21 Radiation Exposure: How Does Radiation Exposure Differ between Open and Minimally Invasive Techniques? Since 1980, there has been a 600% increase in medical radiation exposure to the U.S. population which is mostly attributable to radiological diagnostic studies. As compared to 15% in the 1980s, medical imaging comprises 50% of the per capita radiation dose.1 It has been shown that patients receive extremely high doses of radiation for diagnostic procedures alone during the average trauma admission, and even more during invasive procedures.2 These imaging modalities have provided much benefit to the patients, but at the same time have likely resulted in cancer in some. Routine use of fluoroscopy in musculoskeletal procedures became popular beginning in the early 1980s.3 Studies have shown that the amount of radiation exposure is 10 to 12 times greater during spine surgery than other fluoroscopically assisted musculoskeletal procedures.4,5 This higher exposure is related to both the amount of energy required to penetrate the torso, which is thicker than the limbs, and the proximity of the surgeon’s hand to both the primary and backscatter sources of radiation during operative imaging.6,7,8,9 The amount of radiation necessary to image a patient undergoing spinal surgery adequately is also likely increasing, given this is directly related to the patient’s size, in particular their torso mass.5 This has been shown in numerous studies.10,11,12 It has been shown that fluoroscopy time and overall procedure time are greater in obese patients.13 There is an ever-growing dependence of spine surgeons on intraoperative fluoroscopy as technology and procedures advance. The amount of radiation emitted during fluoroscopy during spine surgery is far from negligible.6,7,8,14,15,16,17 Quality fluoroscopic images are needed for placing instrumentation during open procedures, but even more importantly during minimally invasive surgery (MIS) that is extremely reliant upon intraoperative imaging. The ability to obtain quality spinal images is of paramount importance to safely, and effectively, perform modern-day spine surgery. The development of minimally invasive approaches to the spine has afforded several advantages over traditional open techniques. Mechanistically, the benefits are in large part derived from the decrease in soft-tissue injury realized through muscle dilation and the use of tubular retractors as compared to subperiosteal dissection of muscle and traditional retractors.18 These improvements can be quantified clinically in terms of decreased length of hospital stay, decreased perioperative blood loss and transfusion rate, and decreased use of narcotics for pain control when compared to open approaches.19,20,21,22,23,24 Minimally invasive spine (MIS) surgeries are also associated with a decreased rate of infection.25,26 MIS surgery is not, however, a panacea and its potential downsides must be examined critically. MIS surgery, in general, relies more heavily on image guidance than open spine surgery, and this typically means more use of intraoperative fluoroscopy. Thus, the benefits of less invasiveness notwithstanding, there is appropriate concern regarding the potential health impact of increased radiation exposure that may come with the utilization of MIS techniques. Traditionally, image guidance in MIS surgery has been achieved with X-ray fluoroscopy. This is a source of ionizing radiation and carries potential long-term health hazards. Today, the use of intraoperative imaging systems integrated with operative instruments has reduced the amount of fluoroscopy needed for a given case, thereby reducing occupational exposure to X-ray radiation. However, since these systems use X-rays to generate their images, their contribution to the cumulative radiation dose a patient receives during their treatment needs to be considered. Ionizing radiation is a potential occupational hazard, but also exists as a part of the environment through natural and man-made sources. Radiation sources include naturally occurring substances such as radon gas and uranium, man-made environmental modifications such as power plants and high-altitude aviation, and occupational/medical exposures such as receiving or working near X-ray producing machines or radioactive substances. Man-made exposures are considered to account for 18% of the average person’s radiation exposure, with the remainder attributed to natural sources. Thus, the risk of radiation exposure from medical treatment for most people is not considered to be significant. The same has not been true historically for occupational radiation exposure. Indeed, the relationship of cancer to radiation exposure became apparent, in part, after increased rates of cancer were noted in radiologists and radiology technicians who worked with early and improperly shielded X-ray equipment.27 In addition to cancer, other health problems have also been linked to radiation exposure, including skin damage27 and cataract formation.10,28 Today, occupational exposure to radiation and its associated health problems have been greatly reduced because of improved safety measures; however, concern still exists regarding the degree of risk from exposure to low levels of radiation over long periods of time. Similarly, the effects of radiation exposure from computed tomography (CT) scans are a concern for