Reestablishing Neuromuscular Control
Scott Lephart, PhD, ATC
C. Buz Swanik, PhD, ATC
Troy Blackburn, PhD, ATC
After reading this chapter,
the athletic training student should be able to:
- Explain why neuromuscular control is essential in the rehabilitation process.
- Define proprioception, kinesthesia, neuromuscular control, and stiffness.
- Explain the physiology of articular and tenomuscular mechanoreceptors.
- Describe the afferent and efferent neural pathways.
- Recognize the importance of feedforward and feedback neuromuscular control.
- Identify the various techniques for reestablishing neuromuscular control in both the upper and lower extremities.
WHAT IS NEUROMUSCULAR CONTROL?
Neuromuscular control refers to the efferent (motor) response to sensory information.81 Several sources of sensory information are essential for producing adequate muscle activity and dynamic joint stability, including proprioception, kinesthesia, and force sense. Proprioception refers to conscious and unconscious appreciation of joint position, whereas kinesthesia is the sensation of joint motion or acceleration.120 The perception of force (force sense) is the ability to estimate joint and musculotendinous loads.82 These signals are transmitted to the spinal cord via afferent (sensory) pathways. Conscious awareness of joint motion, position, and force is essential for motor learning and the anticipation of movements, while unconscious proprioception modulates muscle function and initiates reflexive joint stabilization. As such, neuromuscular control encompasses motor output that is responsible for producing movement and providing dynamic joint stability and postural stability.
Two motor control mechanisms (feedforward and feedback) are involved with interpreting afferent information and coordinating efferent responses.41,82 Feedforward neuromuscular control involves planning movements based on “real-time” sensory information that is integrated with learned somatosensory patterns from past experiences,41,98 while feedback processes continuously regulate muscle activity through reflex pathways. Feedforward mechanisms are responsible for preparatory muscle activity; feedback processes are associated with reactive/reflexive muscle activity. Because of skeletal muscle’s orientation and activation characteristics, a diverse array of movement capabilities can be coordinated involving concentric, eccentric, and isometric activation, while excessive joint motion is restricted. Therefore, dynamic restraint is achieved through preparatory and reflexive neuromuscular control.40,41,58,62,73
Muscle activity enhances dynamic joint stability by increasing joint congruency, providing eccentric absorption of external forces applied to the body, and increasing muscle stiffness. Many joints (eg, glenohumeral and tibiofemoral) possess limited bony congruency and are, therefore, reliant on muscle activation to limit loading of passive capsuloligamentous structures. An enhancement in joint stability can be achieved via muscle activity by increasing compressive force across the joint and increasing joint contact area such as occurs when the rotator cuff pulls the humeral head into the glenoid fossa. Muscle activity also limits loading of passive tissues by providing eccentric absorption of force applied to the body (ie, “shock absorption”). For example, Norcross et al122 demonstrated that lower extremity energy absorption characteristics influence biomechanical factors associated with anterior cruciate ligament (ACL) loading and injury.
The level of muscle activation, whether it is preparatory or reactive, greatly modifies the muscle’s stiffness properties.75,121,129 From a mechanical perspective, muscle stiffness refers to the ratio of the change of force to the change in length.5,40,42 In essence, muscles that are stiffer resist lengthening more effectively and provide more effective dynamic restraint to joint perturbation.5,113 Therefore, muscle stiffness generated by feedforward neuromuscular activity prior to joint loading is one of the most important mechanisms for dynamic restraint of joints.75,135,142,145 However, high levels of muscle stiffness would restrict the fast joint motions necessary for physical activity, so muscle stiffness regulation occurs continuously to optimize both joint stability to motion.155,156 Clinical studies have recently established the importance of muscle stiffness in the dynamic restraint system.59,67,75,144 In the knee, for example, increasing hamstring muscle activation also increases hamstring stiffness, and there is a moderate correlation between the degree of muscle stiffness in ACL-deficient patients and their functional ability.108,138 Additionally, individuals with greater hamstring stiffness display less anterior tibial translation in response to joint perturbation and lesser frontal and sagittal plane knee loading during landing.16,18 Therefore, efficient regulation of muscle stiffness might be vital for restoring functional stability.
