Fig. 14.1
x, y, and z axes. The three-dimensional universe can be plotted on the axes: x, y, and z. Considering that objects in space may either translate (move to another location without reorientation) or rotate (turn, spin, and be reoriented), these two types of motion on three axes represent the “six degrees of freedom” that engineers use as a constant frame of reference. The permutations for position, considering that translation and rotation can each proceed in positive or negative directions, are huge. Component position in knee arthroplasty can be described precisely as departures from a neutral position according to translation (millimeters or length) and rotation (degrees). In this figure, the z-axis is used to define varus-valgus as rotation around the “z”-axis and “anterior and posterior” as translation on the same “z”-axis
Borrowing from a classic text on spine injury, we can describe stability in a knee arthroplasty as the ability of the joint to maintain its structure and yet permit motion within functional limits when subjected to physiologic loads [2]. When the quadriceps muscle contracts, the knee extends fully but not into recurvatum. The hamstring muscles contract, and the tibia rotates around the “x-axis” (flexes), translates slightly on the “z-axis” (femoral rollback), and rotates internally a few degrees under the femoral condyles around the” y-axis” (screw-home mechanism). (Fig. 14.2). Similar rules apply to the patella. To a lesser degree, the tibia and femur displace in the frontal or coronal plane under varus-valgus bending moments as the collateral ligaments absorb energy and deform elastically.


Fig. 14.2
Stable knee flexion . (a) The hamstring muscles contract, and the tibia rotates around the “x-axis” (flexes), translates slightly on the “z-axis” (femoral rollback), and rotates internally a few degrees under the femoral condyles around the “y-axis” (screw-home mechanism). (b) The tibia does not however dislocate posteriorly once it reaches 90 degrees of flexion, as would happen if only the hamstrings were managing motion. The posterior cruciate and the extensor mechanism help convert hamstring tension into knee flexion. (c) Without the PCL and extensor, the hamstring is likely to sublux the tibia posteriorly. Constraint, including an ultra-congruent articulation, posterior stabilization, and non-linked constrained implants and hinges, can help restore stability, ensuring that hamstring tension continues to result in knee flexion. The essence of stability is to be able to maintain structure and physiologic motion under load
The title of this chapter makes two assumptions: that stability must necessarily have been lost (in order to be restored) and that something described as the “joint line” needs to be retained in a specified position. The former is not a problem in every revision as some arthroplasties fail for reasons other than instability [3]. However, the revision technique for any mode of failure must still ensure stability when components are replaced.
Revisions differ from primary surgery in many ways, including the role of conventional soft tissue releases that are used to correct arthritic deformity and stabilize a primary arthroplasty [4]. These techniques are rarely feasible in revision surgery unless the releases should have been done at the primary surgery but were not. “Rerelease” of ligaments is not viable. Stability in revision surgery is the product of the existing soft tissues and the component’s size, position, and design. A mechanically constrained implant is not necessarily required unless ligaments required for stability have failed. Specific indications for constraint are described below.
Secondly, that the “joint line” should be a primary key to revision surgery is contentious. As with all aspects of surgery, basic precepts and received wisdom should be challenged if revision knee arthroplasty is to be practiced expertly and improved in the future.
Stability and Instability
TKA instability has been reviewed [5, 6]. Several principles guide revision knee arthroplasty and are pertinent to cases of instability. First, no surgery can be recommended before clear steps are planned to solve specific problems that caused a recognized mode of mechanical failure [3, 7, 8] (Fig. 14.3). A generic “repeat arthroplasty,” one performed without an explanation of pain, instability, or stiffness, is likely to fail, as was established in 1988 [9]. The era of “exploratory” or diagnostic surgery has long passed; every failed knee replacement should be evaluated systematically and comprehensively [10]. The patient’s description of symptoms is essential information but does not constitute a diagnosis [5, 11].