patients. These risks are difficult to quantify and are not really known. In the absence of such data, limits on radiation exposure are based on models that assume a linear drop-off between radiation exposure and cancer, and use this assumption to calculate risk from known cancer rates after much higher amounts of radiation exposure than one would likely ever encounter working in medicine. These models estimate that a threshold single whole-body dose of 100 mSv or 10 rems over background may be sufficient to produce one additional cancer in 100 adults over their lifetimes. The significance of this number with regard to medicine might be best understood in the context of patient exposures to imaging studies requiring radiation. Patient radiation exposure to a mammogram produces around 0.13 mSv or 0.013 rems of radiation exposure, whereas a whole-body CT scan can result in 12 mSv or 1.2 rems of radiation exposure. Thus, although the radiation effects of a mammogram are likely to be exceedingly low, the radiation effects of multiple whole-body CT scans are potentially significant.27 According to one estimate, the radiation dose threshold for a measurable, statistically significant increase in solid cancer risk among individuals exposed to radiation from atomic bombs is between 50 and 100 mSv, a number that could be accrued over five whole-body CT scans. The health effects of daily or weekly exposures to much smaller amounts of radiation are even harder to conceptualize with the data that currently exists. The kind of large, long-term population studies that would be required to answer these questions do not exist. Current Occupational Safety and Health Administration (OSHA) guidelines limit occupational exposure of 1.25 rems per quarter or 3 rems in a year to the “whole body” which includes head and trunk, blood-forming organs, lens of the eye and gonads, 18.75 rems/quarter to the extremities (hands/forearms, feet/ankles), and 7.25 rems/quarter to the skin.29 Radiation exposure during spine surgery can be thought of separately as the radiation dose received by the patient and the doses received by the operative team. For the patient, the radiation doses are expected to be higher, but these higher doses are tolerated because, with notable exceptions, the patient is unlikely to have considerable radiation exposure outside of their medical treatment. For the operative team, limiting radiation exposure on a daily basis is very important, and is significantly mitigated by wearing appropriate protective gear and removing or distancing oneself from X-ray sources as much as possible. In spine surgery, radiation is generated from two sources: intraoperative fluoroscopy and intraoperative imaging systems that use X-rays to generate three-dimensional (3D) images for navigation. For the latter, images are stored for intraoperative use, making it possible for the operative team to remove itself to an adjacent room while the scan is being performed. Under these conditions, the exposure to the operative team is negligible.30 The effective radiation dose to the patient during a lumbosacral scan, according to measurements using a popular system’s lowest recommended settings, is between 2.25 and 6.83 mSv depending on the size of the patient. This is between 81 and 85% of the amount of a similar scan performed on a 64-slice CT.31 When fluoroscopy is used, radiation exposure to the patient is less than if a 3D imaging system is used, but the exposure to the operative team is much more because it is not possible or practical for the operative team to remove itself from the operating room every time an X-ray is taken.32 Assuming that proper shielding garments are worn, the two most important variables determining occupational X-ray exposure from a spine surgery are the distance of the exposed body part to the side of the fluoroscope that contains the X-ray source and the time of exposure. The area of greatest exposure is the scatter zone on the side of the patient nearest to the X-ray source. The decrease in radiation exposure as distance from the source is increased is logarithmic. Distancing oneself just 35 cm away from the patient–X-ray interface will reduce the exposure three- to sevenfold depending on the size of the patient.5 Thus, highest radiation exposure occurs when a given surgical technique requires the surgeon to hold an instrument in the operative field while standing on the side of the X-ray source, particularly if the technique requires multiple X-rays or live fluoroscopy. A 55-year-old woman with a several-year history of progressive back and leg pain presented with an acute foot drop and worsening leg pain despite nonoperative management. Magnetic resonance imaging demonstrated grade 1 spondylolisthesis at L4–L5, associated with disc space collapse, and severe central canal and foraminal stenosis ( Following prone positioning on a Jackson operating table, fluoroscopic localization was utilized to demarcate the correct level and to place tandem, paramedian 3-cm incisions, approximately 4 cm off midline. The level was localized with X-ray by clamping a Kirschner wire (K wire) to the drape adjacent to the patient’s iliac crest, with the surgeon standing behind the X-ray source (surgeon out of field, SOF). After skin incision, the fascia was incised with a monopolar cautery and the facet palpated through the muscle. The K wires were