Figure 6-1. Functional stability paradigm depicting the influence of mechanical instability and proprioceptive deficits on neuromuscular control and functional stability, which predisposes the knee to repetitive injury.
WHY IS NEUROMUSCULAR CONTROL CRITICAL TO THE REHABILITATION PROCESS?
Reestablishing neuromuscular control is a critical component in the rehabilitation of pathological joints. The objective of neuromuscular control activities is to refocus the patient’s awareness of peripheral sensations and process these signals into more coordinated motor strategies. This muscle activity serves to protect joint structures from excessive strain and provides a prophylactic mechanism to recurrent injury. Neuromuscular control activities are intended to complement traditional rehabilitation protocols, which encompass the modulation of pain and inflammation, restoration of flexibility, strength, and endurance, as well as psychological considerations.
Peripheral mechanoreceptors within articular and tenomuscular structures mediate neuromuscular control by conveying joint motion and position sense to the central nervous system (CNS). The primary roles of articular structures such as the capsule, ligaments, menisci, and labrum are to stabilize and guide skeletal segments while providing mechanical restraint to abnormal joint movements.1 However, capsuloligamentous tissue also has a sensory role essential for detecting joint motion and position.51,83,133
Injury to articular structures results not only in a mechanical disturbance that manifests a joint laxity, but also in a loss of joint sensation. Microscopic nerves arising from peripheral mechanoreceptors within articular structures may also be damaged, which is referred to as deafferentation.80,129,137 This partial deafferentation disrupts sensory feedback necessary for effective neuromuscular control and joint stabilization. There is substantial evidence suggesting that the aberrations in muscle activity subsequent to joint injury are a result of disrupted neural pathways.12,20,80,107,126,151,157 Therefore, joint pathology reduces mechanical stability and often diminishes the capability of the dynamic restraint system, rendering the joint functionally unstable (Figure 6-1).
The concept of mechanical vs functional stability can be illustrated by comparisons of ACL-deficient and ACL-reconstructed patients. Some ACL-deficient individuals, labeled “copers” by Chmielewski et al28,29 are capable of high levels of function and dynamic joint stability in the presence of mechanical instability attributable to the absence of the ACL. These individuals develop enhanced dynamic restraint capabilities via the rehabilitation process, potentially by enhancing hamstring stiffness.114 Conversely, ACL reconstruction results in mechanical stability of the knee joint as evidenced by reduced anterior knee laxity,2 but many ACL-reconstructed patients experience sensations of the joint “giving way,” which is indicative of functional instability.117
The goal of reconstructive surgery is to restore mechanical stability, but evidence supports the potential for reinnervation of graft tissue by peripheral receptors.123 Therefore, surgery, combined with rehabilitation, promotes several neuromuscular characteristics associated with the dynamic restraint system.102,103 Clinical research has revealed a number of activities that enhance these characteristics and are beneficial to developing neuromuscular control.27,70,146 To accomplish this, clinicians must identify the peripheral and central neuromuscular characteristics that compensate for mechanical insufficiencies and encourage these adaptations to restore functional stability.
Rehabilitation of the pathological joint should address the preparatory (feedforward) and reactive (feedback) neuromuscular control mechanisms required for joint stability. The 4 elements crucial for reestablishing neuromuscular control and functional stability are joint sensation (position, motion, and force), dynamic stability, preparatory and reactive muscle characteristics, and conscious and unconscious functional motor patterns.101,142 The following sections will define the sensory receptors and neural pathways that contribute to joint stabilization. The theoretical framework for reestablishing neuromuscular control will be presented, followed by specific activities designed to encourage the peripheral, spinal, and cortical adaptations crucial for improving functional stability.
