This article provides a summary of clinical assessment methods and nonpharmacologic rehabilitation techniques used for concussed patients. It describes concussion-relevant physical examination methods to identify underlying symptom generators. This approach allows practitioners to prescribe targeted rehabilitation therapies to treat postconcussion symptoms. Evidence-based rehabilitation approaches include cervical rehabilitation, vestibulo-ocular rehabilitation, and sub–symptom threshold aerobic exercise.
Key points
- •
Older guidelines for sport-related concussion (SRC) recommended strict rest until symptom resolution. However, emerging research is identifying causes for many post-SRC symptoms based on the predominant symptom generators identified on physical examination.
- •
The most recent International Concussion in Sport Group guidelines recommend a multidisciplinary team approach to SRC treatment. This article introduces clinicians to a systematic approach to SRC assessment and treatment based on a pertinent physical examination.
- •
The physical examination assessment of SRC for the purposes of guided physical rehabilitation is focused on the autonomic, ocular, vestibular, and cervical subsystems and, where indicated, assessment of exercise tolerance. These clinical profiles rarely exist in isolation and often overlap.
- •
The approach to SRC assessment and treatment is undergoing a paradigm shift. This article presents the current evidence base to inform best practice by providers.
Introduction
Most adult patients with sport-related concussion (SRC) recover spontaneously within 7 to 14 days, whereas adolescents may require up to 4 weeks to recover; however, some take significantly longer. SRC is a complex disorder that the most recent Concussion in Sport Group (CISG) international guidelines state is one of the most challenging diseases to diagnose and manage. There is no gold-standard treatment of SRC so treatment modalities must be individualized based on the predominant symptom generators. It is important to identify the cause for SRC symptoms because they can vary and evolve over different time frames. Some investigators have categorized SRC into the following clinical profiles: physiologic, cervicogenic, vestibulo-ocular, and mood related. , Clinically, these profiles rarely exist in isolation and often overlap. Fig. 1 summarizes the nonpharmacologic treatment modalities for each SRC clinical profile, which are described in detail later. This article introduces clinicians to a systematic approach to SRC assessment and evidence-based, nonpharmacologic rehabilitation. Pharmacologic therapies are discussed (See article “ Medical Therapies for Concussion ” by Jacob C. Jones and Michael J. O’Brien) in this issue. There are no pathophysiologic findings specific to mood-related SRC. The management of this clinical profile is challenging because of the extensive overlap with symptoms of preexisting primary mood disorders, and treatment involves symptom-specific pharmacologic and nonpharmacologic therapies. The CISG guidelines recommend a multidisciplinary team approach to treatment. Assessment and treatment of the neuropsychological aspects of SRC is also discussed elsewhere in this issue. Posttraumatic headaches are also common after SRC; the medical management of these is discussed elsewhere in this issue.

Assessment
The physical examination assessment of SRC for the purposes of guided physical rehabilitation is focused on the ocular, vestibular, and cervical subsystems and, where indicated, assessment of exercise tolerance. A recent study showed that early assessment and intervention for the clinical profiles of SRC resulted in better outcomes versus delayed evaluation.
Assessment for Ocular Dysfunction
The incidence of oculomotor dysfunction after mild traumatic brain injury (mTBI) can be substantial, with some investigators estimating it to be present in up to 70% of patients. However, the incidence has been reported to vary from 9% to 90%. The variability stems from the sensitivity of the measurement tools used to identify ocular dysfunction. Impairments in smooth pursuits, saccades, alignment, vergence (convergence and divergence), and accommodation have been reported, which can be detected using standard physical assessment techniques. After taking a history, including mechanism of injury, a thorough binocular functional vision examination should be completed that highlights eye tracking skills (smooth pursuits and saccades) and eye teaming/focusing skills (vergence and accommodation).
Eye tracking smooth pursuits
Eye tracking smooth pursuits can be examined with a simple H, I, or X test where a target is moved slowly in the formation of the letter and the examiner observes for any sustained nystagmus (fatigable nystagmus is normal), difficulty with gaze holding, and accuracy.
Eye tracking saccades
The patient is asked to visually track an object moving slowly in the horizontal direction with the head stationary. Target movement should be limited to 30° from the midline to avoid eliciting end-gaze nystagmus. Abnormal is sustained nystagmus, staccatic (or jerking) eye motion, loss of conjugate vision, corrective (catch-up or backup) saccades, loss of visual fixation, or symptom provocation (dizziness, nausea, or headache).