then docked onto the ipsilateral facets and the level confirmed by X-ray (SOF). The turgor of the soft tissue is sufficient to hold the K-wires in place so that the surgeon stepped away during the X-ray. The muscle was dilated and the retractors were inserted, locked into position, and the final retractor position was confirmed by X-ray (SOF). Fig. 21.1 (a) T2-weighted midsagittal image of the lumbar spine demonstrating degenerative spondylolisthesis at L4–L5. (b) T2-weighted axial image through the L4–L5 disc space showing severe spinal stenosis in the same patient. On both sides, soft-tissue dissection was performed to expose the L4 lamina, the L4–5 facet, the L4 pars and transverse process, and the base of the L5 transverse process. A left-sided L4 laminotomy was performed with Kerrison rongeurs. An osteotomy was made in the left L4 pars and the articular process was removed. The osseous component of the stenosis decompression was completed using Kerrison rongeurs on the ipsilateral side. On the contralateral side, a drill was used to remove the base of the L4 spinous process, the ventral L4 lamina, and the medial L4–5 facet. Finally, the ligament was freed from its bony attachments with curettes and removed using pituitaries and Kerrison ronguers. This part of the case required no fluoroscopy. Next, the transforaminal lumbar interbody fusion (TLIF) was performed. An osteotome was used to remove L5 superior articulating process just superior to the L5 pedicle. The inferior edge of the exiting left L4 root was seen. Superior to the pedicle, the disc space was entered and a total discectomy was performed, and endplates were prepared. The disc space was irrigated. An X-ray was then performed with a rotating shaver in place to assess graft size. Contact with the endplates is enough to hold the shaver in position without needing to be held by the surgeon (SOF). A trial was selected, placed, and evaluated with an X-ray (SOF). The disk space was filled with arthrodesis material and a cage was placed. Several X-rays were used to adjust cage placement (SOF). We utilized image guidance to place pedicle screws. A reference arc was clamped to the L2 spinous process through a small separate incision, and an O-arm (Medtronic) was performed to generate an intraoperative 3D image with which to navigate pedicle screws. The entire staff vacated the operating room for the 15-second scan. The pedicle screw starting points were clearly seen under direct vision and pedicles were cannulated using a navigated gearshift probe. The cannulations were verified using a ball-tipped probe. K wires were then placed into the cannulations, and cannulated taps were navigated through the pedicle isthmi. A ball-tipped probe again verified cortical integrity. On the right side, a posterolateral fusion was performed by decorticating the L4–5 facet joint and the L4 pars and transverse process. Arthrodesis material was placed. The retractors were collapsed and removed, and the pedicle screws were navigated into place. Rods were passed through the screw extensions and their placement was confirmed with fluoroscopy after provisional tightening of locking screws (SOF). The locking screws were tightened finally. Another 3D spin was performed to confirm construct placement. Postoperatively, a CT scan was not required because its equivalent had already been performed intraoperatively. The patient will be followed up with plain radiographs ( The patient was taken to the operating room and general endotracheal intubation was performed. The patient was then turned prone using a Jackson table turn with the head placed in a prone view head holder. Pillows were placed under the femurs and tibiae to produce lordosis and prevent decubitus ulcers. The upper extremities were positioned on arm boards that were padded to prevent neuropraxia. Positioning should allow for optimization and ease of intraoperative fluoroscopic imaging to confirm spinal alignment and level and implant position/reduction. Following a posterior midline exposure over the L4–L5 interspace, using the iliac crests as an external marker, a Kocher clamp was placed on the spinous process of L4. This was confirmed with a single lateral fluoroscopic image. The erector spinous muscles were then subperiosteally elevated to expose the posterior elements of the spine bilaterally out to the transverse processes of L4 and L5. Self-retaining retractors were placed deeply and anatomic landmarks for pedicle screw placement were identified.
21.1 Introduction
21.2 The Dangers of Radiation Exposure
21.3 Case Illustration
Fig. 21.1). Surgical intervention was recommended to decompress the neural elements and stabilize the L4–L5 motion segment.
21.4 Surgical Technique in Minimally Invasive Surgery
21.4.1 Localization and Retractor Placement
21.4.2 Decompression and Interbody Fusion
21.4.3 Screw Placement and Posterolateral Fusion
Fig. 21.2) at 6 weeks, 6 months, 1 year, and 2 years postoperatively. The cumulative fluoroscopy time was 44 seconds and the radiation exposure was 0.29 mSv to the patient. The exposure to the surgeon was not measured, but reasonably expected to be a tiny fraction of this, given the surgeon was at least 3 ft away from the fluoroscope while any X-rays were taken. The two O-arm spins were estimated to produce an exposure of 7 mSv to the patient and negligible exposure to the operative team.