THE PHYSIOLOGY OF MECHANORECEPTORS
Articular Mechanoreceptors
The dynamic restraint system is informed by specialized nerve endings called mechanoreceptors.61 A mechanoreceptor functions by transducing mechanical deformation of tissue (eg, stretching, compression) into frequency-modulated neural signals.61 Increased tissue deformation is coded by an increased afferent discharge rate (action potentials/second) or a rise in the quantity of mechanoreceptors stimulated.61,64 For example, increasing pressure placed on the skin increases the number of cutaneous receptors that are activated, as well as their activation frequencies. These signals provide sensory information concerning internal and external forces acting on the joint. Three morphological types of mechanoreceptors have been identified in joints: Pacinian corpuscles, Meissner corpuscles, and free nerve endings.51,61,85 These mechanoreceptors are classified as either quick adapting because they cease discharging shortly after the onset of a stimulus, or slow adapting because they continue to discharge as long as the stimulus is present.31,51,61,83,132 In healthy joints, quick-adapting mechanoreceptors are believed to provide conscious and unconscious kinesthetic sensations in response to joint movement or acceleration, while slow-adapting mechanoreceptors provide continuous feedback and, thus, proprioceptive information relative to joint position.29,49,57,130
Tenomuscular Mechanoreceptors
Any change in joint position simultaneously alters muscle length and tension. Muscle spindles, embedded within skeletal muscle, detect muscle length, changes in muscle length, and the rate of muscle lengthening, transmitting these signals to the CNS through the fastest afferent nerves.6,30,64 This sensory information from the muscle spindle regarding changes in muscle length and the rate of change in muscle length (Type Ia afferent neurons) contributes to the sensation of kinesthesia, while input regarding muscle length (Type II afferent neurons) contributes to proprioception. Muscle spindles are also innervated by small motor fibers called gamma efferents.6,64,95 Activity of these motor fibers permits the muscle spindle to become more sensitive, if necessary, and accommodates for changes in muscle length while continuously transmitting afferent signals.6,64,79,81 Muscle spindle afferents project directly on skeletal motoneurons through monosynaptic reflexes.159 When muscle spindles are stimulated, they elicit a reflex contraction in the agonist muscle (eg, the spinal stretch or “knee jerk” reflex).79,116,159
Golgi tendon organs (GTOs) are also capable of regulating muscle activity and are responsible for monitoring muscle tension or load.44,74 Located within the tendon and tenomuscular junction, GTOs are force detectors and, thus, are able to protect the tenomuscular unit by reflexively inhibiting muscle activation when high tension might cause damage. During physical activity, moderate levels of muscle tension may actually reverse this reflex, thus making muscle tension a stimulus to muscle recruitment. Generally, with high muscle tension, GTOs would have the opposite effect of muscle spindles by producing a reflex inhibition (relaxation) in the muscle being loaded.61,74
Cutaneous Receptors
Pressure and stretch receptors located in the skin are thought to contribute to proprioception, kinesthesia, and force sense. Their contribution to force sense is intuitive, as compression of the skin, such as occurs in the fingers and hand while grasping an object, provides an indication of the force being exerted on the object. Similarly, joint motion causes stretching and compression of skin on a joint, thus stimulating cutaneous receptors and contributing to sensations of joint position and motion. These notions are supported by research demonstrating improvements in proprioception and neuromuscular control with the use of compression devices (eg, bandages and neoprene sleeves) and athletic tape.32,33,77,134
NEURAL PATHWAYS OF PERIPHERAL AFFERENTS
Understanding the extent to which articular, tenomuscular, and cutaneous sensory information is used requires analysis of the reflexive and cortical pathways employed by peripheral afferents. Encoded signals concerning joint motion, position, and force are transmitted from peripheral receptors, via afferent pathways, to the spinal cord.43,51 Within the spinal cord, interneurons link ascending pathways (tracts) to the cerebral cortex to permit conscious appreciation of proprioception, kinesthesia, and force. Two reflexive pathways couple articular receptors with motor nerves and tenomuscular receptors in the spinal column. A third monosynaptic reflex pathway links the muscle spindles directly with motor nerves.
Sensory information from the periphery is used by the cerebral cortex for somatosensory awareness and feedforward neuromuscular control, whereas balance and postural control are processed at the brainstem.31,53,64,82 Balance is influenced by the same peripheral afferent mechanisms that mediate joint proprioception and is partially dependent upon the inherent ability to integrate somatosensory input (joint position sense and kinesthesia) with vision and the vestibular apparatus. Any disassociation between these 3 sensory modalities can lead to exaggerated postural sway. Balance, therefore, is frequently used to measure sensorimotor integration and functional joint stability because deficits can result from aberrations in the afferent feedback loop of the lower extremity.