Repetitive saccades
The examiner holds both index fingers 0.5 m apart at half an arm length from the patient. The patient is instructed to move the eyes side to side in rapid succession in the horizontal plane, rapidly switching focus between the examiner’s 2 index fingers. The test may be repeated in the vertical plane as well. Abnormal responses include delayed initiation of eye movement, slow velocity, or inaccurate movements such as overshooting/undershooting with greater than 1 refixation saccade. Abnormal movements or symptom provocation qualify as abnormal responses. Patients are considered to have healthy function when they can do 20 repetitions with normal velocity and accuracy without eliciting symptoms of headache, dizziness, or nausea. This test in the healthy population can sometimes elicit eye strain but should not provoke symptoms of increased headache or dizziness.
Eye teaming/focusing
Eye teaming skills are necessary for depth perception to coordinate eye movements as objects move closer to or further away from the person. Vergence is the simultaneous movement of both eyes in opposite directions to obtain or maintain single binocular vision as objects move toward (convergence) or away from the person (divergence). Near point convergence (NPC) can be measured by placing a standard accommodation ruler at the forehead just above the patient’s nose. Starting at a point where the patient can easily focus on the target (20–30 cm from nose), the patient is asked to focus on the target as it is moved slowly toward the face. As soon as the patient reports splitting of the target in 2, the NPC distance is recorded to the nearest 0.5 cm. Ideally, this should be performed 3 times and the better recording reported. This distance should be less than 10 cm in the general population, and less than 6 cm in children and adolescents. Note that the NPC is not taken where the target blurs but where it splits. Accommodation (monocular vision) is also measured to the nearest 0.5 cm using the same ruler and is acquired over 2 trials for each eye. The patient is instructed to cover 1 eye and, starting at the furthest distance away from the nose, the target is moved slowly toward the face. Accommodation distance is determined when the patient reports blurring (not doubling) of the image. A value of greater than or equal to 12 cm is considered to be abnormal.
A more objective and thorough assessment of ocular function is possible using computerized eye tracking software. This technology, which includes a combination of high-speed cameras and infrared sensors, evaluates predictive attention performance and synchronization in the spatial and temporal domains, both of which can be impaired after SRC.
Assessment for Vestibular Dysfunction
SRC often produces symptoms of dizziness, headache, nausea, visual motion sensitivity, vertigo, feeling disconnected from the environment, and/or feeling off balance. There is significant interaction of the vestibular and oculomotor systems; for example, intact binocular visual function is essential to normal vestibular function, so the symptoms of injuries to both often overlap or reinforce one another. It is important to remember that dynamic vestibular examinations should not be performed until significant cervical dysfunction has first been ruled out or treated. It is thought that most vestibular dysfunction after SRC is central (brainstem or cerebellar), although peripheral injury can on occasion be observed.
Vestibulo-ocular reflex
The vestibulo-ocular reflex (VOR) integrates the vestibular and oculomotor systems by testing the ability to keep an image centered on the fovea during horizontal and vertical head motion. The patient is asked to maintain visual fixation on the examiner’s finger located directly in the frontal-central field of vison, held approximately 30 cm from the nose, while rotating the head as rapidly as possible for at least 10 complete turns. Inability to maintain visual fixation (ie, beating back to the center) and/or symptom provocation of headache, dizziness, or lightheadedness is abnormal. The VOR is often impaired after SRC so that head movement at speeds necessary (≥2 Hz) for daily activities causes dizziness, nausea, or blurred vision. , The head thrust maneuver is another method to assess VOR dysfunction. It involves a rapid twisting motion of the neck rather than active patient-initiated motion, so it should be performed only after a significant cervical injury has been ruled out by an experienced practitioner.
Dynamic visual acuity
Static visual acuity measured with a Snellen or handheld eye chart provides a baseline of visual acuity without head motion. Using the same eye chart, the patient is instructed to read the lowest line on the chart while performing horizontal head rotation at greater than or equal to 2 Hz. The difference in line numbers on the eye chart between stationary (static) versus head motion (dynamic) should be no more than 1 to 3 lines for the healthy population, and less than 2 lines in higher-level athletes. Visually complex environments are more difficult to maintain a functioning VOR; therefore, the dynamic visual acuity (DVA) should be tested in a calm environment.
