9 Rationale and Biomechanics of the Reversed Shoulder Prosthesis: The French Experience Treatment of the cuff-deficient shoulder has been a vexing problem for many years. Although the rotator cuff provides control of overhead rotation of the humerus and internal and external rotation (ER) of the arm, its main function is to stabilize the humeral head in the confines of the glenoid, thereby creating a stable fulcrum around which the deltoid can act, providing forward flexion of the humerus. When the rotator cuff is torn, the dynamic balance of the shoulder can be lost; however, not every advanced cuff tear (CT) leads to loss of forward flexion beyond 90 degrees (i.e., pseudoparalysis). In fact, some patients can present with complete tears of the posterior cuff, but maintain forward flexion if there is a balance between internal and external rotatory forces. An intact coracoacromial arch can provide a stable articulation allowing the deltoid to work when there is a balance between the subscapularis and teres minor. If the dynamic balance of the joint is lost and arthropathy ensues, what options are available? Constrained arthroplasty seemed to provide great promise for restoration of function because the humerus could be stabilized, allowing the deltoid to work. Completely constrained ball and socket designs, introduced in the 1970s, were adapted from hip prostheses (Bickel, Macnab-English, Stanmore, Michael-Reese, Post).1,8 Subsequently, several prostheses were introduced based on a reverse ball-and-socket design (Fenlin, Gerard, Kessel, Kölbel, Liverpool, Neer, and Avery ll).2,3 To achieve appropriate resting length and tension on the deltoid, the original reverse ball and socket designs lateralized the humeral component; concomitantly, the center of rotation was lateralized. In fact, the instant center of rotation was lateralized outside of the scapula, creating a lever arm between the center of rotation and the bone/implant interface. Because of the vectors involved with humeral movement below 90 degrees (i.e., the initiation of abduction or humeral flexion), both torque and sheer between the implant and the bone were created, resulting in loosening (Fig. 9–1). Moreover, the constraint between the humeral and the glenoid components meant that all of the forces were transmitted to the glenoscapular interface. The failures and poor results led to abandonment of both reverse architecture and constrained designs. Experience with unconstrained total shoulder arthroplasty for the cuff-deficient shoulder was also unsatisfying as the “rocking-horse” effect led to early failure, and hemiarthroplasty became the recommended treatment.3,4 Bipolar arthroplasty gained a brief following, and has remained the procedure of choice for a select few.5,6–19 Figure 9–1 Previous reverse ball and socket prostheses tended to fail because their design resulted in excessive torque or shear forces on the glenoid component (notice the small head and neck placing the center of rotation outside the scapula). Dissatisfied with the results of standard architecture arthroplasty for the cuff-deficient shoulder, Professor Paul Grammont, a French orthopedic surgeon, designed a semi-constrained reverse prosthesis based on different, but sound, biomechanical principles. To replicate the stability of the intact cuff, a semiconstrained design was chosen to give the deltoid a stable fulcrum; to give mechanical advantage to the deltoid, he increased its resting length and recruited the posterior deltoid. Prior to Grammont, constrained shoulder prostheses tended to fail because their design resulted in excessive torque and shear forces at the glenoid component–bone interface. Furthermore, although they usually allowed some active elevation, this was, in most cases, less than 90 degrees and, primarily, scapulothoracic motion. Prosthetic instability was also a concern. Many never went beyond the experimental stage, and most are no longer commercially available. Grammont recognized that the lateral center of rotation found in previous designs created a large lever arm between the “ball” and its bone interface. By medializing the center of rotation (so that it actually lies at the glenoid bone–prosthesis interface), Grammont reduced the torque on the glenoid component. Furthermore, with initiation of abduction, the vector changed from parallel to the component/scapula interface (i.e., shear) to perpendicular to the glenoid component (i.e., compression), theoretically increasing stability of the construct when it is most vulnerable (Fig. 9–2). To power the “engine” of this new design, the deltoid, Grammont sought to maximize the resting tension on the deltoid while involving more of both the anterior and the posterior fibers. To