Synapses in the spinal cord link afferent fibers from articular and tenomuscular receptors with efferent motor nerves, constituting the reflex loops between sensory information and motor responses. This reflexive neuromotor link contributes to dynamic stability by using the feedback process for reactive muscular activation.20,126,139 Interneurons within the spinal column also connect articular receptors and GTOs with large motor nerves innervating muscles and small gamma motor nerves innervating muscle spindles. Johansson et al78 contends that articular afferent pathways do not exert as much influence directly on skeletal motoneurons as previously reported, but rather they have more frequent and potent effects on muscle spindles. Muscle spindles, in turn, regulate muscle activation through the monosynaptic stretch reflex. Articular afferents therefore have some influence on the large skeletal motor nerves as well as the spindle receptors, via gamma motor nerves.78,80
This sophisticated articular-tenomuscular link has been described as the “final common input.”3,80 The final common input suggests that muscle spindles integrate peripheral afferent information and transmit a final modified signal to the CNS.3,80 This feedback loop is responsible for continuously modifying muscle activity during locomotion via the muscle spindle’s stretch reflex arc.72,121 By coordinating reflexive and descending motor commands, muscle stiffness is modified and dynamic stability is maintained.80,90
Several reflexes derived from peripheral mechanoreceptors contribute to dynamic joint stability. Increases in muscle length such as those occurring with joint perturbation (eg, the peroneals during rapid ankle inversion) excite muscle spindle afferents. The resulting afferent volleys result in spinal (short latency), medium-latency, and long-latency stretch reflex responses. These reflex responses, in turn, provide heightened resistance to further muscle lengthening, thus resisting joint perturbation.13
Similarly, mechanoreceptors in ligament have been demonstrated to elicit reflexive responses in the musculature antagonistic to the imposed loading. For example, tensile loading of the ACL elicits a reflexive response in the hamstrings designed to limit ligament loading (ie, the ligament stress-elicited reflex).52,130
FEEDFORWARD AND FEEDBACK NEUROMUSCULAR CONTROL
The efferent response of muscles transforming neural information into physical energy is termed neuromuscular control.81 Traditional beliefs about the processing of afferent signals into efferent responses for dynamic stabilization were based on reactive or feedback neuromuscular control pathways.99 More contemporary theories emphasize the significance of preactivated muscle tension in anticipation of movements and joint loads. Preactivation suggests that prior sensory feedback (experience) concerning the task is used to preprogram muscle activation patterns. For example, visual input is combined with “sensory memories” from previous experiences to increase preparatory activity of lower extremity musculature with increasing landing height in anticipation of greater impact forces.49,54 This process is described as feedforward neuromuscular control.42,49,60,96
Feedforward neuromuscular control uses advance information about a task, usually from experience, to determine the most coordinated strategy for executing the impending functional task.37,91 These centrally generated motor commands are responsible for preparatory muscle activity and high-velocity movements.82 For example, de Britto et al39 demonstrated that increasing the height of drop-landing tasks resulted in an increase in preparatory quadriceps activity (ie, before ground contact) in an effort to accommodate greater impact forces. Preparatory muscle activity serves several functions that contribute to the dynamic restraint system. By increasing muscle activation levels, the stiffness of the tenomuscular unit is increased.118 This increased muscle activation and stiffness can improve the stretch sensitivity of the muscle spindle system while reducing the electromechanical delay (EMD) required to develop muscle tension.32,40,62,73,80,113,118,129 EMD refers to the period that elapses between the arrival of a neural impulse (electrical) initiating muscle contraction and the development of force (mechanical). Clinical research has also shown that the stretch reflex can increase muscle stiffness 1 to 3 times.65,108 Heightened stretch sensitivity and stiffness could improve the reactive capabilities of muscle by providing additional sensory feedback and superimposing stretch reflexes onto descending motor commands.40,79,115 Whether muscle stiffness increases stretch sensitivity or decreases EMD (or both), it appears to be crucial for dynamic restraint and functional stability (Figure 6-2). Preactivated muscles provide quick compensation for external loads and are critical for dynamic joint stability.40,62 Sensory information about the performance is then used to evaluate the results based on how the brain expected the task to feel, and helps arrange future muscle coordination strategies.
