Fig. 4.1
RX AP flamingo view demonstrating (b image) a 3 mm left-upward symphysis excursion, indicating pubic instability in standard anterior posterior view (a image) and with monopodalic alternate bearing (b image)
An alpha angle greater than 50° measured on the Dunn projection or greater than 69° on the standard AP view establishes a radiologic diagnosis of cam FAI. Pubic instability is directly measured as the amount of vertical displacement (vertical offset greater than 2 mm) or widening (greater than 7 mm) observed at the symphysis on “flamingo” stress radiographs [2–4].
Ultrasound (US) is a valuable tool in focused assessment of both acute and chronic groin pain and allows for dynamic evaluation and, of course, is also very useful in image-guided diagnostic intervention. High-frequency linear array probes are used to perform musculoskeletal US examinations; during the examination, it is of paramount relevance to palpate with the probe on the skin the point of maximal tenderness [5]. To avoid artifacts or pitfalls, comparison with the opposite side is required. US is used in the initial assessment and diagnostic evaluation of tendons and muscle injuries including para-symphyseal tendinopathies or tears at one or more location, tendon snapping, or joint fluid in the younger athletes. In low-grade or chronic injuries of the adductor and hamstrings, US has a low sensitivity for detecting tenoperiosteal-entheseal disease. Especially the hamstring enthesis is difficult to assess with US because of its deep location. The capability of US real time imaging and the possibility to perform provocative stress maneuvers during the US scanning protocols, consent US-guided focused clinical examination; US therefore is a useful technique in integrated imaging protocol for depiction of clinically occult groin hernias or weakness of the posterior inguinal wall (e.g., sports hernia) [6].
Computed tomography (CT) imaging is a helpful imaging tool for the evaluation of bony abnormalities in athletes requiring surgical intervention (e.g., arthroscopic surgery); from the data set, images can be reformatted in other planes (2-D multiplanar technique) or be used for volume rendering (3-D technique) for a more effective display of complex anatomic and pathologic structures. Furthermore, collision detection algorithm when using 3-D models from CT scan can localize regions of anticipated mechanical impingement in symptomatic patients with hip pain requiring surgery [7].
Magnetic resonance imaging (MRI) of the pelvis as well as imaging of the groin and symphyseal region has proven to be the “gold standard” imaging modality in diagnosis of acute and chronic soft tissue injuries as well as radiographically occult and subtle fractures. The advantages of MRI include its multiplanar capabilities, excellent soft tissue and bone contrast, and lack of ionizing radiation. A closed-bore, high-field system (1.5 T or greater) will generate the best images. While a reasonably small field of view and high imaging matrix are desirable to facilitate higher spatial resolution (e.g., tendon attachments to the pubis), MRI protocol should include at least a screening examination of the entire pelvis to rule out visceral causes of groin pain. Musculoskeletal MRI commonly includes a combination of pulse sequences in both long-axis (sagittal or coronal) and short-axis (axial) planes. Axial oblique imaging perpendicular to the pubic bodies is a useful tool for depicting the tendon attachments and better separating the adductor muscles from each other. Short TR/TE (T1-weighted) images grant specificity for marrow abnormalities, sensitivity for muscle atrophy, characterization of blood products, and high-resolution depiction of structures that are surrounded by fat. Fluid-sensitive images (T2-weighted or STIR) are better used to identify the marrow and soft tissue edema that typify most pathology [1].
MR arthrography (MRA) is the current standard technique of advanced imaging for the evaluation of patients with suspected labral tears and chondral lesions. Limited data suggest that high-resolution unenhanced MRI, particularly performed at 3 T, may be as accurate as MR arthrography in the detection of labral and chondral tears. Large-FOV unenhanced MRI is far inferior to both MR arthrography and 3 T-unenhanced MRI in the detection of labral and chondral abnormalities of the hip [8]. A new technique called “traction MR arthrography” safely enables accurate detection and grading of labral and chondral lesions. Accuracy for detection of labral lesions reported was 92%/93%, 91%/83% for acetabular lesions, and 92%/88% for femoral cartilage lesions for reader 1 and reader 2, respectively [9].