effect the changes in the deltoid, a combined inferiorization and medialization of the humerus relative to the acromion increases deltoid tension and resting length, recruiting more anterior and posterior deltoid fibers and improving the force vectors (Fig. 9–3). The realization of these principles involved two major technological innovations: (1) on the glenoid side, use of a large ball (36 or 42 mm in diameter) with no neck; (2) on the humeral side, a small cup inclined to a non-anatomic humeral inclination of 155 degrees with conforming—but not fully constrained—articular surfaces. Both the large glenoid hemisphere and the small and conforming humeral cup optimize the range of movement, minimize impingement between the components, and improve stability. The first model of reverse prosthesis, designed by Paul Grammont in 1985, had only two components (Fig. 9–4). The glenoid component was either a metallic or a ceramic ball, initially two thirds of a sphere and 42 mm in diameter. It was designed to fit over the glenoid like a glove and was fixed with cement. The humeral component was a cemented polyethylene socket. Its concave surface was one third of a sphere, and its stem was trumpet-shaped for cementing into the humeral medullary canal. A bell saw was used to prepare the glenoid, and two broaches were used to prepare the different parts of the humerus—one for the epiphysis and one for the diaphysis. The preliminary results published (in French) in 1987 showed eight cases: three post-radiotherapy necrosis cases, one inflammatory osteoarthritis case, and four revisions of failed prostheses.9 The mean patient age was 70 years, and the cuff was absent or destroyed in all cases. Mean follow-up was only 6 months. A transacromial approach (with osteotomy of the lateral acromion) was used in all but one case. Revision osteosynthesis of an acromial nonunion was required in three cases. All shoulders were pain-free, but mobility was variable. In three cases, active anterior elevation was 100 to 130 degrees, but in the other three cases, it was less than 60 degrees. Unsatisfied with these results, Grammont made further modifications, arriving at the current design. Because he had several failures with the cemented glenoid component, he decided to change the glenoid to an uncemented system: a glenoid component fixed with a pressfit central peg supported by screws of divergent direction that counteract the initial shearing forces.2,9 He also changed the radius of the articular surface from two thirds of a sphere to half of a sphere to place the center of rotation directly in contact with the glenoid surface, decreasing lateral offset at the glenohumeral articulation, thus decreasing shearing forces. Grammont named this reverse prosthesis “Delta,” as the concept was based solely on the deltoid for both function and stability. Figure 9–2 By placing the center of rotation medial, torsion and shear at the bone–glenoid interface are reduced; furthermore, with the adduction, the force vector goes from parallel to the articular surface (shear) to perpendicular (compression). Figure 9–3 (A,B) The increased force of the deltoid is gained from both the medialization of the center of rotation, which recruits more deltoid fibers, and the lowering of the humerus, which tensions the deltoid. (A) L = increased length. The figure shows a shoulder with a reverse prosthesis and compares the center of rotation and humeral position to a native glenohumeral joint (B). There is an obvious increase in acromiohumeral distance and an overall lengthening of the humerus. Figure 9–4 The first model of the Grammont reversed prosthesis, designed in 1985, had only two components: the humeral component was all polyethylene and trumpet shaped; the glenoid component was a metallic or ceramic ball, initially ⅔ of a sphere and 42 mm in diameter. It was designed to fit over the glenoid like a glove and was affixed with cement. The second model, the Delta III reverse prosthesis (DePuy Orthopaedics, Inc., Warsaw, Indiana), became available in 1991 and is still in use today. The glenoid is uncemented, and either cemented or uncemented options are available for the humerus. The Delta III has five parts: the glenoid base plate (metaglenoid), the glenosphere, the polyethylene humeral cup, the humeral neck, and the humeral stem (Fig. 9–5). The glenoid component (metaglene) is a 29-mm disk, with a rough surface and hydroxyapatite coating. Initial fixation is ensured by a 29-mm-long central peg and four peripheral, divergent screws (3.5 or 4.5 mm in diameter). The aim is to place one screw in the base of the coracoid and one screw into the inferior scapular pillar for maximum hold. The pyramidal, divergent assembly of the screws