21.5 Surgical Technique in Open Surgery
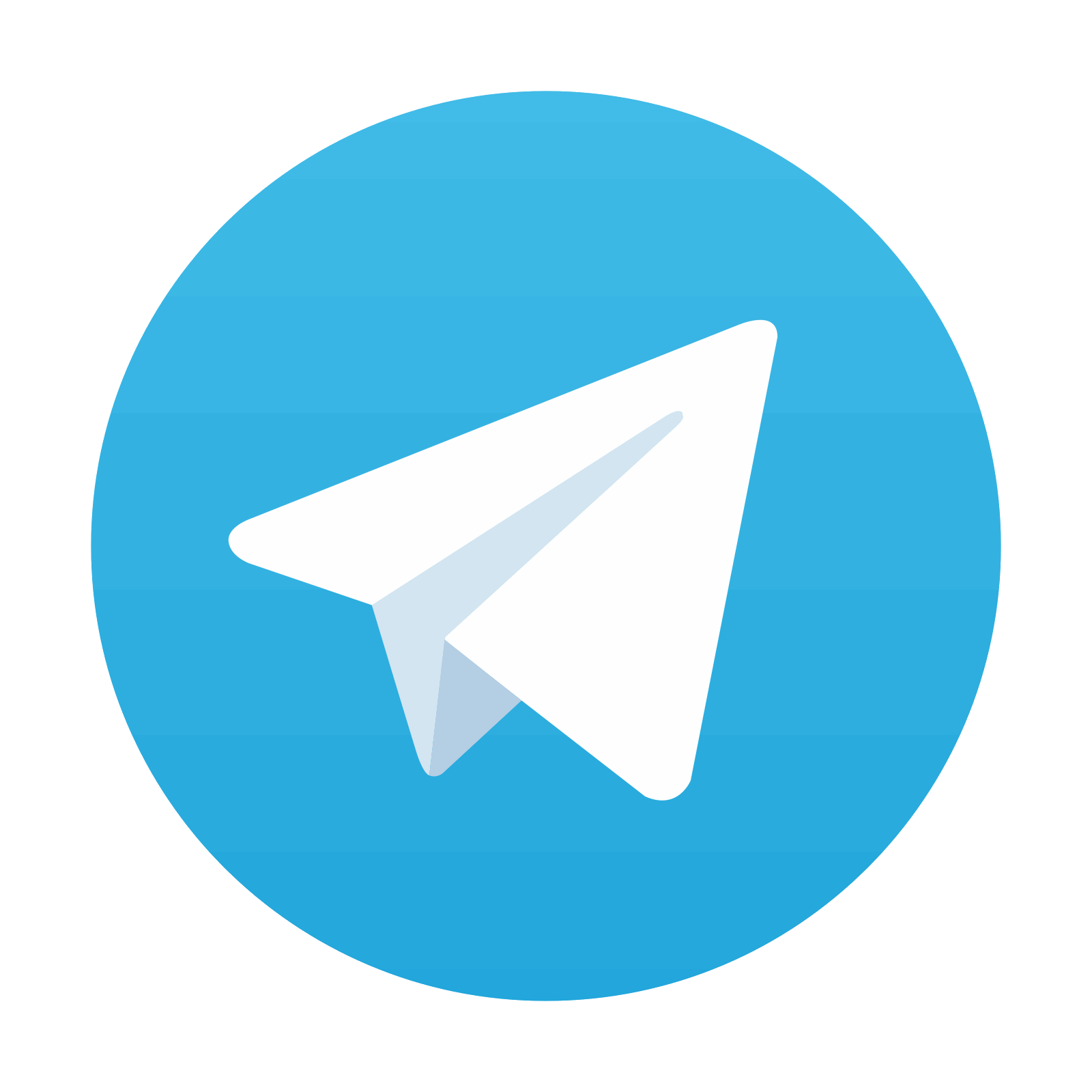
Stay updated, free articles. Join our Telegram channel

Full access? Get Clinical Tree
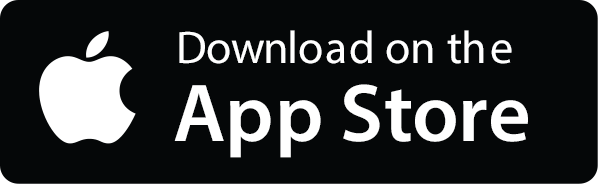
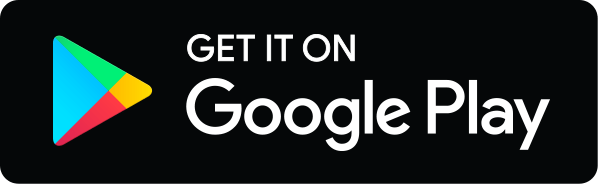