4.2 Imaging of Common Hip and Groin Injuries in the Athlete
Sports-related injuries during the latest London Olympic Games had an overall injury rate of 12.9 per 100 athletes; types of injuries varied according to sport and athletes; soccer and ice hockey caused the greatest portion of injuries [10]. Cause of pain for discussion purposes can be summarized in hip pathology (e.g., femoroacetabular impingement, acetabular labral tears, cartilage pathology, loose bodies), soft tissue injuries including acute and chronic muscle-tendon pathology (anterior, medial, and lateral soft tissue injuries of the hip and groin), and skeletal injuries (acute/stress fractures, avulsions in skeletal immature athlete, osteitis pubis, and symphysis pubis arthropathy). Other differential diagnoses including visceral pathology (inguinal hernia, urogenital pathology) and nerve entrapment syndrome pathology should be considered in evaluating these athletes. This chapter examines the imaging features of the most common acute and chronic hip and groin injuries.
4.3 Hip Pathology
4.3.1 Femoroacetabular Impingement
In the athlete, FAI is a major cause of hip pain, reduced range of motion, and decreased performance. FAI has become increasingly recognized as a disorder that can lead to progressive labral and chondral injury and early hip degeneration. The most common structural deformities that lead to dynamic mechanical overload are a loss of femoral head-neck offset (“cam” impingement, Fig. 4.2), a focal or global acetabular overcoverage (“pincer” impingement, Fig. 4.3), and a combination of impingement deformities [11].



Fig. 4.2
RX AP view demonstrating cam FAI with nonspherical femoral head shape (pistol grip deformity)

Fig. 4.3
RX AP view demonstrating pincer FAI with crossover sign (COS). The COS testifies the overlap between the anterior and the posterior wall of the acetabulum. The posterior acetabular wall is outlined in yellow and the anterior in red. In a hip with a normal anteversion, the line of the anterior wall remains medial to the line of the posterior wall; instead in acetabular retroversion, the line of the posterior wall crosses medially the line of the anterior wall
The anatomic alterations underlying impingement may be secondary to another insult to the hip including developmental dysplasia, Legg-Calvé-Perthes disease, slipped capital femoral epiphysis, as well as posttraumatic and postoperative deformities [12–14].
Cam impingement is the consequence of an abnormal femoral head-neck relationship resulting in an osseous bump lateral or anterosuperior (1–2 o’clock) position using a clockface orientation on the femoral neck. It is more common than pincer impingement and is characteristically seen in young male athletes. Abnormal osseous morphology underlying cam impingement includes decreased femoral anteversion, abnormal femoral head-neck offset, shallow taper between the femoral head and neck, nonspherical femoral head, femoral neck “bump” or osseous excrescence, pistol grip deformity, and generalized enlargement of the femoral head (coxa magna) [15–18]. Conventional radiographs are very useful in identifying many of these anatomic alterations; measuring the alpha angle has been described to quantify the atypical head-neck correlation (Fig. 4.4) [19].


Fig. 4.4
The alpha angle. This angle is used for identifying an abnormal femoral head-neck junction. A best-fit circle is drawn, which outlines the femoral head. The angle is calculated as the angle formed between line that bisects the femoral neck and the point where the femoral head protrudes anterior to the circle. The angle ACB should be less than 50°
In this variety of impingement during movement of hip flexion, internal rotation, and adduction, bony outgrowth or bulge of the femoral neck avoids the normal movement of the femur within the acetabulum. This results in a characteristic pattern of shear injury to the transition zone and adjacent acetabular articular cartilage. Labral tears secondary to primary cam impingement typically result in detachment at the transition zone cartilage rather than intrasubstance injury. The cartilage break begins at the margin of the joint and progresses more centrally (outside to inside delamination) as the damage advances [20].
Pincer impingement is not as commonly seen as the cam mechanism; it occurs during flexion and internal rotation movements in middle-aged females. The underlying pathoanatomical condition is caused by acetabular overcoverage. Osseous impaction along the anterosuperior or superior femoral neck with compression proceeds with injury of the anterosuperior labrum and a small rim of chondromalacia. Posteroinferior cartilage lesions are caused by “contrecoup” forces in pincer-type impingement. This condition may be seen with excessive acetabular retroversion, protrusio acetabuli, and coxa profunda [21, 22]. The primary site of injury is the labrum. It is “pinched” between the femoral neck and the acetabular rim, and the damage is usually intrasubstance tearing of the labrum; the secondary site of injury is articular.