Static and dynamic balance
Static balance is assessed by tandem stance. The patient puts hands on the hips and 1 foot is planted directly in front of the other, attempting to hold it for 20 seconds with eyes closed. Dynamic balance is assessed using tandem gait, eyes open and closed. The patient is asked to walk in a straight line for 5 steps eyes open, heel to toe, hands at the side, while looking straight ahead at a fixed point on the wall. The patient then continues for 5 steps with eyes closed. The patient then walks backward with eyes open, toe to heel, along the same line for 5 steps. The patient then repeats this with eyes closed. Inability to walk the line, stumbling, or stepping out of line while walking forward, backward, or during tandem stance is abnormal. More objective methods of assessing balance include adding a dynamic component as well as a cognitive dual task. Balance and gait assessment technologies, which are more reliable than clinician-performed techniques, are also available but require specialized equipment and may be expensive.
Less often, athletes injure the peripheral vestibular system when they sustain a concussion. In contrast, they may have an isolated vestibular injury without concussion, such as benign paroxysmal positional vertigo (BPPV). BPPV is assessed through a variety of head and body positions, most commonly the Dix-Hallpike maneuver. In addition, proper balance assessment must include a cervical examination (described later). The postural control system relies on multiple inputs from the somatosensory/proprioceptive organs in the extremities and the cervical muscles and joints, as well as from the vestibular and visual systems. This complex interplay allows people to maintain head and body position, perceive motion, stabilize gaze, control eye movements, and orient themselves in space. Cervical injury affects cervical afferents and the proprioceptive system of mechanoreceptors, including muscle spindles, joint position receptors, and Golgi tendon organs, which can cause dizziness of cervical origin, called cervicogenic dizziness. Unlike vertigo, which is typically associated with a spinning or motion sensation, cervicogenic dizziness is commonly described as feelings of disorientation and unsteadiness exacerbated by neck motion. Injuries to the cervical spine and associated myofascial pain often cause restricted cervical motion, which can further cause feelings of unsteadiness.
Assessment for Cervical Dysfunction
There are numerous structures in the neck that may generate pain following a concussive head injury, including the cervical zygapophyseal (facet) joints, bony structures, parascapular muscles, ligamentous structures, and nerve entrapments. The C1 nerve (suboccipital nerve) provides innervation to the atlanto-occipital joint, with pain commonly referred to the posterior occiput. The C2 nerve passes in close proximity to the atlantoaxial joint and innervates it and the C2-C3 zygapophyseal joint. The C3 nerve also innervates the C2-C3 zygapophyseal joint, which can refer pain to the frontotemporal and periorbital regions of the head, and is thought to be involved in up to 70% of cervicogenic headaches.
The evaluation of neck pain following SRC should include a comprehensive history and a physical examination. A detailed history of neck pain is important because different cervical disorders cause different patterns of pain specific to the particular muscles that are disrupted through the biomechanics of the injury. These pain syndromes are treated with a combination of focused physical therapy strengthening exercises, manual therapy, myofascial release, and/or trigger point injections individualized to the disorder. Cervical physical examination includes palpation and range-of-motion assessment. A palpatory examination should evaluate the rhomboids, trapezii, infraspinatus, supraspinatus, and cervical paraspinal musculature, with a particular focus on the splenius cervicis and splenius capitis, because both are common myofascial pain generators. Range of motion includes flexion, extension, lateral rotation, and lateral flexion of the neck. The strength of both the sternocleidomastoids and the shoulder elevators (trapezii) should be noted, because both reflect the integrity of the spinal accessory nerve (cranial nerve XI). Listening for carotid or cavernous bruits is warranted in some cases.
One of the primary objectives of cervical assessment is to screen for any red flag signs or symptoms that warrant further evaluation. These signs include myotomal weakness or sensory loss in a dermatomal pattern, change in bowel/bladder function, or the presence of the Spurling or Lhermitte sign, all of which may indicate the presence of a radiculopathy or myelopathy. The Spurling test for cervical radiculopathy is best viewed as confirmatory rather than as a screening test because of its high specificity and low sensitivity. In patients with cervical instability, these tests should be performed with caution because of the provocative stress required. The presence of the Hoffmann reflex may suggest an upper motor neuron lesion in the brain, brainstem, or cervical spinal cord, although it may be a normal finding in a subset of healthy patients who are generally diffusely hyper-reflexic. , A positive Wallenberg (vertebral artery test) test may indicate vertebrobasilar insufficiency and warrants further investigation.