Fig. 14.3
Modes of failure planning worksheet for the failed knee replacement. This version is based on mechanisms of failure described by Moreland [8] and has been updated from an earlier version [3]. The eight indications for revision knee arthroplasty are listed on the second column on the left. The sequence, first to eighth, represents a clinical algorithm for consideration of the failed arthroplasty. For each diagnosis, the essential data points that should be recorded are in the columns on the right. The process is intended to be systematic and comprehensive. This sheet represents the system, which can be applied to every problem TKA. To be comprehensive, all eight diagnoses must be considered, even if one diagnosis is established. For example, infection can accompany any other mechanical failure. A knee can be stiff and loose. Because infection should be suspected in all cases, this is listed at the top. It may be considered a “biological” failure. Loosening, fracture, and prosthetic breakage are grouped sequence as “structural failures,” and extensor insufficiency, stiffness, patellar tracking, and tibial-femoral instability are “kinematic” failures. Tibial-femoral instability is listed last, as any of the above can present with a report of buckling, instability, or giving way. Three modes of instability are listed in the columns to the right: 1. varus-valgus, 2. flexion, and 3. recurvatum
There is an unfortunate tendency to equate “instability” with ligament failure . This works in the assessment of native knee dysfunction after trauma or sports injuries but is a premature conclusion when a patient describes instability or “giving way” of a knee arthroplasty. Another unfortunate tendency is to plan revision surgery with a highly constrained prosthetic product whenever a patient reports instability. It is essential to identify why a knee joint is giving way and to correct the cause.
Instability is a symptom similar to pain: there can be many causes [11–13]. Indeed pain itself can be a cause of instability through “pain inhibition.” Any physical action that incites pain will be curtailed. The patient who steps off a curb and suffers anterior knee pain may experience quadriceps inhibition, with unopposed knee flexion and buckling. They might report that the knee “gives out,” and this may be misconstrued as a case of “instability.” A painful infected knee may buckle, especially if a large effusion prevents full extension. Pain may originate from an ipsilateral arthritic hip or quadriceps weakness from lumbar disease [14, 15]. These examples represent “functional,” secondary, or dynamic instabilities. They are not, strictly speaking, cases of knee “instability.” The source of pain requires attention, but the knee may not benefit from revision.
Not all knee arthroplasties that require revision will be cases of instability even if the patient describes the knee as “unstable.” The periprosthetic fracture is an example. Every surgeon would agree that a fracture makes the joint “unstable” but that the solution to the problem is neither ligament reconstruction nor necessarily a constrained implant, but rather fixation of the broken bone.
And so, a knee may lack stability due to a structural problem that should not be characterized primarily as instability. Other examples include instability from component loosening and bone loss or implant breakage [16]. These may require revision arthroplasty, but not necessarily with a highly constrained implant [17] (Fig. 14.4). Mechanical constraint is indicated specifically for ligamentous incompetence, not bone loss. Correction of the primary problem restores comfort, function, and stability by placing good ligaments under functional tension, thus avoiding the problems of constrained implants.


Fig. 14.4
(a–d) Loosening not true instability. This dramatic failure is Fig. 1 in a paper entitled “Revision knee arthroplasty with a rotating-hinge design in elderly patients with instability following total knee arthroplasty” [90]. Though the knee joint is clearly unstable, this is a case of component loosening with bone loss, (plus perhaps even infection), but it is not primarily a case of knee instability. In all probability stability could have been restored to this arthroplasty with a non-constrained device. Failure into varus, like this case, protects the medial collateral ligament, which is then available for reconstruction. The use of a constrained device can be defended if it was difficult to balance ligaments at the revision. This paper highlights the difficulty with definition of “instability.” True cases of instability result directly from failure of ligaments or are due to the size and positioning of the prosthesis. This distinction is important because it guides the reconstruction accurately and avoids unnecessary constraint (From Rodriguez-Merchan EC, Gomez-Cardero P, Martinez-Lloreda A. Revision knee arthroplasty with a rotating-hinge design in elderly patients with instability following total knee arthroplasty. J Clin Orthop Trauma. 2015;6(1):19–2, with permission)
True tibial-femoral instability might be described as: “failure to maintain the structure of the arthroplasty or failure to limit excursion of the joint under load as a result of failure (plastic deformation) of ligaments or deficiencies with component size and position that fail to place good ligaments under physiologic tension.” The two phenomena are related: a TKA with excess valgus alignment may overload the medial collateral ligament, which fails in tension. The cause of instability will be valgus alignment that results in medial soft tissue failure. Revision surgery must mitigate the cause (realign the knee) and then restore stability, perhaps with a constrained implant or, uncommonly, a ligament allograft [18].
