Fig. 10.1
(a) Schematic drawing showing the extensor-supinator muscles. BR: Brachiorradialis. ECRL: extensor carpis radialis longus. ECRB: extensor carpis radialis brevis (responsible for most of the pathology). ECD: extensor digitis comunis. ECU: extensor carpis ulnaris. (b) Anatomic specimen showing diseccion of the tendons. Notice the deeper location of the ECRB to the ECRL on the surface
The tendinous degeneration commonly referred to as tendinosis appears to be the end result of the inability of tenocytes (fibroblasts) to maintain the extracellular matrix in physiological conditions, mainly due to disruption of the remodeling/degradation (anabolism/catabolism) balance. This alteration of the extracellular matrix also affects the metabolism and fibroblast activity, thus perpetuating a vicious circle. There have been major immunohistochemical advances and gene expression analysis of pathological tendons showing proinflammatory mediators such as interleukin-1α, interleukin-β, TNF-α, as well as immunocompetent cells that may contribute to tendon inflammation [27]. In addition, extracellular matrix fragments stemmed from the breakdown of tenascin and hyaluronan may act as triggers of tendon resident macrophages, thereby unleashing an inflammatory response [27].
These intrinsic and extrinsic factors may determine the workload threshold beyond which the metabolic remodeling ability of tenocytes (fibroblasts) is insufficient to maintain an extracellular matrix. That can adapt to the higher level of mechanical stress resulting from the activity undertaken (allostasis). The most important factors that affect the disruption of the balance are shown in Fig. 10.2.
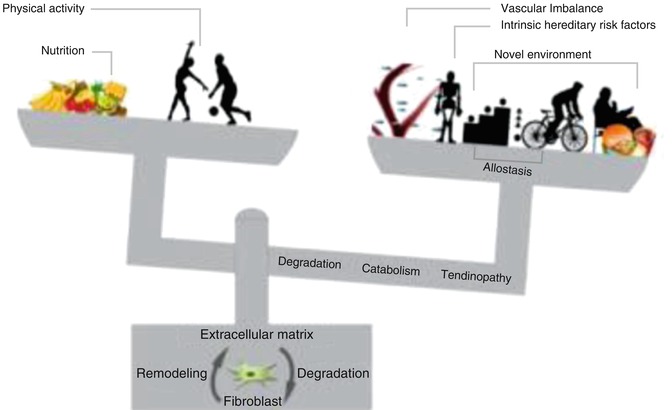
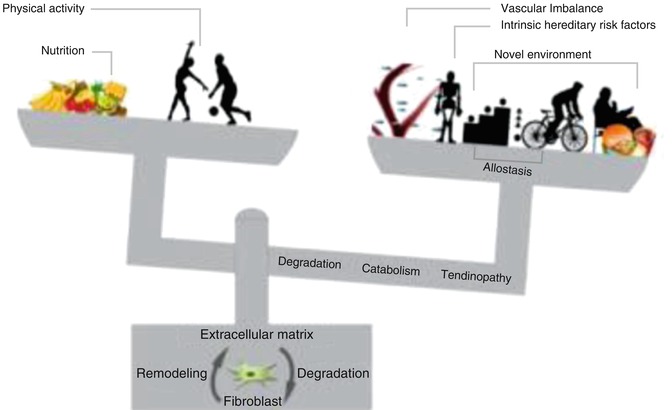
Fig. 10.2
Tendinopathy fisiopathology theory. “With permission of authors of A new biological Approach to Orthopedic Surgery and Sport Medicine 1st Ed. Teamwork Media”
A number of pathophysiological hypotheses have been proposed to explain the underlying causal mechanism of tendinopathies. Thus, apoptosis, vascular changes, and pain-related inflammation have all been suggested by [28], whereas Alfredson and coworkers [29, 30] have described intratendinous lactate and glutamate alterations, as well as neovascularization phenomena. Thus, suggesting their metabolic/vascular/neural involvement in tendon degeneration, these mechanisms may interact in an overlapping manner (uncompensated vascularization, localized temperature increase, acidosis, new environment, and intrinsic factors).
10.3.2 Pathogenesis of Lateral Epicondylitis
In 1936, Cyriax [12] proposed that microscopic or macroscopic tears of the common extensor origin were involved in the pathogenesis of this condition. Thereafter, other investigators showed that the disease base is actually a degenerative tendinopathy [31–33] (añadir REFERENCIA).