has been designed precisely to counteract the shearing forces during initial abduction. The glenosphere is a cobalt-chrome sphere, available in two diameters: 36 and 42 mm, with a 19-mm offset. Initially, the fixation of the sphere on the metaglene was done by use of peripheral threads, but this mechanism had a tendency to unscrew, particularly in right shoulders. In 1996, glenosphere-metaglene fixation was changed to a peripheral Morse taper and reinforced by a central countersunk screw; the design change has almost completely eliminated glenosphere dissociation except in trauma cases. Figure 9–5 The Delta III reversed prosthesis has five parts: the glenoid base plate (metaglenoid), the glenosphere, the polyethylene cup, the humeral neck, and the humeral stem. A lateralized spacer, the rehausser (not pictured), can provide greater deltoid tension if the polyethylene is insufficient. Both a Delta I and a Delta II exist, but they are variations of a conventional arthroplasty that utilize the same humeral stem. The humeral stem is conical, and its surface is either polished or hydroxyapatite-coated for cemented or un-cemented fixation, respectively. It is available in three lengths: 100 mm for the standard prosthesis and 150 and 180 mm for the revision prosthesis. The humeral neck is screwed onto the humeral stem. It has a fin to control rotation, and there are holes to allow tuberosity osteosynthesis. Like the stem, it is available with a polished or a hydroxyapatite-coated surface. Three sizes are available: 36–1 and 36–2 for a 36-mm-diameter cup and 42–2 for a 42-mm-diameter cup. Initial unscrewing between the neck and the stem resulted in the placement of a polyethylene bushing between these components. The humeral cup is made of polyethylene and has two diameters conforming to the 36- and 42-mm glenospheres. It is 6 mm thick and pressfitted onto the humeral neck component. A 9-mm metallic extension may be screwed onto the neck to increase the humeral offset. The humeral cup is also available in a more constrained form with a deeper cup. The Delta I and II prostheses are “standard” unconstrained versions of the Delta III prosthesis. All three prostheses share the same stem and humeral neck. The Delta I is a hemiarthroplasty, which is easily converted from a Delta III by fixing a metal head onto the humeral neck, whereas the Delta II is a total shoulder prosthesis with a polyethylene glenoid component in place of the glenosphere. A further evolution of Grammont’s designs occurred under the direction of Dr. Gilles Walch and Prof. Pascal Boileau. The Aequalis reverse prosthesis, available since 2002, follows the Grammont design, but incorporates prosthetic changes designed to enhance both the ease of implantation and survivability: improved instrumentation, variable angle locking screws, and an array of polyethylene thicknesses. The baseplate maintains the titanium HA design, but adds the ability to vary the direction of the locking screws up to 30 degrees superiorly for the upper screw and 30 degrees inferiorly for the lower screw; furthermore, they both can be angled ±15 degrees in the anteroposterior (AP) direction. For the humeral component, a polyethylene bushing was added between the neck and the stem to minimize the risk of disassembly. The cemented stem is rough-finished cobalt chromium with scalloping to increase rotational stability; the noncemented stem is titanium with HA coating. There are four stem lengths available—100 mm, 150 mm, 180 mm, and 210 mm. There are three polyethylene sizes available: 6 mm, 9 mm, and 12 mm, plus an additional 9-mm titanium spacer. A humeral head adaptor to accommodate an Aequalis standard head is available should the prosthesis need to be converted to a hemiarthroplasty. This design confers mobility, increased deltoid torque, and stability, while minimizing the unfavorable glenoid stresses, which led to the failure of previous reverse prostheses. According to Grammont, the middle deltoid is most important for abduction. This is particularly true in a normal shoulder where parts of the anterior and posterior deltoid are at the level of, or even medial to the center of rotation;8 therefore, they either contribute very little to abduction or, in the case of the more medial fibers, may even be adductors. However, in patients with a reverse prosthesis, the medialized center of rotation may allow some of these fibers to become more effective abductors, thus augmenting the role of the anterior and posterior deltoid and further increasing the force of the deltoid overall (Fig. 9–6). To effect the necessary changes to the deltoid, it must be tensioned despite the medialization of the humerus. Unfortunately, intraoperative determination of deltoid