Several modes of instability must be considered: (1) Varus-valgus, (2) plane of motion, and (3) flexion (Fig. 14.5). Varus-valgus instability (more frequently valgus) is the prototypical manifestation of the unstable TKA. Varus instability, for example, results from loads and an alignment that induce an insupportable bending moment (or torque) across the knee joint in the coronal plane. As the deformity increases, the moment arm of the load and the bending moment across the joint increase. Deformity and instability increase in a positive feedback loop until the knee collapses (Fig. 14.6).



Fig. 14.5
Modes of instability. True tibial-femoral instability of the knee can be identified in 3 basic forms, each of which has a different cause and requires a different approach at surgery. Some unstable arthroplasties of course may exhibit more than one mode, and they will require more complex techniques for reconstruction. On the left is the prototypical instability, in the frontal plane, also described as “varus-valgus” instability. It results from problems with tibial-femoral alignment, aberrant loading during gait, and failure of the collateral ligament envelope on medial and lateral sides. The middle is instability in the plane of motion. It is encountered less frequently and is often described as “buckling.” If this occurs because of patellar maltracking, then the case belongs in that category where attention to component rotation, etc. will be required (see Fig. 3, failure mode #7 “Patella and Malrotation”). If it occurs due to fixed flexion deformity and quadriceps fatigue, then the issue is stiffness (Fig. 3, mode 6). If it occurs due to recurvatum, then it is a true form of tibial-femoral instability, where the root cause is often extensor deficiency. The third mode is described on the right, instability of the knee in flexion, where generally the tibia subluxes posteriorly under the femoral condyles. This is a problem of flexion and extension gap mismatch

Fig. 14.6
(a–e): Moment arm – alignment and stability. (a) The effect of lever arm (redrawn from Einhorn et al. [93]). If a load of magnitude “F” is applied symmetrically to the top of a (nonelastic) column, that same load is eventually delivered through the column to its base. (b) If the load “F” is applied to this structure from above, a lever arm is created, with moment arm equal to “m.” This lever arm will increase as the angle between the two limbs of the “column” increases. The “action arm” will remain unchanged despite the angle. The torque or “bending moment” created at the apex will equal the moment arm times the force: m × F. (c) The lever arm can be redrawn to resemble a knee with a crude varus alignment. Now the line of action has been altered, and the moment arm is increased. (d) We must remember however that the force “F” is actually applied to the weight-bearing limb in stance phase from the center of gravity of the human body, generally in the front of the 4th sacral vertebra. This increases the moment arm and the bending moment on the “knee.” (e) Conversely, valgus alignment decreases this moment arm. Assuming stability in the articulation, this valgus alignment diminishes the destabilizing force at the articulation. (f) Varus instability, for example, results from loads and an alignment that induce an insupportable bending moment (or torque) across the knee joint in the coronal plane. As the deformity increases, the moment arm of the load and the bending moment across the joint increase. Load, pain, deformity, and instability increase in a positive feedback loop until the knee collapses
Loads across the knee are not symmetrically compressive as we might idealize from an anteroposterior radiograph (Fig. 14.7). The knee is usually subject to bending moments that create compression in one compartment and tension in the opposite collateral ligaments. Alignment and stability are both important to knee joint function. However, the unstable arthroplasty fails immediately, whereas the malaligned knee may take years to succumb to wear, osteolysis, and loosening. Stability is important because it controls alignment. The stable knee has one alignment (with minor physiologic deflections under load), but an unstable knee suffers many alignments, unfortunately always the worst one for a given load: a valgus load increases valgus alignment, increasing the destructive lever arm (Fig. 14.8).