The diagnostic imaging evaluation protocol for FAI should include an AP pelvis radiograph and an elongated neck (Dunn) lateral view of the affected hip [23]. The AP pelvis has been demonstrated to be a valid indicator of cranial retroversion in the presence of a positive crossover sign; this is defined with the anterior rim line being lateral to the posterior rim in the cranial part of the acetabulum and crossing the latter in the distal part of the acetabulum [24]. The Dunn view (at either 45° or 90° of hip flexion) provides an improved evaluation of the femoral head-neck geometry. This view allows for identification of the cam morphology and calculation of an alpha angle, which estimates the degree of asphericity of the femoral head.
MRI with or without gadolinium contrast of the affected hip will allow accurate delineation of the intra-articular and periarticular soft tissue structures, including the femoral and acetabular chondral surface, labrum, capsule, and surrounding extra-articular tendinous insertions. Advanced cartilage imaging including T2 mapping, T1 rho, and delayed gadolinium-enhanced MRI of cartilage (dGEMRIC) techniques has allowed for improved quantitative assessment of cartilage integrity. Limited data suggest that high-resolution unenhanced MRI, particularly performed at 3 T, may be as accurate as MR arthrography in the detection of labral and chondral tears. Large-FOV unenhanced MRI is far inferior to both MR arthrography and 3 T-unenhanced MRI in the detection of labral and chondral abnormalities of the hip [8]. A new technique called “traction MR arthrography” safely enables accurate detection and grading of labral and chondral lesions. Accuracy for detection of labral lesions reported was 92%/93%, 91%/83% for acetabular lesions, and 92%/88% for femoral cartilage lesions for reader 1 and reader 2, respectively [9].
A CT scan with three-dimensional reconstruction is particularly useful for minimally invasive techniques such as arthroscopic or mini-anterior approaches to surgical management of impingement. It also helps to delineate complex combined mechanical pathomorphology. Post-processing computer analysis of three-dimensional CT imaging can allow for analysis of conflict patterns within the involved hip. Computer-assisted modeling of surgical corrections can be used for preoperative planning with improved accuracy of surgical intervention [7].
An ultrasound-guided intra-articular analgesic and steroid injection into the hip may be used for both diagnostic and therapeutic purposes and is an important adjunct to the overall evaluation. Response to an intra-articular injection has been shown to be 90% reliable as an indicator of an intra-articular abnormality.
4.3.2 Acetabular Labral Tear
The underlying cause of the labral injury should be delineated. There appear to be five common causes of labral tears: (1) trauma, (2) laxity/hypermobility, (3) bony impingement, (4) dysplasia, and (5) degeneration [25]. The labrum is a rim of fibrocartilage that attaches to the base of the acetabular rim. It surrounds the perimeter of the acetabulum and is absent inferiorly where the transverse ligament resides. Labral tears can be traumatic or acute, chronic, and in some cases degenerative in nature. Loss of labral function can lead to overloading of the articular cartilage of the hip and may be a precursor of osteoarthritis in the hip. Associations between labral tearing and articular cartilage damage have been reported. The articular lesions are most often located adjacent to the labral tear, often at the labral-chondral junction [26]. Direct MRA has been shown to be the best imaging modality for assessment of labral pathology [27, 28]. The labrum is triangular in shape, with its base attached to the acetabular rim and its apex extending laterally along the capsular side of the acetabular rim. The labrum demonstrates typical MRI features of organized collagen, with decreased low signal on T1- and T2-weighted images [29].
Most labral pathology occurs in the anterior and anterosuperior aspect of the joint. Tears are divided into three major groups: detached, intrasubstance, and degenerative. Labral detachments involve separation of the labral from the acetabular rim and are identified by contrast material interposed between the labrum and the rim (Fig. 4.5).


Fig. 4.5
MRI arthrogram of the hip demonstrating labral tears. (a) The white arrow demonstrates where the labrum is torn away from the acetabulum with well-defined fluid intensity lesion formation (anterior paralabral cyst). (b) The white arrow demonstrates where the labrum is partially torn away from the acetabulum
Detachments may be complete or partial, with or without a displaced fragment, and are best seen on coronal images in the setting of superior predominant tears and oblique axial images for anterior predominant tears.
Intrasubstance labral tears classically demonstrate intrasubstance fluid or contrast signal, usually extending to the articular side of the labrum, which is often oblique or curvilinear in shape. However, the signal may also be complex and extend in multiple directions in the long and short axis of the labrum. Increased signal alone within the labrum can be seen without frank fluid or contrast signal in the setting of labral tears with opposed surfaces [30].