The application of stress to a tendon normally leads to increased cross-linkage and collagen deposition [8]. When the rate of stretching and loads to the tendon exceed the tolerance of the tendon, a micro-tear results. Then the balance is lost, and the adaptation of the tendon to multiple micro-tears leads to tendinosis. Collagen within the tendons gets degraded because it is kept under high stress and it gets degraded in such quantity that the tenocytes are not able to replace it. From a mechanical point of view, these cumulative microtraumas result from repetitive wrist extension and alternating forearm supination and pronation [31, 32]. Histologically four stages are described that result from such repetitive microtrauma [8, 34, 35]:
Stage 1: It starts with an acute inflammatory response, which can sometimes resolve completely.
Stage 2: If the aggression is maintained, a concentration of fibroblasts, vascular hyperplasia, and disorganized collagen, known in conjunction with angiofibroblastic hyperplasia, can be seen histologically.
Stage 3: Continuous accumulation of pathological changes leads to structural failure. In this stage the tendon suffers partial or complete rupture.
Stage 4: To the characteristics described in stage 2 or 3, other changes such as fibrosis are associated, as well as soft matrix calcification within the disorganized loose collagen and hard osseous calcification.
In 1973, Coonrad and Hooper were the first to describe macroscopic tearing in association with the histologic changes within the ECRB [31]. Six years later Nirschl called these histologic findings “angiofibroblastic hyperplasia” [33] as he showed that those findings were characterized by disorganized, immature collagen formation with immature fibroblastic and vascular elements. The term used today is angiofibroblastic tendinosis [36]. Ultrasonographically, tendon thickening or thinning, focal areas of hypoechogenicity, tendon tears, calcification, and even bony irregularity can be demonstrated mostly in the stages 3 and 4 [3, 37].
10.4 Diagnosis
Epicondylitis causes pain and disability, both in general population and in athletes. In addition, it has an economic cost in terms of days off the working activity and training. Thus, proper diagnosis and treatment are of paramount importance. An accurate, detailed, and thorough history and physical examination, combined with appropriate imaging studies in case of need, are essential in understanding the mechanisms and pathophysiology of the injury and making a specific diagnosis [3, 33, 36].
10.4.1 History and Physical Examination
During the history it is advisable to ask the patient for those sporting activities or job circumstances that could cause or exacerbate the symptoms.
Clinically, LE is characterized by tenderness or pain over the lateral humeral epicondyle or, more typically, in the area where the common extensor muscles (specially the ECRB) meet the lateral humeral epicondyle. The patient may refer to a direct trauma to the lateral aspect of the elbow, but often the pain can be gradual and insidious. The pain often radiates down the forearm and unusually is proximal to the elbow. The intensity of the pain can range from intermittent and mild to constant and severe, affecting all daily activities.
The patient usually suffers weakness in grip strength that affects sports practice, working activities, and even activities of daily living as shaking hands, shaving, lifting, or raising a coffee mug.
It is recommended to rule out cervical spine pathology, followed by an examination of the entire upper extremity, with special attention to the shoulder, comparing with the unaffected, contralateral extremity. Palpation of the lateral humeral epicondyle or the origin of the ECRB will reproduce the pain.
A number of tests that could reproduce this pain, helping to the diagnosis, have been described:
Resisted third finger extension can be painful because of selective recruitment of the ECRB tendon (Maudsley’s test)
Resisted wrist extension with the elbow fully extended and in pronation stresses the whole common extensor origin and can reproduce the pain (Thompson maneuver).
Asking the patient to lift a chair with the forearm pronated recreates the combination described above and also causes lateral elbow pain (“chair test” or Gardner test, Fig. 10.3) [38–40].
Fig. 10.3
Chair test or Gardner test
Others like Bowden test, Cozen’s test, and Mill’s test can be helpful.
Generally, range of motion at the wrist and elbow is not affected. Grip strength may be decreased as a result of pain.
10.4.2 Imaging and Complementary Test
In most cases a diagnosis of lateral epicondylitis can be made clinically. The X-rays can be helpful in demonstrating calcifications in the soft tissue at or near the insertion of the ECRB (found in 25 % of the cases [41]). They are helpful to rule out other potential causes of pain (including loose bodies, osteoarthritis, and osteochondritis dissecans) (Figs. 10.4 and 10.5).