Fig. 14.7
(a) In a simplified model, load applied to a rigid structure creates uniform compression. (b) Eccentric loads create compression and tension. (c) The monolith can be rendered as two columns, representing lower limbs. (d) In single stance phase, uniform compression is replaced by a strong bending moment, with compression on the media side and tension on the lateral side. (e and f) Varus alignment or aberrations in gait that might shift the application of load away from the affected joint increase the moment arm at the knee as well as the compressive and tensile loads. There will be a point at which structures fail and instability results. Alignment therefore is an important factor in maintaining or restoring stability. Conversely, valgus instability, observed frequently in knee arthroplasty, is exacerbated by loads applied from outside the lower extremity (scoliosis and coxalgic gait), and only the medial collateral ligament is available to resist tension—there is no counterpart to the dynamic muscular stabilization of the iliotibial band

Fig. 14.8
Stability and alignment. Stability means that ONE alignment angle will be maintained. Instability means that there are many alignment angles—always the worst one for the load applied. As the knee is loaded in varus, the alignment shifts to maximum varus and the destructive lever arm is maximized. Unfortunately, the arthroplasty literature studies alignment and stability separately. When studies report “alignment,” they rarely evaluate whether the arthroplasty was actually stable and vice versa
Physical activity loads the knee unpredictably. Surgical alignment can modulate the moments across the knee joint, but only statically and not in response to loading. If we lean to the left side, for example, the left knee cannot go “into varus” to reduce compression in the lateral compartment. By contrast, the extensor mechanism can respond to greater load with more forceful contraction or by changing the flexion angle. Less flexion requires less power from the quadriceps for stability. A person carrying groceries avoids walking in a squat.
Two sides of the stability equation must be considered: mitigation of deforming loads and restoration of stabilizing forces. Limb alignment, gait abnormalities, and spinal deformity (scoliosis) may contribute to knee joint overload and instability. Failure to correct valgus deformity in the arthritic knee combined with failure to substitute for an incompetent medial collateral ligament predictably results in instability. Similarly, failure to equalize the lengths of the medial and lateral ligaments, (balancing the knee) after correction of deformity, results in instability. A constrained implant alone is not a complete solution to the problem of instability. Although the majority of arthroplasties function very well, the ideal alignment for any given knee joint, much less all knee replacements, is difficult to specify [19]. Consider how loading changes in a typical knee with the usual anatomic valgus alignment of five degrees if thigh girth is increased, resulting in a wider stance (Fig. 14.9). Though we may not be able to predict the ideal alignment, we might draw useful conclusions regarding alignment for a revision if varus or valgus instability had been the reason for failure of the primary.


Fig. 14.9
Thigh girth . (a) Short radiographs can only depict anatomic alignment: the angle between the shafts of the tibia and femur. This is an incomplete depiction of the loads across the joint. (b) Full-length bilateral standing radiograph of patient with bilateral valgus deformities, predominantly lateral compartment osteoarthritis on the right knee, and a valgus instability of the primary total knee arthroplasty on the left knee. Dotted lines depict the mechanical axes of the limbs as lines from the center of hips to the center of ankles. These pass outside the arthritic joint and through the lateral compartment of the arthroplasty. Radiographs and simple angles do not depict the loading pattern in detail. (c) Note the dotted line depicting the skin contour of each medial thigh. These are overlapping as the radiographer has forced the patient to include both limbs on one cassette. This is clearly not how the legs are positioned as the patient walks: the feet must be separated further, stance widened, and the distance from the ankle to the center of gravity of the body will increase. This increases the bending moment across the knee. To maintain balance, many patients like this will shift their center of gravity over the affected limb with each step, accelerating the torque across the knee. (d) If we modify the radiographic image by abducting the right limb at the hip so that skin contours can clear each other during swing phase, we appreciate the necessary width of stance and the bending moment on the right knee in valgus. (e) Rather than planning the right knee arthroplasty at a standard angle, in this obese patient, it seems that a very few degrees of valgus (stippled box) might establish a more reasonable stance width (foot separation) and a decreased lever arm across the joint. Diminished valgus alignment raises concerns about the risk of medial overload and loosening. However, we must remember that the patient with osteoarthritis and valgus deformity is at greater risk of failure from instability than loosening [94]
Plane of Motion Instability
“Plane of motion stability” depends on the relationship of the static stabilizing structures in the back of the knee and the extensor mechanism in the front. The posterior structures limit hyperextension, and the quadriceps controls spontaneous flexion (buckling). If the quadriceps muscle cannot resist loads across the knee joint, flexion occurs without control.