A labrum with abnormal irregular contours and a thin morphology, with or without intrasubstance fluid or a contrast signal that extends to the free margin, is considered a degenerative-type tear. In athletes, it is not uncommon to have a combination of detached or intrasubstance tears with superimposed degenerative components.
4.3.3 Loose Bodies
Loose bodies within the joint can cause pain and may mimic the snapping hip phenomenon. Anterior groin pain, episodes of clicking or locking, buckling, giving way, and persistent pain during activity suggest an intra-articular loose body. Loose bodies within the hip, whether ossified or not, have been correlated with locking episodes and inguinal pain [31]. Besides hip trauma, other diseases known to be associated with loose bodies include Legg-Calvé-Perthes disease, osteochondritis dissecans, avascular necrosis, synovial chondromatosis, and osteoarthritis. Imaging with CT scan versus MRI should be thoughtfully assessed depending on the most likely diagnosis. If the loose particles are more likely to be chondral or soft tissue, then MRI is more likely to yield useful diagnostic information. The use of cartilage-sensitive MRI sequences (e.g., T2 mapping, T1 rho, and delayed gadolinium-enhanced MRI of cartilage (dGEMRIC) techniques) for the detection of lesions, therefore, may be preferable, although CT scans are more sensitive than MRI for detection of suspected bony loose bodies [32].
4.4 Soft Tissue Injuries of the Hip and Groin in the Athlete
4.4.1 Adductor-Rectus Femoris—Hamstring Injuries
Acute muscle injury occurs as a result of two mechanisms. Direct blunt trauma or compressive injury results in contusions and hematomas. Movements, where the muscle is being stretched through forceful contraction, lead to muscle strains and ruptures.
In most cases, the lesion is close to the musculotendinous junction, but in some cases, the tendon itself or the enthesis where the tendon inserts into the bone is the site of the injury. The three most common acute musculotendinous injuries in the hip and groin region occur in the adductor muscles (usually the adductor longus), the hamstring muscles (most often biceps femoris), and the quadriceps muscle (most often the rectus femoris).
In other cases, hip and groin injuries have the characteristics of an overuse injury, and the exact moment of injury can be hard to establish.
Adductor strains (pectineus, brevis, longus, magnus, and gracilis) occur primarily in the proximal portion of the adductor longus, at the muscle-tendon junction, or at the insertion of the pubic bone itself. The adductor longus and gracilis muscle enthesis are the most affected (Fig. 4.6a, b).


Fig. 4.6
(a) MRI coronal FWGE SAT and (b) MRI coronal STIR demonstrating complete detachment of adductor longus muscle enthesis with hematoma formation
The microtearing pattern is more often related to the development of chronic groin pain but may come early as complete rupture. In low-grade injuries, US has demonstrated a low sensitivity for detecting partial strain or tenoperiosteal disease. In these cases, MR imaging displays more reliably the pathoanatomy process. On the other hand, the US diagnosis of complete detachment of both muscles from their insertions is simple.
The rectus femoris has two heads, the direct originating from the anteroinferior iliac spine (AIIS) and the indirect originating from the superior acetabular rim and hip capsule. Proximal tears occur less frequently than tears in the midsubstance of the muscle belly involving the central aponeurosis or at the distal myotendinous junction. Rectus femoris tendon ruptures either at its insertion into the bone with detachment of bone fragment (avulsion from the apophysis in skeletally immature patients) or at the proximal myotendinous junction involving its direct head. In adolescent, acute pain proximally at the muscle origin indicates an apophyseal injury and must be high on the checklist of differential diagnosis. Partial tendon tears are more common than complete detachments. Tears affecting the distal insertion of the muscle fibers into the deep distal aponeurosis seem more common (Fig. 4.7a, b) [33].


Fig. 4.7
(a) US short-axis rectus femoris and (b) US long-axis rectus femoris. Central aponeurosis tears of the rectus femoris with large hypoechoic area (circle) associated with anechoic fluid collection (arrow) reflecting hematoma formation
The proximal hamstring complex has a strong bony attachment on the ischial tuberosity. The footprint on the ischium is composed of the semitendinosus and the long head of the biceps femoris beginning as a common proximal tendon footprint, with a distinctly separate semimembranosus footprint. Hamstring tendons can be injured following chronic microtrauma or a single acute injury. The proximal insertion of the long head of the biceps femoris and the semitendinosus is more commonly involved than that of the semimembranosus (Fig. 4.8a, b).