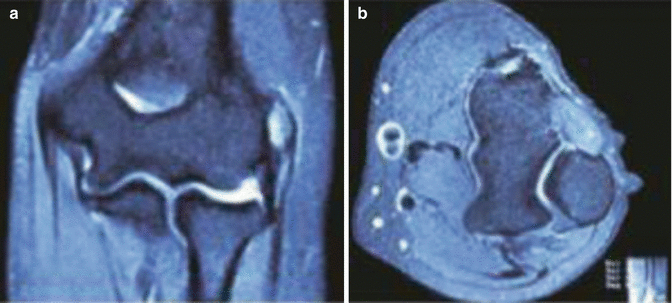
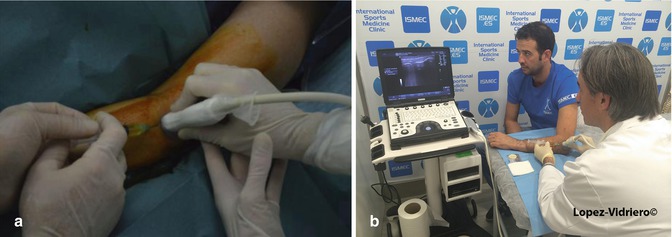
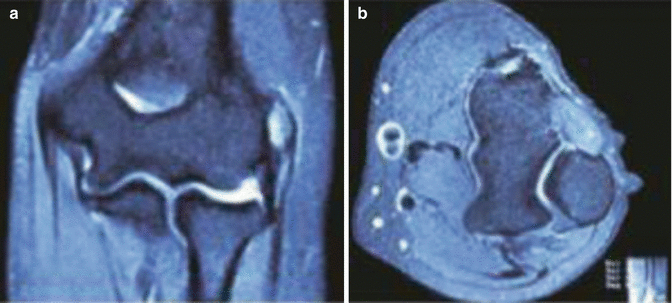
Fig. 10.4
MRI images of a lateral epicondylitis
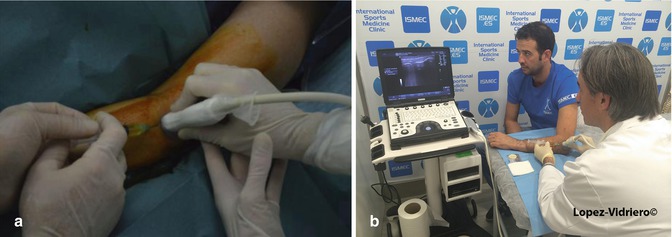
Fig. 10.5
(a) Ultrasound guided inyection with 4 hands. The surgeon injects while is helped by the radiologist using the probe. (b) Ultrasound guided injection with 2 hands. The surgeon triangulates by himself injecting with one hand while the other holds the probe. The skin is prepared with clorhexidine. Notice that sterile gel is used. The probe is protected with sterile latex sheath. The needle in the screen generates the typical reverberance due to the fact that is made of metal
Ultrasound imaging can be useful by identifying structural changes in the affected tendons (thickening or thinning, tendon tears, calcification, bony irregularity, etc.). Doppler ultrasound is able to detect neovascularization.
MRI can help to confirm diagnoses involving the extensor tendon origin. MRI has 90–100 % sensitivity and 83–100 % specificity for detecting epicondylitis [42]. Magnetic resonance imaging may also be useful if concomitant intra-articular pathology or ligamentous injuries are suspected.
Electromyography can be useful in excluding posterior interosseous nerve entrapment (radial tunnel syndrome).
10.4.3 Differential Diagnosis
Accurate diagnosis of lateral epicondylitis may be difficult since there are other conditions with similar symptoms (pain and reduced strength). Differential diagnosis for lateral epicondylitis has to include [3, 24, 33, 36]:
1.
Cervical radiculopathy with pain irradiated to elbow and forearm.
2.
Elbow overuse due to an ipsilateral shoulder malfunction (compensatory mechanism).
3.
Entrapment of the posterior interosseous nerve (PIN), also known as radial tunnel syndrome, which affects 5 % of LE patients, does not cause increased pain with resisted wrist extension (see Sect. 4.1). Pain may be caused by resisted forearm supination as the supinator muscle is one of the areas of compression of this nerve. Electromyography and local injection of anesthetic to the region of the PIN may relieve the pain [43].