Lack of quadriceps control may be apparent in the physical examination as an inability to extend the knee fully, even when the joint is capable of full extension (extensor lag). Disruptions of the extensor mechanism anywhere from the quadriceps muscle to the tibial tubercle create gross extensor insufficiency. Quadriceps atrophy, denervation, and general deconditioning may be responsible for functional extensor weakness. The patient with a patellectomy or a thinned patella from a prior arthroplasty may suffer relative extensor insufficiency due to loss of mechanical advantage despite a strong muscle [20]. These situations are similar in their effect on stability.
Patients with a deficient, weak, or dislocating extensor mechanism often compensate by locking the knee in full extension or hyperextension, during stance phase. This was first recognized in polio patients who lack quadriceps strength. Similar compensation may be observed in the obese patient whose extensor mechanism is close to normal strength but incapable of supporting increased body weight: they tend to walk with a stiff knee. Patients with patellofemoral pain, especially if obese, also limit knee flexion with a “patellar avoidance gait” that reduces anterior knee pain [21]. They may develop severe arthritis without a fixed flexion contracture because of this gait pattern. Eventually, just like the polio patient without L4 anterior horn cell function, the posterior soft tissues stretch and recurvatum ensues.
Patients with recurvatum instability as the result of discontinuity or attenuation of the extensor mechanism are best considered for revision arthroplasty as cases of “extensor mechanism deficiency,” not instability. The solution to the cause of trouble is restoration of extensor strength, often with an extensor mechanism allograft [22]. Neurologic compromise of the quadriceps with recurvatum instability has traditionally been considered a contraindication to arthroplasty surgery [23] and more reliably treated with arthrodesis. Linked constrained implants (hinges) that allow the knee to lock in slight hyperextension have been recommended for the polio patient [24]. Once the “hyperextension” stop in the prosthesis engages, however, the forces on the implant and its fixation are high: the lever arm resembles a common crow bar. While short-term success is feasible, later failure may be catastrophic. If hyperextension is completely eliminated with any prosthesis in these patients, the knee will tend to buckle. A prosthesis may replace the joint, but it cannot provide extensor power.
Just as instability from extensor deficiency is a failure mode distinct from “tibial-femoral instability,” patellar maltracking is also separate. Maltracking, though it may cause buckling and instability, is generally the result of combined internal rotation position of the tibial and femoral components [25–27]. The solution to patellar maltracking is a revision that corrects the rotational position of the components.
Fixed flexion deformities, though seemingly the converse of recurvatum, may also result in “instability” or buckling. The patient who cannot fully extend the knee becomes exhausted walking with a crouched gait as they expend more energy [28]. Many elderly patients experience this problem [29], especially if kyphosis requires flexion at the hip and knee to maintain the body’s center of gravity over the feet (Fig. 14.10).


Fig. 14.10
Posture and knee stability . (a) Schematic depiction of youthful posture. Physiologic lumbar lordosis and thoracic kyphosis lead to a nicely balanced torso, with weight centered over the feet. (b) Increased thoracic kyphosis obliges other changes in posture to maintain balance. Notably knee flexion shifts the center of gravity posteriorly, restoring balance, but also increasing the risk of knee buckling. Surgery to correct a knee flexion contracture in this setting is likely to fail as flexion is essential to balance
Restoring full extension is an important goal in revision knee arthroplasty. However, a flexed knee posture will recur in some patients with multiple balanced contractures, for equilibrium. These individuals should probably not undergo revision if a fixed flexion contracture is the only knee problem, even if they report the joint as unstable. The patient with good function in other joints, whose knee arthroplasty is buckling due to a flexion contracture and muscle fatigue, should be considered in the category of the “stiff knee” as this prescribes the appropriate surgical techniques to restore function.