tension is difficult and guided mostly by surgical experience; reduction should be as tight as possible, but allow for full adduction. We also have found that the conjoint tendon, exposed during a deltopectoral approach, should feel taut after reduction with the arm at the side and the elbow extended. Active elevation is restored by the fixed center of rotation, the congruent joint surfaces, and the increased deltoid torque. Deltoid torque is augmented by both the increased lever arm and the increased deltoid force (Torque = Lever Arm x Force), as shown in Fig. 9–7. The elevation force with a large ball is greater and proportional to the length of the radius.8 Grammont conducted studies on a deltoid simulator and calculated that Figure 9–6 (A,B) The seven portions of the deltoid; in a normal shoulder, only the middle deltoid (portion III) and part of the anterior deltoid (portion II) are lateral to the elevation axis, and can participate to active elevation (C); in a shoulder with a reverse prosthesis the axis of elevation is displaced medially which allows some of the anterior and posterior deltoid fibres (portion I and IV) to become more effective elevators (D). –A 10-mm medial displacement of the center of the sphere on the glenoid increases the abduction moment of the middle deltoid by 20% at 60 degrees of elevation. –A 10-mm inferior displacement of the center of the sphere on the glenoid increases the abduction moment of the middle deltoid by 30% at 60 degrees of elevation.9,10 From a functional standpoint, according to Fick,11 Strasser,12 and Kapandi13 the deltoid can be divided into seven parts (Fig. 9–6 A,B): –The anterior part, is inserted on the clavicula, and has two portions: I and II –The middle part is inserted on the acromion, and has only one portion: III –The posterior part is inserted on the spine of the scapula, and has four portions: IV, V, VI, and VII In a normal shoulder, if we consider that the axis of elevation is perpendicular to the plane of the scapula and is located within the humeral head (at the level of the center of rotation), we observe that only the middle deltoid (portion III) and part of the anterior deltoid (portion II) are lateral to this axis and can provide active elevation (Fig. 9–6 C). The other portions of the deltoid serve as adductors until humeral elevation progresses to the point that these different portions pass lateral to the sagittal axis and become elevators. Figure 9–7 The main principles of the Grammont reverse prosthesis are (1) a fixed and medialized center of rotation (C) reduces the torque on the glenoid component; to increase the deltoid lever arm (L), L2 is greater than L1; and (2) a lowering of the humerus relative to the glenoid to restores tension and augments deltoid force (F2 > F1). In a shoulder with a reversed prosthesis, the axis of elevation is medially displaced, located at the level of the glenoid surface (the center of rotation). In this situation, we can observe that, in addition to the middle deltoid (portion III), almost all the anterior (portions I, II), and even part of the posterior deltoid (portion IV) become elevators from the start of the movement (Fig. 9–6D). Nevertheless, medializing the center of rotation also medializes the humerus and the relative position of the deltoid insertion. This means that the deltoid muscle lever arm will be effectively decreased and weakened unless the upper arm is also lengthened, restoring tension. Grammont even recommended overtensioning the deltoid, slightly, to increase its force, as demonstrated by the increased acro miohumeral distance after insertion of a reverse prosthesis (Fig. 9–7). In addition, as emphasized by DeWilde et al,14 the elevation force is a function of the moment, a product of the muscle force and its lever arm, and as little as a 10% increase in muscle length can improve the moment; furthermore, the moment arm can be improved by the relative increase in distance between the deltoid line of action and the center of rotation. Stability is provided by (1) the large prosthetic head (Fig. 9–8)—dislocation requires displacement superior to the radius of the head, as in a hip replacement where a large head is more stable than a small 22-mm head;15 and (2) the increased compressive force of the anterior and posterior deltoid as a result of lowering the humerus (as described above). Grammont calculated that with the Delta prosthesis, compressive forces become superior to shearing forces beyond 45 degrees.9 Figure 9–8 Improved stability—as in the hip, a large prosthetic head is more stable than a small one. Improved stability is also provided by the increased compressive forces (C) of the anterior and posterior deltoid, as a result of lowering the humerus.