4.
Joint problems: Ulnar collateral ligament injury, loose bodies, degenerative changes at the radiocapitellar joint, and osteochondritis dissecans. Rajeev and Pooley found 59 % of degenerative changes in 117 elbow arthroscopies performed for LE treatment [44]. It should be taken into account for the treatment of LE either in young or in middle-aged population.
5.
Infection or tumors around or within the joint may also mimic LE clinical features and sometimes could appear as a mass.
10.5 Treatment of Lateral Epicondylitis
The aims of treatment for LE should be:
Pain control
Preservation of movement-function of the joint and upper limb
Improvement in grip strength and endurance
Return to normal function and activity
Avoidance of further histological and clinical deterioration
Some studies have reported unpredictable healing patterns and have identified factors linked to poor outcomes. In this way, high baseline pain scores, manual work, and involvement of the dominant extremity have directly seen related to worse outcomes [14].
10.5.1 Described Treatment Options
Some authors have shown that the lateral elbow tendinopathy is a self-limited condition, and rest with or even without the use of some analgesic or anti-inflammatory medication in the acute phase of pain could resolve the symptoms.
The average duration of a typical episode is about 6 months to 2 years, but most patients (89 %) recover within 1 year [45].
To date, a standardized, universally accepted program for LE treatment has not been established by the orthopedic surgeon’s community [3]. It leads to a wide diversity of treatment ranging from an expectant waiting approach, nonsteroidal anti-inflammatory drugs (NSAIDs), physical therapies, bracing, acupuncture, laser therapy, extracorporeal shock wave therapy, percutaneous radiofrequency thermal lesioning, topical nitrates, injection of glucocorticoid, botulinum toxin, autologous blood injection, and platelet-rich plasma therapies to surgery.
The choice, as reported by some studies depends on experience, expertise, and equipment at any given clinic or center. What is quite clear is that patient education is usually one of the important core elements of any plan or protocol.
The evidence indicates that wait and see policy would be enough for most patients [45, 46]. Injection with glucocorticoids has been used since the 1950s and has been the treatment of choice for most of the physicians. However, nowadays its efficacy and utility are considered controversial, since some studies addressed that long-term outcome of steroid injections is poorer than expected and could even alter the ability to heal and damage the tendon and tissues around. It is reported that 72 % of patients treated with steroid injections experience a recurrence within 12 months, compared with 9 % in those treated with a wait and see strategy [47–49].
Furthermore, as confirmed by the systematic review by Dean et al. [50], the local administration of glucocorticoid has significant negative effects on tendon cells, including reduced cell viability, cell proliferation and collagen synthesis, collagen disorganization, and cell necrosis, leading to a reduction of mechanical properties of the tendon. This should mean that in case of planning an infiltration of glucocorticoids for any tendinopathy and the use of an ultrasound guidance would be of paramount importance to avoid intratendinous injection.
It is well established that surgery is reserved for patients who fail to respond to nonoperative treatments, and multiple variations on open approach as percutaneous and arthroscopic procedures have been described. Studies of Nirschl and Pettrone [33] are considering that a range of 4–11 % of patients ultimately could require surgical treatment for relief of their symptoms.
Until today, the evidence about surgical treatment for LE is lacking, and the Cochrane Library has classified surgical treatment as having insufficient evidence to support or refute its use [51]. Despite exhaustive nonsurgical management and even correct surgical intervention, there is a small percent of patients who continue to feel symptoms, usually in terms of pain. In such cases the possibility of a wrong initial diagnosis or an associated pathology should be considered and ruled out.
10.5.2 Biological Therapies for Tendinopathies: PRP
Despite the fact that there are many options to treat injured tendons, as described previously, it is a reality that none of those are foolproof and it leads to the need of further studies and investigations on pathogenesis of tendon damage to understand and develop new strategies of treatments.
Therefore, mainly in the last decade, minimally invasive interventions with the theoretical ability of boosting the healing response or neutralizing degenerative changes in tendinopathy have received the watchful eye of the community and are being investigated.
Among the emerging technologies, with “biologically friendly” or “regenerative” profile, autologous whole blood and platelet-rich plasma (PRP) have been recently used in several clinical studies for the treatment of LE. PRP is defined as “a sample of autologous blood with concentrations of platelets above baseline values” [52].