Cervical posttraumatic disorders
Injuries to the cervical spine may occur in patients who sustain head trauma, with or without a concussion. The same acceleration-deceleration forces that are transmitted to the brain can be transferred to the neck, causing excessive extension-flexion of the neck and concomitant whiplash injury. Injuries to the cervical spine include sprain-strain and joint injuries that can cause symptoms similar to those of SRC.
Cervical strain and sprain injuries
Cervical strain and sprain are the cause of most acute neck pain after whiplash injuries. Cervical strain refers to injury to the muscles or tendons, whereas cervical sprain refers to overstretching or tearing of the spinal ligaments. Acceleration-deceleration forces can cause the cervical spine to take on an S-shaped curvature. As the neck flexes forward, the posterior neck extensors are prone to injury as they eccentrically contract to decelerate the head. This contraction can strain the levator scapulae, superior trapezius, sternocleidomastoid, scalene, and suboccipital muscles. The anterior longitudinal ligament merges with the intervertebral disc, injury to which can be associated with injuries to the cervical disc. Patients with cervical strain and sprain injuries may have tension-type headaches typically described as a dull, pressure pain located on both sides of the head. On examination, there may be pain to palpation of the muscles with limited range of motion and muscle guarding. When clinically warranted, radiographs of the cervical spine with flexion and extension views may be obtained to evaluate for bony abnormalities or to rule out acute instability before initiation of treatment.
Cervical myofascial pain syndrome
Myofascial pain syndrome refers to pain generated from a palpable taut band of hypercontracted muscle fiber called a myofascial trigger point that causes referred pain. Myofascial trigger points are categorized as active if they cause pain spontaneously, or latent if they are painful only on palpation. The underlying mechanism that causes myofascial trigger points is unknown but is thought to involve muscular overuse or overload. Pain is often described as dull, deep, diffuse, aching, or a soreness. As mentioned earlier, physical examination should focus on palpation of rhomboids, trapezii, infraspinatus, supraspinatus, and the cervical paraspinal musculature, particularly the splenius cervicis and splenius capitis, because these are all common myofascial pain generators.
Cervical-joint pain
After a whiplash-type injury, the cervical zygapophyseal (facet) joints have been shown to be among the most common sources of pain. Reports indicate that up to 60% of cases of posttraumatic neck pain involve the facet joints alone or in combination with injury to the cervical intervertebral discs. Other studies have shown that 58% to 88% of patients with chronic facet joint pain complain of cervicogenic headaches. Branches of the C1 and C2 ventral rami innervate the atlanto-occipital (C0-C1) and atlanto-axial joints (C1-C2), whereas medial branches from the cervical dorsal rami innervate the cervical facet joints. Injury to the facet joints during a whiplash injury is likely secondary to excessive compression of the facet joints and capsular ligament sprain. Cervical facet joint–mediated pain is commonly described as dull and achy with particular referral patterns to the head, neck, and periscapular regions. Pain from the C1-C2 joint tends to refer to the occipital and suboccipital region but can also be referred to the vertex, orbit, and ear. Pain from C2-C3 facet joints refers to the occipital region, but can also spread to the parietal, frontal, and orbital regions. Pain from C3-C4 and C4-5 facet joints more commonly refers to the upper and lateral cervical region. The C5-6 facet joint produces pain over the supraspinatus fossa of the scapula, whereas C6-C7 facet joints typically produce pain over the scapula. However, there are no clinical examination findings that are diagnostic specifically for cervical facet joint pain.
Cervical disc pain
Cervical intervertebral discs can be injured in conjunction with the facet joints. There can be internal disc disruption, which is damage of the internal nucleus pulposus or annular fibers without evidence of external deformation. More significant injury can lead to cervical disc herniation, which can be described as protrusion, extrusion, or sequestration. Patients complain primarily of axial, bilateral paravertebral, occipital, or scapular pain that is exacerbated by activities that increase intradiscal pressure, such as prolonged sitting, coughing, sneezing, or lifting. When examining patients, it is always important to do a thorough neuromuscular examination to exclude myelopathy or radiculopathy. It is notable that many disc abnormalities seen on imaging are not associated with pain or physical examination findings; they reflect the normal aging process of the cervical spine.