The feedback mechanism of motor control is characterized by numerous reflex pathways continuously adjusting ongoing muscle activity.21,41,99,116 Information from joint and muscle receptors can reflexively initiate and coordinate muscle activity for motor tasks. This feedback process, however, can result in long conduction delays and is best equipped for maintaining posture and regulating slow stereotyped movements such as walking.82 For example, Konradsen et al91 demonstrated that the combination of conduction delays and EMD prolonged the reflexive response of the peroneal musculature to sudden ankle inversion such that it was incapable of preventing capsuloligamentous loading. The efficacy of reflex-mediated dynamic stabilization is therefore related to the speed and magnitude of joint perturbations. It is unclear what relative contribution feedback-mediated reflexes provide when in vivo loads are placed on joints.
Figure 6-2. Diagram depicting the influence of muscle stiffness on EMD and muscle spindle sensitivity, which enhances the reactive characteristics of muscle for dynamic joint restraint.
Partial differentiation in combination with inflammation, joint effusion, joint laxity, and pain often leads to voluntary muscle activation failure following capsuloligamentous injury, particularly in the early stages of rehabilitation.131 This phenomenon is known as arthrogenic muscle inhibition (AMI), and is particularly common following knee pathology.68 AMI can also be present bilaterally following unilateral injury.14,149 Various rehabilitation modalities such as electrical stimulation, ultrasound, and vibration may represent effective approaches for minimizing AMI.19,140
Both feedforward and feedback neuromuscular control can enhance dynamic stability if the sensory and motor pathways are frequently stimulated. Each time a signal passes through a sequence of synapses, the synapses become more capable of transmitting the same signal.65,71 When these pathways are “facilitated” regularly, memory of that signal is created and can be recalled to program future movements.65 Frequent facilitation therefore enhances both the memory about tasks for preprogrammed motor control and reflex pathways for reactive neuromuscular control. Therefore, rehabilitation exercise must be executed with technical precision, repetition, and controlled progression for these physiological adaptations to occur and enhance neuromuscular control.
REESTABLISHING NEUROMUSCULAR CONTROL
Patients who have sustained damage to the articular structures in the upper or lower extremities exhibit distinctive proprioceptive, kinesthetic, and neuromuscular deficits.7,8,12,20,98,103,106,110,135,136,138,158 Although identifying these abnormalities might be difficult in a clinical setting, a thorough appreciation of the pathoetiology of these conditions is necessary to guide clinicians who are attempting to reestablish neuromuscular control and functional stability. Disruption of the articular structures results in deafferentation to ligamentous and probably capsular mechanoreceptors.44,46,98,103,104,136,138 In the acute phase of healing, joint inflammation and pain can compound sensory deficits; however, this cannot account for the chronic deficits in proprioception and kinesthesia associated with pathological joints.10,85 Research has demonstrated that patients with congenital or pathological joint laxity have diminished capability for detecting joint motion and position.50,56,138 These proprioceptive and kinesthetic characteristics, coupled with mechanical instability, can lead to functional instability.99,141
Developing or reestablishing proprioception, kinesthesia, and neuromuscular control in injured patients will minimize the risk of reinjury. Capsuloligamentous retensioning and reconstruction, coupled with traditional rehabilitation, is one option that appears to restore some kinesthetic awareness, although not equal to that of noninvolved limbs.38,103,123 The objective of neuromuscular rehabilitation is to develop or reestablish afferent and efferent characteristics that enhance dynamic restraint capabilities with respect to in vivo loads. Four basic elements are crucial to reestablishing neuromuscular control and functional stability:
- Proprioceptive and kinesthetic sensation
- Dynamic joint stabilization
- Reactive neuromuscular control
- Functional motor patterns99