The management of musculoskeletal injuries with PRP therapies has been advocated since 2003 [53], when Sánchez et al. published, as far as we know, the first paper on the use of PRP to treat an articular osteochondral avulsion of the knee.
Since then, this promising and innovative technology has stimulated translational research and interest among both the scientific and medical communities and has widened PRP applications to several musculoskeletal problems [54–57].
In the other hand, the term “PRP” is wide enough to generate confusion. Due to the fact that different preparations, with unequal cell population and activation method, with different amount of platelets above peripheral blood baseline, and, even more, with a nonconsensual protocol of application, are being used and investigated under the name of PRP [54, 58–60], therefore, different attempts of classification have been described, and some authors [55, 59, 60] have proposed systems that try to classify PRP systems by activation mechanism, platelet number, and/or cell content. The absence of a validated classification system that identifies crucial differences between PRP formulations makes it difficult to compare studies, and it involves that, despite intensive research and huge number of publications in the last years, there is a gap in the basic knowledge necessary to establish the best PRP product for each clinical condition, as well as the guidelines for clinical applications [57].
There is some evidence to state that PRP formulations (number of platelets, presence of white blood cells (WBCs), balance between platelet secreted and plasma proteins, mechanism of plasma activation) and/or application procedures (i.e., number of doses, volume, activation, and injection procedures) could be linked to clinical effect [57, 61, 62]. In this way there are enough reasons to believe that the use of leukocyte-rich PRP (L-PRP) and leukocyte-depleted PRP (pure or P-PRP) should not be the same (L-PRP is more proinflammatory when injected in rabbits [63], it increases the levels of metalloproteases when assayed in tenocyte cultures [64], and it induced more transient postinjection swelling and pain when injected into the knee for treatment of knee osteoarthritis [65]). There are some trials performed with a combination of local anesthetics and PRP. An in vitro study by Carofino et al. concluded that the addition of either anesthetics or corticosteroids to PRP resulted in statistically significant decreases in tenocyte proliferation and cell viability [66].
Even more, there is no consensus about the frequency and number of PRP injections in chronic injuries. The majority of the studies have been performed with a single PRP application, but in our opinion a degenerative process could not be solved with just one intervention, and therefore, two or even three injections would be more efficient than a single PRP application, but, actually, this issue remains to be clarified [67].
Our group has been using from the beginning of our research a 100 % autologous PRP with a standardized composition and dosage (PRGF-Endoret, BTI, Vitoria-Spain). It contains a moderated platelet concentration (2- to 2.5-fold increase compared with peripheral blood) [57], obtained after a single spinning. One of the most relevant and controversial issues is the presence of WBCs in PRP. The first and most widely used classification would define the system we are using, as pure PRP (P-PRP) because it does not contain WBCs [59] The PRGF-Endoret is classified as type 4-B (minimal WBCs, activated with calcium chloride, and platelet concentration below 5) as proposed by Mishra et al. for sports medicine classification [55]. Finally, PRGF would fit in the P2-x-Bb category (platelet count greater than baseline levels to 750,000 platelets/mL, exogenous activation with calcium chloride, with WBCs, and specifically neutrophils, below or equal to baseline levels) according to the PAW (platelets, activation, and WBCs) classification [60].