A 2016 Delphi study by Luedtke and colleagues concluded that the most useful techniques used by physical therapists for patients with headache include the craniocervical flexion test, cervical flexion rotation test, active range of cervical movement, trigger point palpation, muscle tests of the shoulder girdle, passive physiologic intervertebral movements, thoracic spine screening, and combined movement tests. The cervical flexion rotation test, smooth-pursuit neck torsion test, and cervical-joint reposition error test have been shown to have high reliability and strong diagnostic accuracy for diagnosing cervicogenic headache, which is often a comorbid contributor or sole cause of headache in this patient population. These tests attempt to minimize visual and vestibular factors while targeting cervical position and movement-sensory information to help isolate cervical disorder. The cervical-joint reposition error test identifies damage to muscle spindles in the neck, whereas the smooth-pursuit neck torsion test is used to identify cervico-ocular disturbances. The right and left alar ligament tests assess the integrity of the upper cervical spine using lateral flexion, which has been validated to significantly increase the length of the contralateral alar ligament. The transverse ligament integrity of the cervical spine is assessed using the Sharp Purser test. ,
Assessment for Exercise Tolerance
Exercise intolerance, the inability to exercise to age-appropriate maximum because of exacerbation of concussion-like symptoms, is a characteristic of SRC. This exacerbation is suspected to be caused by abnormalities in the autonomic nervous system that lead to abnormal cerebral blood flow (CBF) regulation. Exercise intolerance is associated with symptoms such as cognitive fatigue, headaches, and balance problems. The degree of exercise intolerance can assist in identifying symptom generators after SRC. Early exercise intolerance (defined as symptom exacerbation at <70% of age-appropriate maximum heart rate) is characteristic of autonomic/physiologic dysfunction in SRC, which is thought to reflect abnormal autonomic control of CBF during exercise.
The safety of exercise tolerance testing after SRC has been assessed in several studies. The Bruce treadmill protocol was used to safely assess exercise tolerance within 5 to 7 days of SRC, and the degree of exercise intolerance was associated with increased recovery time. Similarly, the McMaster All-Out Progressive Continuous Cycling Test was used to assess exercise tolerance after mTBI. Although there was an increase in symptoms on the day of the test, patients improved significantly within 24 hours, and temporary symptom exacerbation was not associated with negative outcomes. In addition, in a randomized controlled trial (RCT), Leddy and colleagues assigned adolescents within 10 days of SRC to perform the Buffalo Concussion Treadmill Test (BCTT) or not and tracked symptoms daily for 2 weeks. There was no significant difference in daily symptoms or recovery duration between the 2 cohorts. Thus, exercise testing is safe to perform in the acute period after SRC provided the patient is not pushed past the point of significant symptom exacerbation. This study also showed that greater early exercise intolerance (ie, the lower the heart rate at symptom exacerbation on the BCTT) was associated with longer duration of recovery. This relationship was subsequently validated in 3 separate cohorts by Haider and colleagues, providing further evidence for the usefulness of exercise tolerance testing. The BCTT is the preferred exercise tolerance assessment protocol because it has been designed for patients with concussion, and its safety and reliability have been validated in patients with SRC. There is now a cycle ergometer version of the BCTT, the Buffalo Concussion Bike Test, which is recommended for patients with orthopedic injuries or significant balance dysfunction that may prevent safely walking on a treadmill.
Considerations for exertion testing
Before ordering any exertion test, patients must be screened for contraindications to exercise, including significant orthopedic or cervical injury, balance problems, or cardiovascular issues. A complete list of contraindications to exertion testing is provided in the American Heart Association guideline for exercise testing. Patients should wear a reliable heart rate monitor because it is important to know the threshold heart rate when symptoms increase. Any increase in concussion-like symptoms is abnormal, defining exercise intolerance. Significant exercise intolerance to terminate the test occurs when symptoms increase by 3 or more points from baseline (a point or more given for symptom increase or appearance of a new symptom). Termination of the test because of voluntary exhaustion, or achievement of 85% to 90% of age-appropriate maximum heart rate, defines normal exercise tolerance. It is hypothesized that early exercise intolerance (test termination because of symptom exacerbation at <70% of age-predicted maximum) is characteristic of physiologic dysfunction from concussion and portends a worse prognosis. , Exercise cessation beyond 70% of age-predicted maximum heart rate is consistent with near or complete recovery from global metabolic dysfunction, or with patients whose symptoms have been identified to emanate from a cervical or vestibulo-ocular disorder.