In the pathological joint, these dynamic mechanisms may be compromised due to deafferentation and can result in a functionally stable joint. Several afferent and efferent characteristics contribute to the efficient regulation of these elements and the maintenance of neuromuscular control. These characteristics include the sensitivity of peripheral receptors and facilitation of afferent pathways, muscle stiffness, the onset rate and magnitude of muscle activity, agonist/antagonist coactivation, reflex muscle activation, and discriminatory muscle activation. Specific rehabilitation techniques allow these characteristics to be modified, significantly impacting dynamic stability and function.12,27,70,76,98,146
Although more prospective clinical research is needed to establish the “best practice” approach in support of the evidence-based medical model, several exercise techniques show promise for inducing beneficial adaptations to these characteristics. The plasticity of the neuromuscular system to change is what permits rapid modifications during rehabilitation that ultimately enhance preparatory and reactive muscle activity.12,71,76,142–144 The techniques include open and closed kinetic chain activities, balance training, eccentric and high-repetition/low-load exercises, reflex facilitation through reactive or “perturbation” training, stretch-shortening activities, and biofeedback training. Traditional rehabilitation, accompanied by these specific techniques, results in beneficial adaptations to the neuromuscular characteristics responsible for dynamic restraint, enhancing their efficiency for providing a functionally stable joint.
To restore dynamic muscle activation necessary for functional stability, one must employ simulated positions of vulnerability that necessitate reactive muscle stabilization. Although there are inherent risks in placing the joint in positions of vulnerability, if this is done in a controlled and progressive fashion, neuromuscular adaptations will occur and subsequently permit the patient to return to competitive situations with confidence that the dynamic mechanisms will protect the joint from subluxation and reinjury.
Clinical Decision-Making Exercise 6-1
Following a grade 2 ankle sprain and a rehabilitation program to regain strength in the lateral lower leg muscles, your soccer patient continues to sustain repeated inversion ankle injuries during cutting maneuvers. What components of neuromuscular control might be deficient in this patient? What type of rehabilitation exercises should you implement to enhance neuromuscular control?
Neuromuscular Characteristics
Peripheral Afferent Receptors
The foundation for feedback and feedforward neuromuscular control is based on reliable motion, position, and force information. Altered peripheral afferent information can disrupt motor control and functional stability. Closed kinetic chain exercises create axial loads that maximally stimulate articular receptors, especially near the end range of motion (ROM), while tenomuscular receptors are excited by changes in length and tension.30,61,80,153,154,161 Open chain activities may require more conscious awareness of limb position because of the non-constrained and freely moving distal segment. Performing open or closed chain exercises under weighted conditions increases the level of difficulty and coactivation, which may be used as a training stimulus.97 Chronic athletic participation can also enhance proprioceptive and kinesthetic acuity by repeatedly facilitating afferent pathways from peripheral receptors. Highly conditioned patients demonstrate greater appreciation of joint kinesthesia and more accurately reproduce limb position than sedentary controls do.9,104,108 Whether this is a congenital endowment or a training adaptation, greater awareness of joint motion and position can improve feedforward and feedback neuromuscular control.104
Muscle Stiffness
Muscle stiffness has a significant role in preparatory and reactive dynamic restraint by resisting and absorbing joint loads.75,111,113,114,145 Therefore, exercise modes that optimize muscle stiffness should be encouraged during rehabilitation. Research by Bulbulian and Bowles23 and Pousson et al128 has established that eccentric loading increases muscle tone and stiffness, and several authors have demonstrated increases in muscle stiffness with isometric loading.17,24,93,94 The GTO receptor is normally associated with muscle inhibition and, thus, protects the tenomuscular unit from excessive strain. However, chronic overloading of the musculotendinous unit may result in connective tissue proliferation around GTOs that, in effect, desensitizes this mechanoreceptor to muscle tension. If this inhibitor effect can be decreased, reactive muscle stiffening may be facilitated through increased muscle spindle activity.74 It is also known that during functional activities, GTO inhibition reverses and may actually enhance muscle recruitment.82 Such evolutions impact both the neuromuscular and tendinous components of stiffness.23,58,118,128