10.5.2.1 The Scientific Rationale behind the Use of PRP Use on Tendinopathies and Lateral Epicondylitis
PRP preparations include growth factors, cytokines, and morphogens contained in platelets, as well as fibrinogen and other plasmatic proteins in a biologically balanced aggregate, managed and delivered in a pharmacological manner [68]. This may account for two special features: the resolution of inflammation and avoidance of fibrosis. In addition to containing GFs, PRP provides the damaged tissue with a transient biological fibrin scaffold, which stems from the polymerization of fibrinogen, a pleiotropic blood protein that regulates coagulation, inflammation, and tissue regeneration. PRP tendon infiltrations are aimed at recruiting, activating, and mobilizing satellite cells and resident macrophages which contribute to repair processes by cell signaling soluble factors. Once the activated preparation rich in growth factors is injected, this liquid-to-gel transition 3D injectable scaffold allows a successful filling of the tissue gaps and defects. With a local and gradual activation and a homogeneous distribution through and interaction with the ECM of tissue, it is converted into a matrix-like viscous and malleable structure [69]. This fibrin scaffold formed “in situ” as a provisional extracellular matrix and containing binding sites for cell adhesion as well as proteins such as thrombospondin-1 (TSP-1), alpha-1-antitrypsin fibronectin, acute phase proteins, or proteins related to lipid metabolisms [70] serves as a highway for mechanical energy to transit from the environment to the cell, thereby bridging cell-to-cell tissue transition, promoting multicellular assembly, providing mechanical support and plastic-elastic stiffness which has a drastic impact on fates of different cell types such as fibroblasts [71], and endowing tissues with a suitable mechanical and chemical microenvironment for biological restoration. In addition, fibrin matrix, by heparin-binding domains, may sequester growth factors such as PDGF, FGF, HGF, BBNF, and VEGF [72, 73] and gradually release them later, exerting a synergistic action on tissue repair.
Since this dynamic spongelike fibrin-matrix scaffold is autologous, bio-reabsorbable, biocompatible, and free of leukocytes and red cells, PRP scaffolds might be considered the best tailored among all the tissue engineering materials [74].
There is a great deal of evidence illustrating the anabolic effects of PRPs on tendon cells [75–78]. PRP stimulates the synthesis of several types of collagen and other oligomeric matrix proteins, resulting in a synthesis of extracellular matrix which is conducive to the tendon anabolism and homeostasis. The wide spectrum of cell response in vitro and in vivo in both tendon stem cell differentiation and tendon cell proliferation, together with a substantial expression of VEGF and HGF by tendon cells, thereby generating a balanced angiogenesis, constitutes the rationale for the application of activated liquid and fibrin scaffolds to the injured site of the tendon to prompt the repair events in one area that brings about a great deal of morbidity. The infiltration of activated liquid form of PRP to a tendon damaged area elicits a set of sequential remodeling events that might lead to the tendon healing. Although the TGF-B1 family drives fibrogenesis and potentially might stimulate the formation of scar tissue in the tendon, the fibrotic effect of TGF-B1 present in the PRP would be either modulated, counterbalanced, or even hindered by the presence and local production of VEGF and HGF, a potent antifibrotic and anti-inflammatory agent, as has been shown by our work on cells cultured on fibrin matrices [79].
10.5.2.2 What Does Evidence-Based Literature Say about PRP and Lateral Epicondylitis?
In the last decade and taking into account the promising role of PRP for LE established by Mishra et al. in 2006 [80], different research projects have been developed, comparing PRP to different classically accepted treatments for LE, as corticosteroids, local anesthetics, and autologous blood. To date, all controlled clinical trials in epicondylitis (nine) have been performed with L-PRP [80–88]. So far there are no direct comparisons between L-PRP and pure PRP. There are also two case series papers with 6 and 30 patients and a single injection.
PRP Versus Bupivacaine Injections
Mishra and Pavelko [80] were the first people performing a case-control study with PRP on patients (n = 20) in which nonsurgical treatment had failed. Fifteen patients were injected with PRP and the other five with bupivacaine, intending these to act as controls. They found a 60 % improvement in VAS in PRP arm at 8 weeks and a 93 % reduction in VAS and function at 24 months of follow-up.
This study opened a way of research on PRP effect for LE, but the real value of it is conditioned by the design itself. In 2014 Mishra et al. [87] published a multicenter randomized and controlled trial (RCT) on 230 patients (116 injected with PRP and 114 with bupivacaine) that had at least 3 months of symptoms and had failed conventional therapy. The injection site was blocked in both cases using 0.5 % bupivacaine with epinephrine, before injecting PRP. At 12 weeks no significant differences between PRP and bupivacaine were found. However, significant VAS improvement and also significant success rates (>25 % reduction in pain score versus baseline) at 24 weeks were encountered in PRP group.