Rehabilitation
Multimodal sub-system impairment-based therapies that are individualized to the patient, including oculomotor, vestibular, and cervical therapies, as well as sub–symptom threshold aerobic exercise, have been associated with a reduced incidence of persistent symptoms. , Most impairments in the vestibular and ocular systems recover spontaneously without treatment; however, if these impairments do not resolve by 2 weeks in adults or by 3 to 4 weeks in adolescents, patients should be referred to an appropriate clinician for focused treatment. Cervical injury or aerobic exercise intolerance can be treated as soon as possible because there is evidence that early intervention improves patient outcome. , ,
Ocular Rehabilitation
After identifying the presence and degree of impairments using physical examination techniques, specific treatments can be prescribed.
Repetitive saccades
Patients are instructed to put 2 targets (eg, Post-it notes) on the wall 0.5 m apart horizontally and vertically, and stand 0.5 m away. While keeping the head still, the patient looks back and forth from each target. Patients start with the number of repetitions that cause symptoms plus 3 more to increase task stamina, then take a brief rest and repeat until they have completed 1 minute of horizontal and vertical saccades each. Patients should advance by 3 to 5 repetitions every 1 to 2 days with an eventual goal of 120 Hz (1 Hz for moving from 1 target to the other) without symptom provocation.
Static fixation
For static fixation, patients are instructed to put 3 beads on a string (Brock string): 1 on the end, 1 in the middle, and 1 as close to the nose as possible but still in focus. Patients start by looking at the farthest, then the middle, then the closest. The goal is to keep each bead in focus (clear, not blurry or double). As patients improve with training, they move the closest bead closer to the nose while keeping it clear, with the goal of getting it within 6 cm from the nose without diplopia.
Dynamic fixation
Patients are instructed to perform pencil pushups. The patient holds a pen at an arm’s length away and focuses on a single point (eg, some letters on the pen) while slowly (∼1 cm/s) bringing the pen closer to the nose and attempting to keep it in focus. When the letters blur, the patient continues until the letters become double. The patient then brings the pen back out until it becomes single and clear again. This process is repeated for at least 1 minute until the patient is able to keep the letters in focus within 6 cm from the nose.
To increase compliance with these exercises, there are multiple smartphone/tablet app-based protocols, approved and recommended by the College of Optometrists in Vision Development, that are easily accessible with built-in parameters for progression.
Vestibular Rehabilitation
In an RCT by Schneider and colleagues, 25 patients with SRC were randomized to either manual cervical therapy (placebo) or manual cervical therapy plus vestibular rehabilitation. Patients in the manual cervical therapy plus vestibular rehabilitation group were medically cleared to return to sports at a much faster rate. Vestibular rehabilitation includes techniques to improve gaze stabilization and dynamic balance. Some patients have a concomitant BPPV after SRC and may require a physical therapist to supervise habituation and desensitization exercises and/or perform canalith repositioning maneuvers.
Gaze stabilization
Patients are instructed to practice focusing on a target while turning the head from side to side, then up and down. The goal is to improve until the head moves 20° to 30° in each direction at greater than or equal to 2 Hz with precise focus (no blurriness) or symptom provocation. Often this means starting at a lower level and making accommodations to achieve perfect visual focus without symptoms; for example, using a larger target in a visually simplistic environment and moving the head at a slower speed (using a metronome helps) until the desired level is achieved.
For patients with visual motion sensitivity or visual vertigo, habituation exercises to desensitize provocative visual environments are beneficial for symptom management and clear vision. To improve balance, minimizing visual input and maximizing vestibular and somatosensory input with challenges to posture, center of gravity, base of support, and head/body movement has been shown to be effective.
Cervical Rehabilitation
The management of cervical strain with cervicalgia is designed to reduce local inflammation and muscle spasm, restore range of motion, and recalibrate communication between the cervical spine and the vestibular and oculomotor systems. This outcome requires a tailored cervical spine rehabilitation program that includes manual therapy, passive and active range-of-motion exercises, low-velocity mobilizations, proprioceptive retraining, and exercises to strengthen the deep and superficial cervical musculature. Empirical support for this approach is provided by the Schneider and colleagues RCT (described earlier), as well as observational studies showing improved pain and sensorimotor outcomes in patients with whiplash treated with multimodal cervical spine rehabilitation. , In addition, a recent review concluded that rehabilitation programs that use cervical spine mobilization and manipulation techniques are effective for cervicogenic headaches. Patients with severe whiplash-type injuries who develop persistent cervicogenic headaches and occipital neuralgia may benefit from pharmacologic management and occipital nerve injections. Patients with predominantly cervical symptoms typically do not experience an early symptom-limited threshold on graded aerobic exercise testing and so may exercise to prevent aerobic deconditioning.