Meding and colleagues reviewed arthritic knees with recurvatum deformities prior to primary arthroplasty. They emphasized the importance of identifying recurvatum due to neurological deficiency and eliminating residual hyperextension at arthroplasty surgery [30, 31]. These principles apply to the failed arthroplasty with recurvatum instability. Surgical techniques have been described to correct non-neurologic recurvatum with primary arthroplasty [32]. This deformity often results from subsidence of a loose (femoral) component, such that the posterior structures are no longer under tension. Restoring the length of the posterior soft tissues by compensating for bone loss with revision surgery is generally successful.
Flexion Instability
Flexion instability describes a TKA with a flexion gap that is larger or more lax than the extension gap, where the modular polyethylene insert has been selected to stabilize the extension gap at the expense of the flexion gap [33]. A larger insert in these cases would limit extension and require treatment for stiffness i.e. flexion contracture. Flexion instability is not generally the result of an incompetent posterior cruciate ligament, as one might expect in the non-arthroplasty knee.
The problem was first described in 1998 by surgeons at the Mayo Clinic [34]. The clinical presentation includes (1) a sense of instability without frank giving way, (2) recurrent knee joint effusions, (3) soft tissue tenderness involving the pes anserine tendons and the retinacular tissue, (4) posterior instability with a positive posterior drawer (not apparent with posterior stabilization) or a posterior sag sign at 90 degrees of flexion, and (5) above average motion. They reported 22 cases revised to posterior-stabilized implants and three cases where only the polyethylene insert was changed. Nineteen of the twenty-two (86%) revisions were improved, but only one of the poly exchanges was better off after surgery. The authors concluded that a “revision operation that focuses on balancing the flexion and extension spaces, in conjunction with a posterior-stabilized knee implant, seems to be a reliable treatment for symptomatic flexion instability after posterior cruciate retaining total knee arthroplasty” [34]. An isolated modular polyethylene exchange, because it tightens both the flexion and extension gaps equally, would not be expected to correct the problem unless it were actually a case of global instability and not “flexion instability.”
Comprehensive evaluation is necessary in any case that presents with symptoms of flexion instability, to ensure that all components are well fixed, correctly sized, and appropriately rotated before recommending an isolated revision of the femoral component to a larger size as a direct means of tightening the flexion gap. A simple technique for balancing gaps in any revision surgery is described below.
The same investigators later reported that flexion instability can complicate posterior-stabilized prostheses [35] highlighting the importance of surgical technique over implant design. Nine of ten revisions for flexion instability in that series were successful. The logical emphasis was placed on implanting larger femoral components. In concept, however, the same sized femoral component might be repositioned more proximally, with additional resection of distal femoral bone, to increase the size of the extension gap such that a thicker polyethylene could stabilize the knee in flexion. The joint line position would be altered, but the complication eliminated. This approach is useful, because in some cases of flexion instability, the femoral component will already be maximally large, i.e., extending from the medial to the lateral cortex. A larger component would overhang the distal femur. The etiology of flexion instability in some cases may be an uncorrected flexion contracture, not an incorrect femoral component size (Fig. 14.11).


Fig. 14.11
Flexion instability. (a) The patient with arthritis and a knee flexion contracture actually has a smaller, tighter “extension gap” than a person with a normal knee joint. (b) If total knee arthroplasty is performed with a typical “measured resection” type of technique, the relatively tightness of the extension gap can be appreciated by surgeons who look for it. (c) The flexion gap is actually “normal” but correspondingly larger and more lax than the extension gap. This is probably the typical origin of “flexion instability,” when the surgeon fails to appreciate the flexion-extension gap discrepancy and selects the articular polyethylene to achieve full extension
Arthritis with a fixed flexion contracture is perhaps the most common condition leading to flexion instability [33]. If a surgeon performs the primary arthroplasty in this situation with a measured resection or “kinematic” style procedure, but without soft tissue releases, the extension gap will end up smaller and tighter than the (more normal) flexion gap. If the surgeon then selects the modular polyethylene that permits full extension without a critical evaluation of stability at 90°, flexion instability is likely. While some low-demand patients may function reasonably well with a lax flexion gap, enjoying easy flexion, more active individuals will experience pain with activity, difficulty on stairs, recurrent effusions, and periarticular tenderness.