The Problem
The Vision
The Realization
The Deltoid and Grammont’s Design
Biomechanics of the Grammont Design
Stability
Reduced Torque on the Glenoid
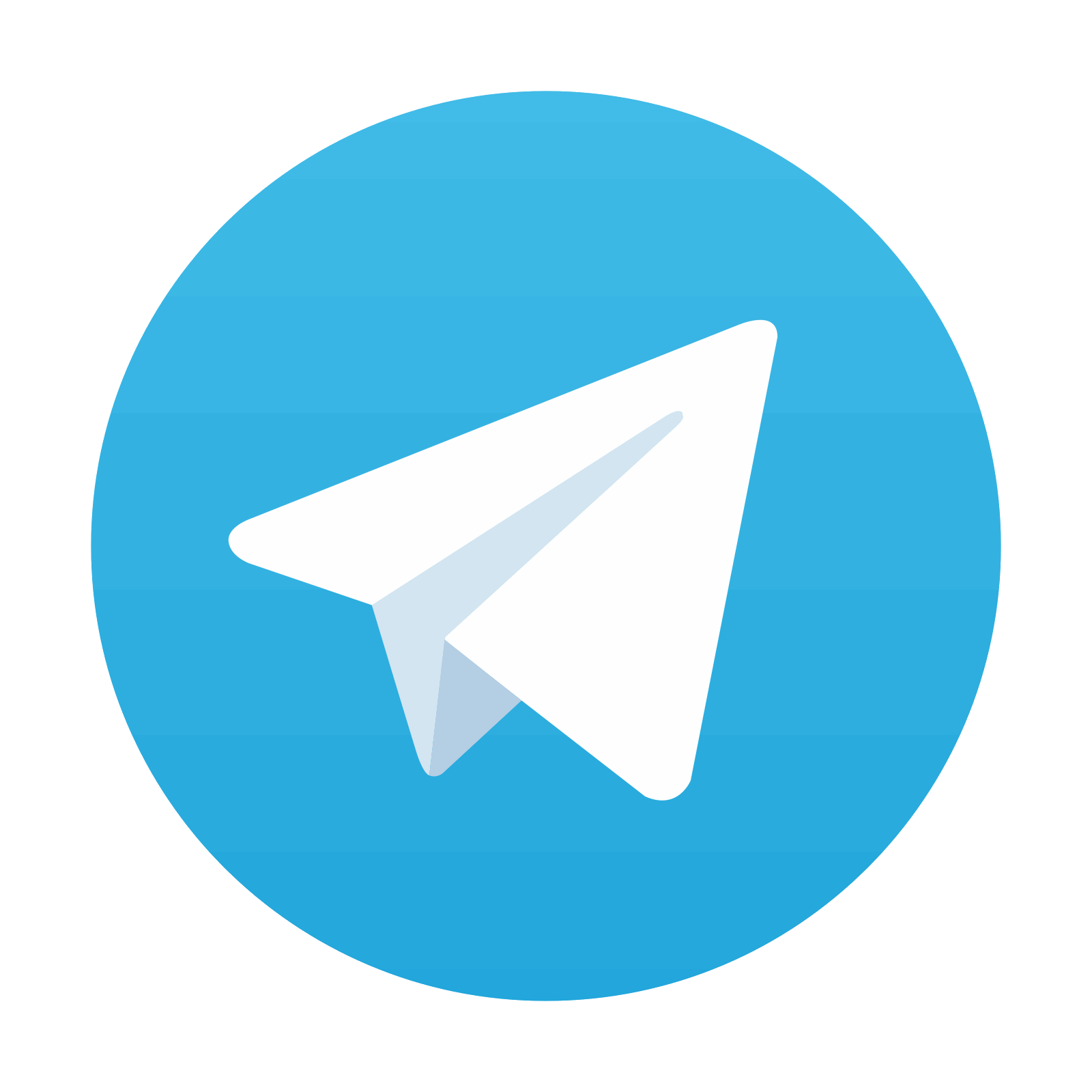
Stay updated, free articles. Join our Telegram channel

Full access? Get Clinical Tree
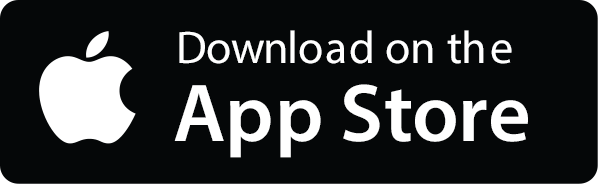
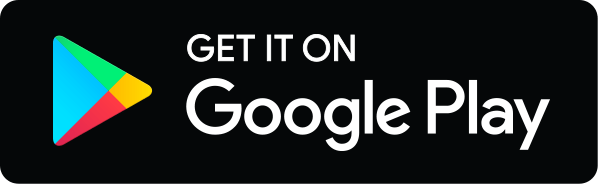