Sub–symptom Exercise Rehabilitation
The treatment of SRC has changed significantly in recent years. Emerging research has shown that mild symptom exacerbation does not damage the brain and may be essential to helping patients recover from SRC. Prolonged rest causes physical deconditioning and impairs CBF regulation, whereas regular exercise is known to improve CBF control. Exercise is known to reduce concussion symptom burden, and improves sleep problems and mental alertness. Several recent RCTs and well-designed experimental trials have shown the benefit of this approach. , Although subsymptomatic aerobic exercise seems to be beneficial for SRC, it is crucial to remember that patients should be cautioned that intense exercise performed soon after injury can increase symptoms and prolong recovery. , In an animal study by Griesbach and colleagues, rats with simulated concussion who were allowed to exercise voluntarily showed improved cognitive performance versus those that were forced to exercise. This study highlights the importance of a correct dose of exercise for SRC rehabilitation.
If exercise tolerance testing is available, an individualized sub–symptom threshold aerobic exercise prescription can be provided. Patients are instructed to exercise to symptom exacerbation or for at least 20 minutes a day at 80% to 90% of the heart rate achieved on the treadmill test. Patients typically return every 1 to 2 weeks to reassess exercise tolerance for a new heart rate prescription. If exercise tolerance testing is not available, a more conservative approach for prescribing exercise should be used to not exacerbate symptoms beyond rehabilitative value. Patients with SRC can calculate their age-appropriate maximum heart rates using the Karvonen equation (maximum heart rate = 220 − age in years) and begin exercising at 50% of their maximum using a heart rate monitor. If the patient is able to tolerate this intensity of exercise without worsening of symptoms, it may be possible to increase the target heart rate by 5 to 10 beats/min the subsequent day. If the patient begins to feel symptomatic, the patient should perform aerobic exercise below that level until able to tolerate higher intensities. It is of paramount importance to caution patients to avoid sustained exercise above the symptom-exacerbation threshold because it may be detrimental to recovery. ,
Aerobic exercise is recommended rather than resistance exercise (eg, rowing and weight lifting) because the concussed brain is intolerant of wide swings in blood pressure. The authors recommend stationary biking to begin with because it is safe and minimizes head motion. If tolerated, the patient may advance to brisk walking or jogging, either on a treadmill or outside. Swimming is an ideal form of aerobic exercise but patients may find it hard to actively monitor heart rate, although water jogging with a flotation device would be acceptable. Similarly, outdoor biking is not recommended to begin with because patients with vestibular dysfunction may be at risk for high-velocity falls. Delayed recovery from SRC despite return of good exercise tolerance should prompt the clinician to evaluate other potential symptom generators (eg, oculomotor, vestibular, cervical, mood, or migraine disorders). In cases where the lowest exercise prescription elicits significant symptom exacerbation, physical rest is indicated. Patients are encouraged to keep a daily diary of exercise performance and symptoms.
Clearance and return to play
The 2016 Berlin CISG guideline defines clinical functional recovery as a return to normal activities, including school, work, and sport. Operationally, this means that the athlete has returned to a baseline level of symptoms with a normal neurologic examination, including an assessment of oculomotor function and balance. Athletes should have returned to normal school participation before returning to sport. As outlined in the Berlin CISG guideline, the recovery process and return to sport participation after SRC follows a graduated stepwise rehabilitation strategy of increasing tolerance to exercise and sport skills. The athlete proceeds to the next level when criteria (activity, heart rate, duration of exercise) are met without recurrence of concussion-related symptoms. In general, in younger and nonprofessional athletes, each step requires 24 hours so that it takes approximately 1 week to proceed through the full return-to-play (RTP) protocol once they have been declared recovered at rest. However, in professional athletes, the rate of progression through the RTP protocol may be modified to the individual to speed recovery and may progress through more than 1 stage a day. The time frame for RTP varies with player age, concussion history, and level of sport.
Disclosure
The authors have nothing to disclose.