Surgeons from the Mayo Clinic, who originally identified flexion instability, recently published an algorithmic approach to the problem. They recommend: “reduction of tibial slope, correction of malalignment, and improvement of condylar offset. Additional joint line elevation is needed if the above steps do not equalize the flexion and extension gaps” [36]. Elevation of the joint line results directly from resection of distal femoral bone to increase the size of the extension gap so that thicker polyethylene can be used to eliminate flexion instability. Joint line position is subservient to balancing the flexion and extension gaps in their analysis. This is a highly reasonable and practical approach.
More severe cases of flexion instability may result not just in pain, swelling, and tenderness but frank dislocation, usually of the flexed tibia posteriorly under the femur. The mechanisms are the same, but the discrepancy in the relative sizes of the flexion and extension gaps is greater. In some cases there will have been a profound mismatch between an arthritic deformity, the design of the implant, and the surgeon’s skills: the wrong surgeon implanting the wrong implant into the wrong patient [37]. Dislocation may be exacerbated in patients with spasticity of the hamstring muscles [38, 39].
Anterior dislocation, of the tibia under the femur, is unusual and generally accompanies extreme recurvatum deformity [40, 41]. Late onset cases generally result from polyethylene wear, not ligamentous failure [42, 43]. Some grossly unstable arthroplasties may be held together by the extensor mechanism, which when it ruptures results in knee dislocation [44]. These should not be interpreted as isolated extensor mechanism ruptures and require full revision with an extensor mechanism allograft [22]. Arthrodesis may be preferred.
The clinical presentation of a posterior-stabilized arthroplasty with the tibial spine dislocated under the femoral cam in flexion will be dramatic, although the lateral radiograph will show only posterior subluxation of the tibia. The patient will be in great pain with a knee locked at close to 90 degrees of flexion [45–50]. These can usually be reduced with hyperflexion and an anterior drawer maneuver to the proximal tibia. Forced extension is a mistake. General anesthesia may not be required, and recurrent or irreducible dislocations will require surgery for flexion instability.
Mid-flexion instability is a contentious entity. Whether it is distinct from either “flexion” or coronal instability is unclear. One cadaver study associated joint line elevation in the primary arthroplasty with instability in the midrange of flexion [51]. Though frequently quoted, this study is not the final verdict [52, 53]. There are no published reports of revision surgery for “mid-flexion” instability.
Clinical Evaluation of the Unstable TKA
There are numerous methods to evaluate a problem arthroplasty prior to surgery. The best will be systematic , following the same sequence of steps or algorithm for every case, and comprehensive , meaning that even if one problem is identified, all others are still considered. A common example of multiple problems presenting as one would be loosening resulting from infection. If only loosening is diagnosed, revision surgery will fail. Comprehensive evaluation of gross clinical instability may reveal that the actual cause is loosening and bone loss, perhaps caused by infection.