Training techniques that emphasize low loads and high repetitions cause connective tissue adaptations similar to those found with eccentric training. However, increased muscle stiffness resulting from this rehabilitation technique can be attributed to fiber type transition.58,74,92,95 Slow-twitch fibers have longer cross-bridge cycle times and can maintain the prolonged, low-intensity contractions necessary for postural control.95 In the animal model, Goubel and Marini58 found that low-load/high-repetition training resulted in higher muscle stiffness compared to strength training. However, Kyröläinen and Komi’s95 analysis of power- and endurance-trained patients inferred that muscle stiffness was greater in the power-trained individuals because the onset of muscle preactivation (electromyography) was faster and higher prior to joint loading. It appears that endurance training might enhance stiffness by increasing the baseline motor tone and cross-bridge formation time, whereas power training alters the rate and magnitude of muscle tension during preactivation. Both of these adaptations readily adhere to existing principles of progressive rehabilitation, where early strengthening exercises focus on low loads with high repetitions, progressing to shorter, more explosive, sport-specific activities. Research assessing the efficacy of low-load/high-repetition training vs high-load/low-repetition training would be beneficial for optimizing muscle stiffness and functional progression in the injured patient.
Reflex Muscle Activation
Various training modes also cause neuromuscular adaptations that might account for discrepancies in the reflex latency times between power- and endurance-trained patients. Sprint- and/or power-trained individuals have more vigorous reflex responses (tendon-tap) relative to sedentary and endurance-trained samples.88,89,150 McComas112 suggests that strength training increases descending (cortical) drive to the large motor nerves of skeletal muscle and the small efferent fibers to muscle spindles, referred to as alpha-gamma coactivation. Increasing both muscle tension and efferent drive to muscle spindles results in a heightened sensitivity to stretch, consequently reducing reflex latencies.74 Melvill-Jones115 suggests that the stretch reflexes are superimposed on preprogrammed muscle activity from higher centers, illustrating the concomitant use of feedforward and feedback neuromuscular control for regulating preparatory and reactive muscle stiffness. Therefore, preparatory and reactive muscle activation might improve dynamic stability and function if muscle stiffness is enhanced in a mechanically insufficient or reconstructed joint.
A limited number of clinical training studies have been directed at improving reaction times.12,76,157 Ihara and Nakayama76 significantly reduced the latency of muscle reactions during a 3-week period by inducing perturbations to patients on unstable platforms. Several other researchers later confirmed this finding with rehabilitation programs designed to improve reflex muscle activation.12,157 Beard et al12 and Wojtys et al157 suggest that agility-type training in the lower extremity produces more desirable muscle reaction times compared to strength training. This research has significant implications for reestablishing the reactive capability of the dynamic restraint system. Reducing EMD between protective muscle activation and joint loading can increase dynamic stability and function. Fitzgerald et al47,48 describe a perturbation training program that is dependent on a sense of force feedback. Patients are exposed to rotatory and translatory movement that progress from predictable perturbations to random, and from small/slow movements to those that are large/fast. Key instruction for the success of the exercises is to match the perturbation but not under- or overreact. This is critical to the concept of optimal stiffness regulation. Overstiffening a muscle/joint complex may provide stability but is not functional, while understiffening may permit episodes of “giving way” or “buckling.”
Figure 6-3. Biofeedback training reestablishes discriminative muscle control, eliminating muscle imbalance and promoting functionally specific muscle activation patterns.