References
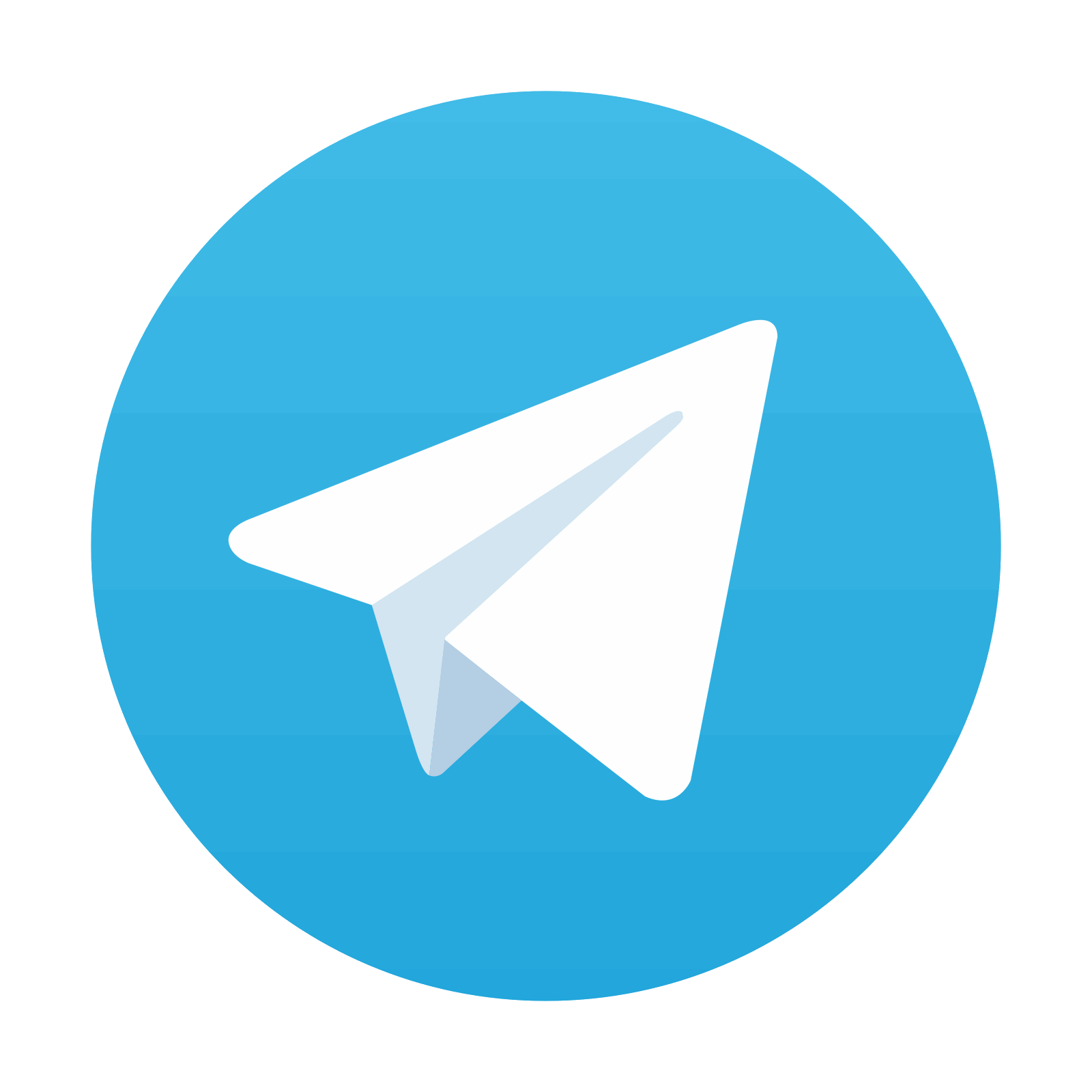
Stay updated, free articles. Join our Telegram channel

Full access? Get Clinical Tree
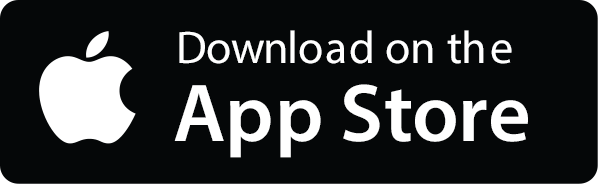
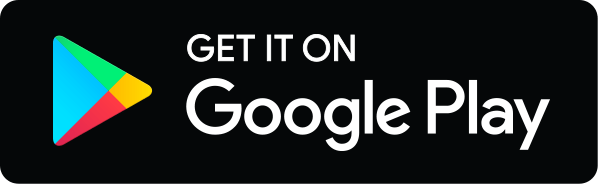