Fig. 4.8
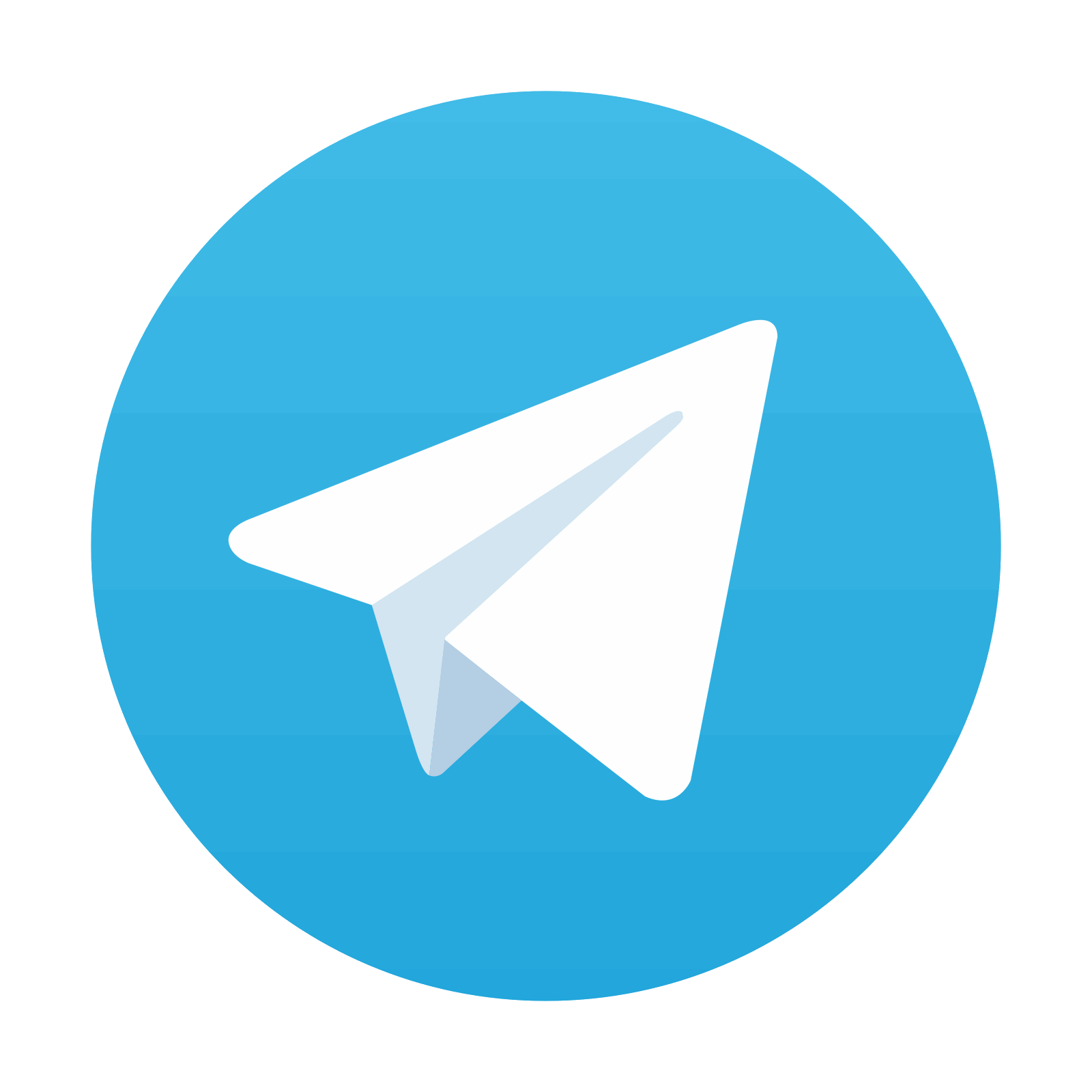
(a) MRI sagittal FWGE T2* and (b) MRI axial STIR slight thickening (arrow) of proximal insertion of the long head of the biceps femoris and the semitendinosus with small partial hyperintense intrasubstance tear (arrow)
