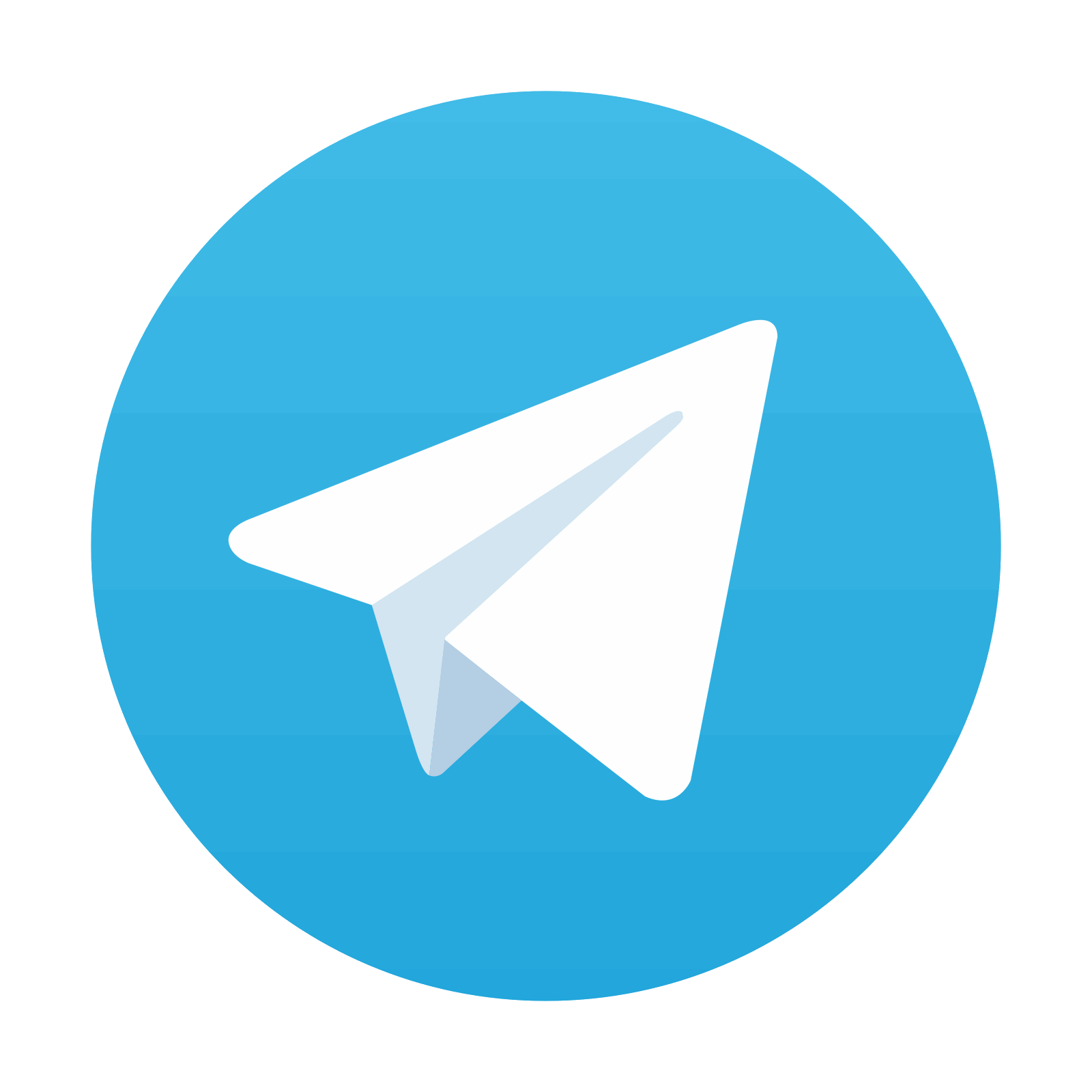
Stay updated, free articles. Join our Telegram channel

Full access? Get Clinical Tree
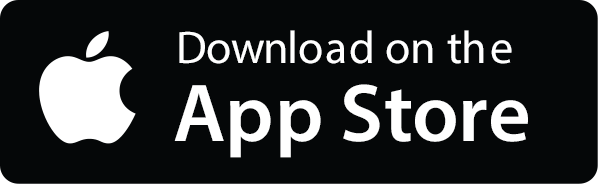
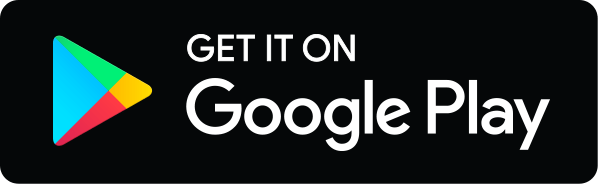