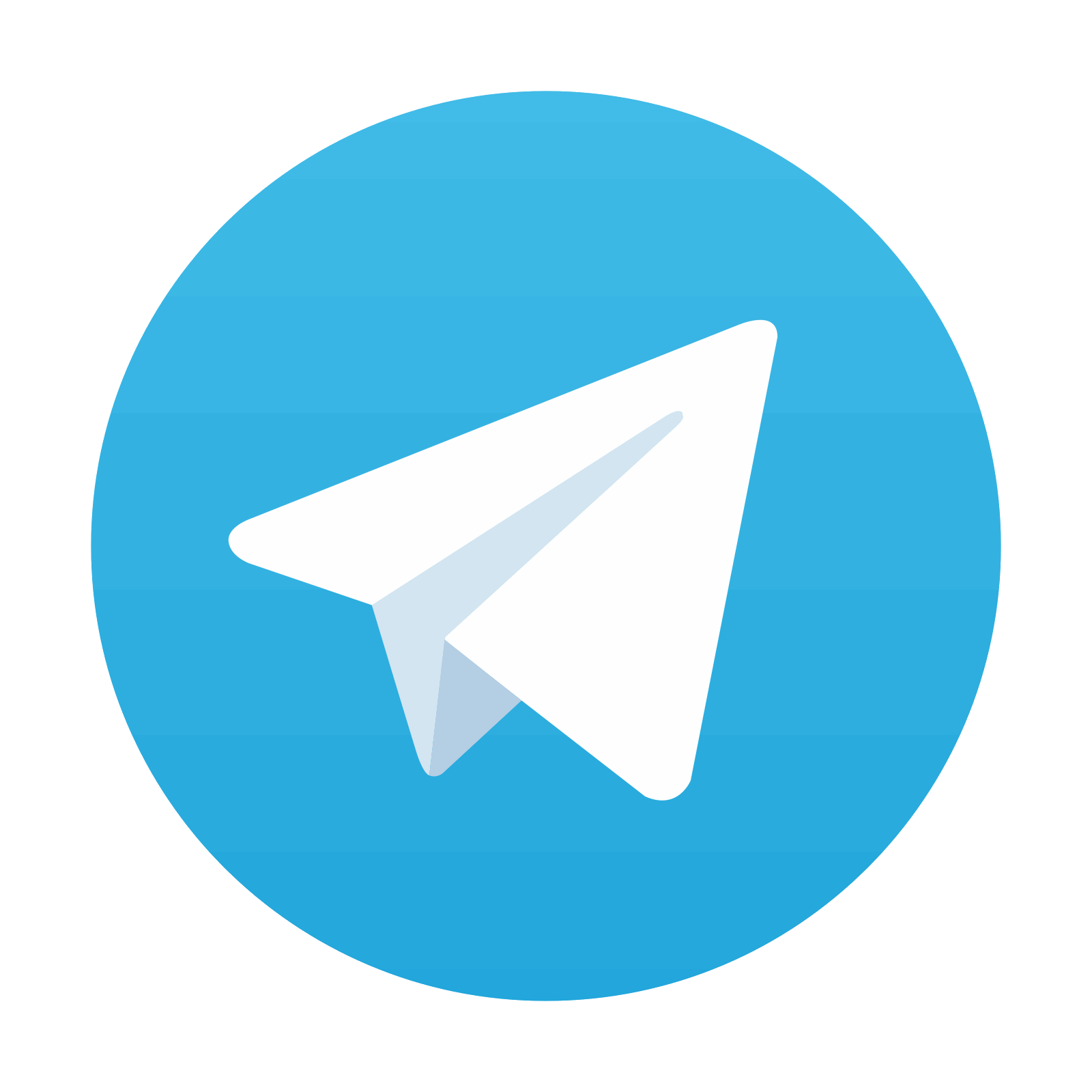
Stay updated, free articles. Join our Telegram channel

Full access? Get Clinical Tree
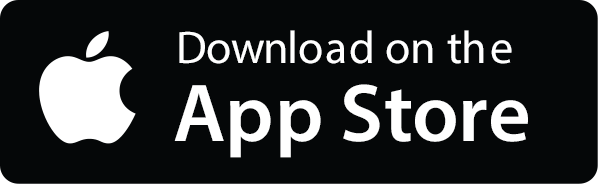
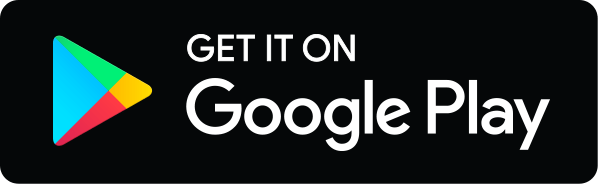