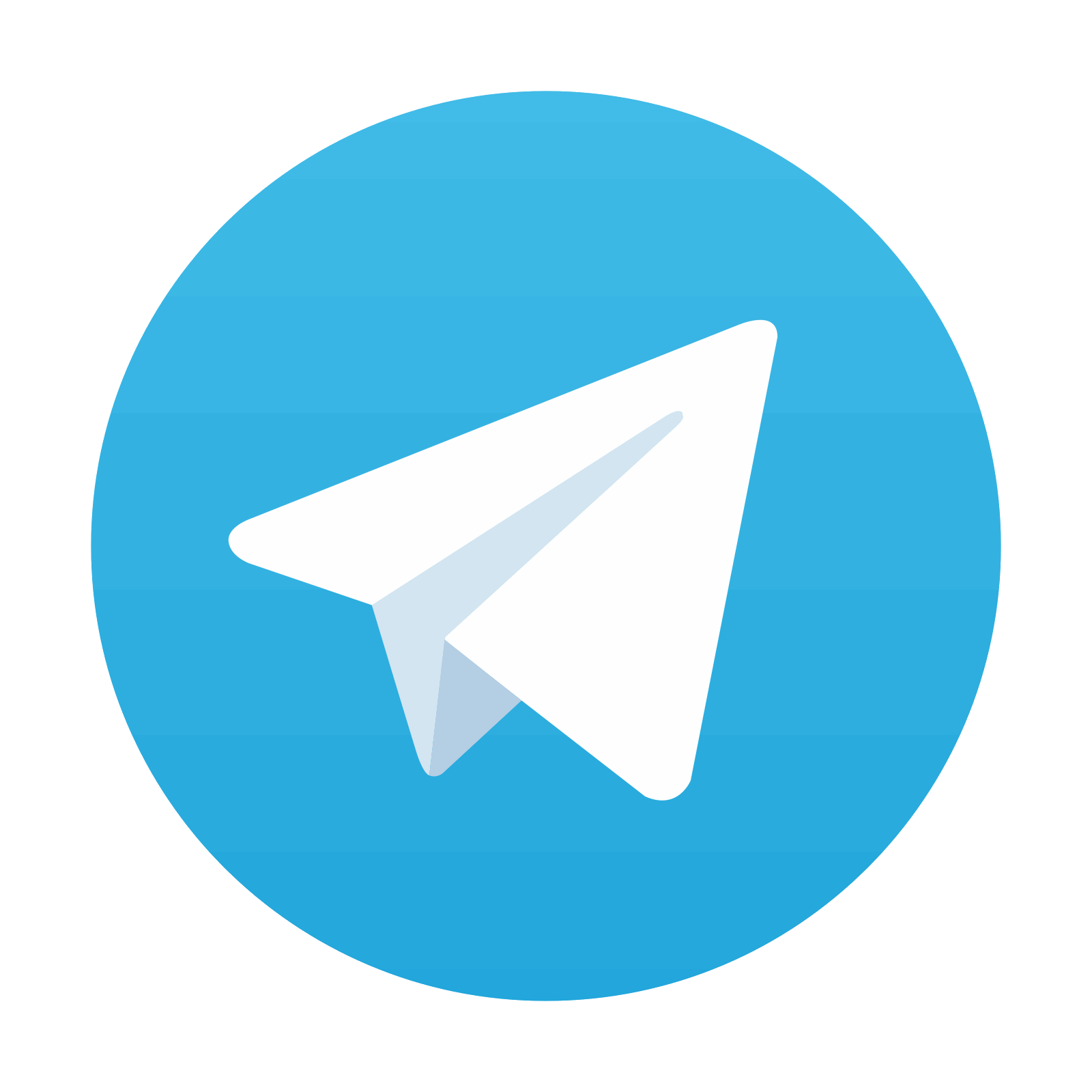
Stay updated, free articles. Join our Telegram channel

Full access? Get Clinical Tree
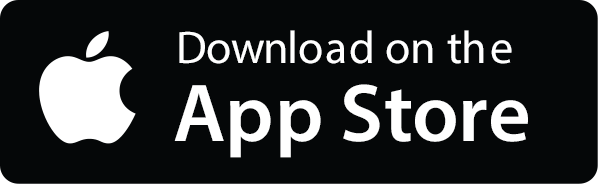
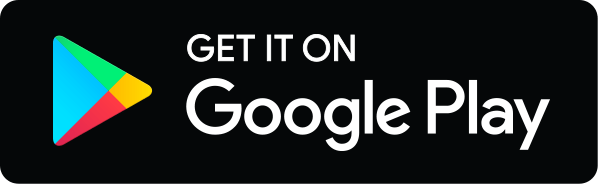