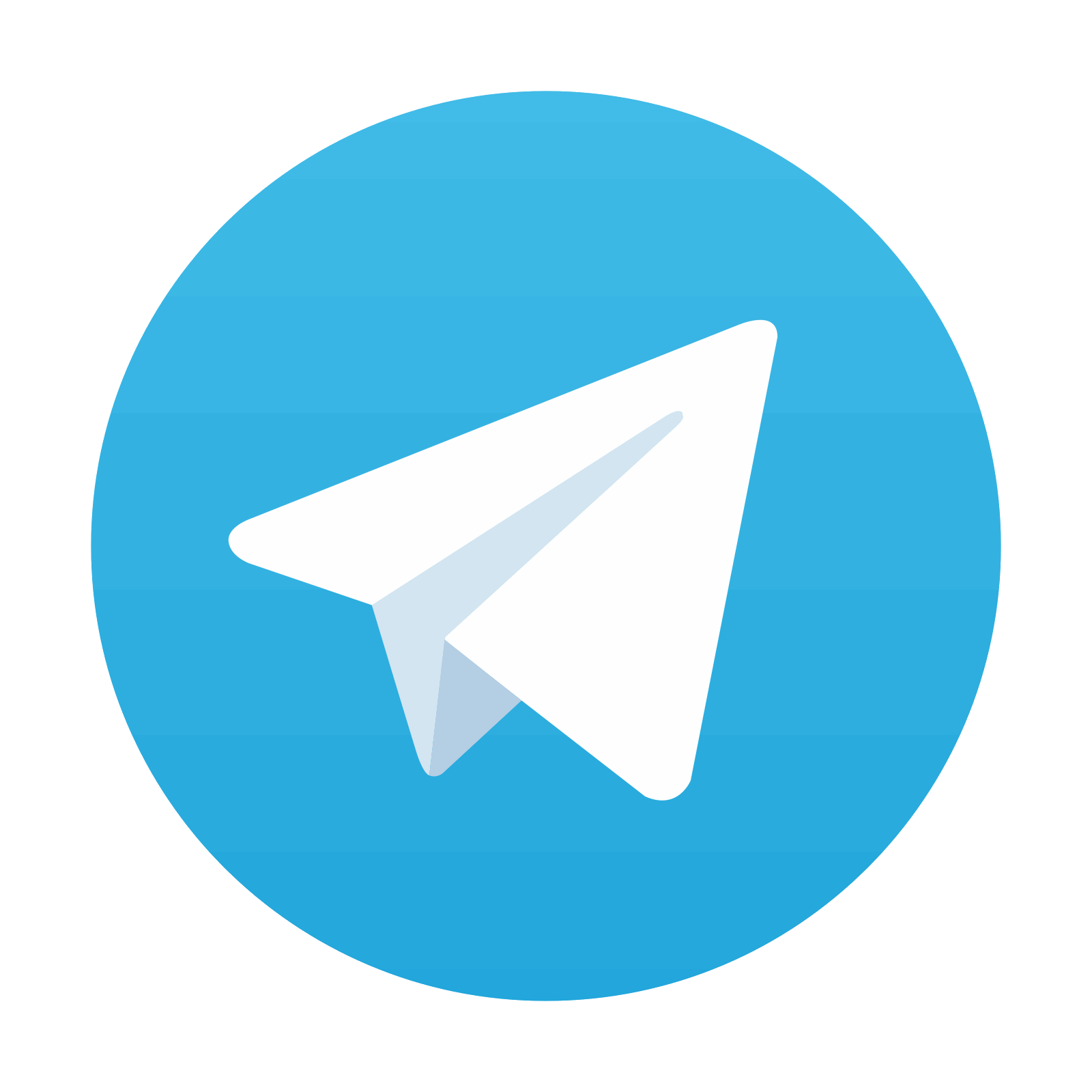
Stay updated, free articles. Join our Telegram channel

Full access? Get Clinical Tree
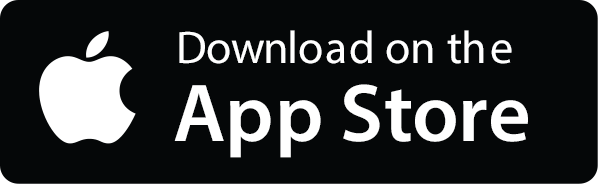
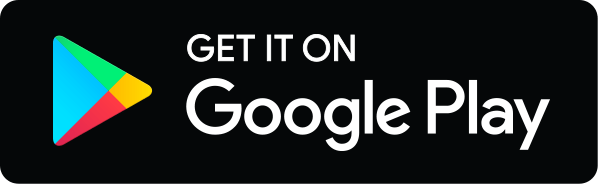