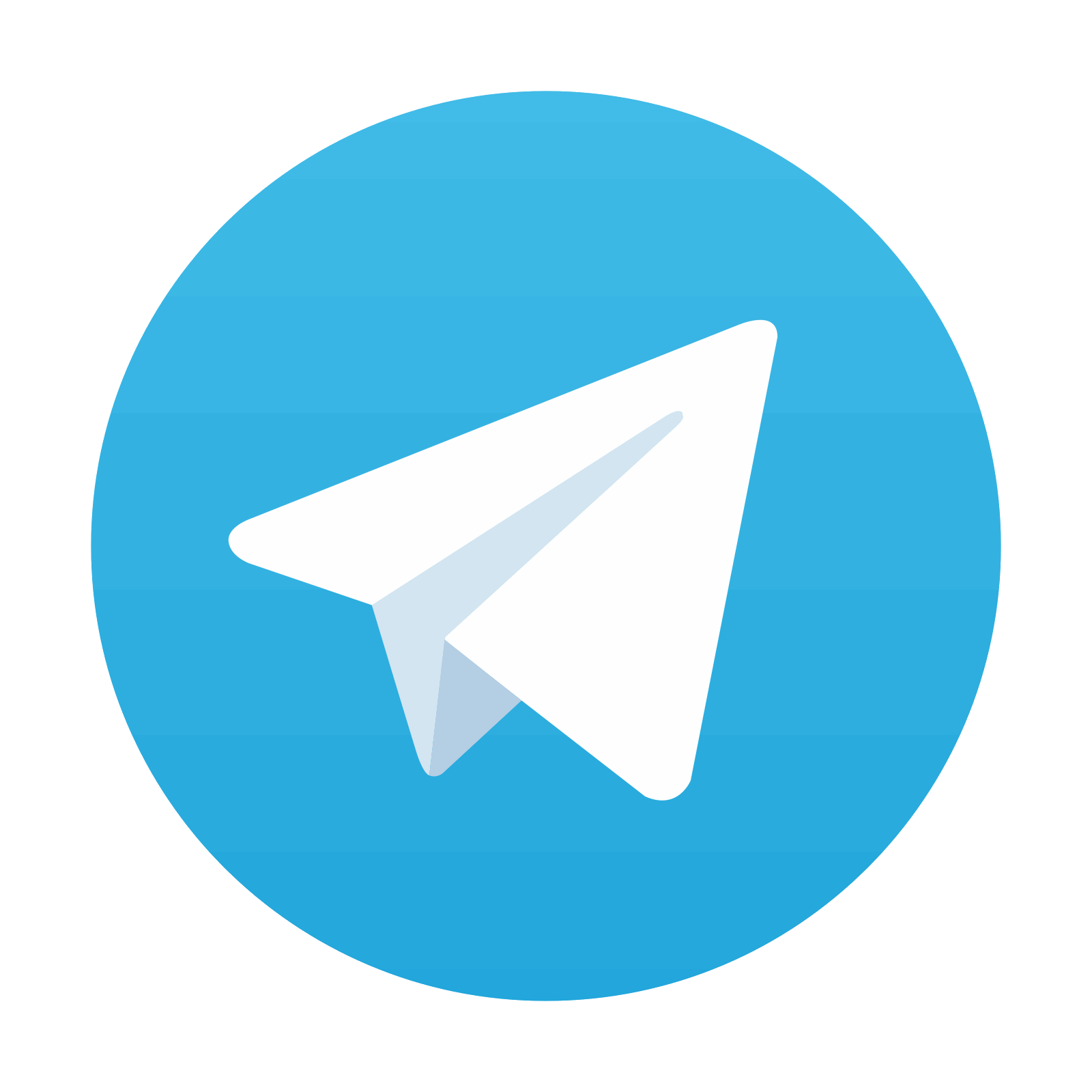
Stay updated, free articles. Join our Telegram channel

Full access? Get Clinical Tree
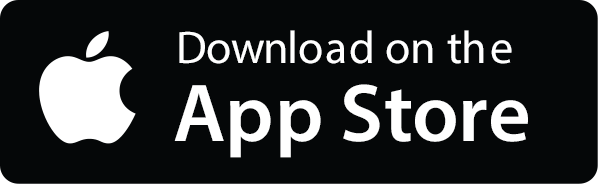
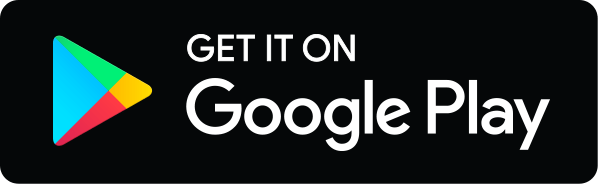