Fig. 26.1
Interactions of ACL fixation devices and graft healing
There are three distinct phases of graft healing in ACLR, including cell repopulation to the graft, proliferation phase, and remodeling phase. It is generally agreed that the graft undergoes necrosis and shows hypocellularity, especially in the center of the graft. Cytokines like TNF-α and interleukin 1-β are released as a consequence of necrosis, which then trigger growth factors for cell migration, proliferation, extracellular matrix (ECM) synthesis, and revascularization [36]. Maximum cellularity is observed during the proliferation phase and the cell number surpasses that of the intact ACL in numerous animal models [62]. Mesenchymal stem cells and activated fibroblasts can be found at the periphery of the graft in bone tunnel region and the mid-substance region [33]. Cell numbers regress toward the intact ACL cellularity at the end of the proliferation phase. The tissue remodeling phase is started with cell-mediated restructuring of extracellular matrix as an adaptive response to mechanical loading on the tendon graft. Fixation devices inevitably affect these processes, and enhancement of graft healing could be achieved by introducing bioactive substances compatible to the surgical procedure. For examples, adding vitamin C into surgical irrigation saline can reduce graft necrosis and promote restoration in knee laxity in a rat model [19]. Intraoperative implantation of cells [37] or biomaterials [66] and postoperative injection of bioactive substances [20] can also promote graft healing in ACLR. These biological enhancements need to be compatible to the fixation device in use, and some bioactive substances could be engineered into the fixation device for a better outcome. Thus, fixation devices that enhance ACL biological healing may be the limelight of future development.
26.4 Femoral Versus Tibial Fixation
There are two key differences between femoral and tibial fixation. First, the bone mineral density of the proximal tibia is less than the distal femur. Bone mineral density has been shown to be directly related to interference screw fixation strength. Second, the line of force on the graft is directly in line with the tibial tunnel while it is obliquely oriented to the femoral tunnel in the weight-bearing position of extension [9]. For these reasons, the tibial graft fixation site is considered the weakest point in ACL reconstructions [1].
26.5 Pros and Cons of Different ACL Fixation Devices
ACL graft fixation devices can be broadly categorized as intratunnel, cortical button (“suspensory”), or extratunnel fixation depending on where the ACL graft is fixed (Fig. 26.1). In the next section, we will examine the issues related to the location of graft fixation, then review the merits and drawbacks of specific types of ACL graft fixation devices. A summary of the pros and cons of different graft fixation devices can be found in Table 26.1.
Table 26.1
Pros and cons of different ACL graft fixation devices
Fixation device | Metal interference screw (MIS) | Bioabsorbable interference screw | Screw and sheath | Cross-pins | Transfemoral suspensory pins | Cortical buttons | Spiked washer and screw | Screw and post | Staples |
---|---|---|---|---|---|---|---|---|---|
Pros | Excellent clinical track record | Equivalent clinical outcomes to MIS | Centralize the screw and increase graft to bone contact area in the tunnel | Simplify revision surgery | High load to failure | Excellent clinical track record | High load to failure | Reasonable load to failure, but less than other devices | Reasonable load to failure, but less than other devices |
Low complication rates | Simplify revision surgery | Higher radial forces than screw alone | Equivalent clinical outcomes with other devices | Equivalent clinical outcomes with other devices | Simplify revision surgery | ||||
Cons | Artifact on MRI | Risk of screw breakage, postoperative knee effusion, and tunnel widening | Limited clinical studies | Breakage may occur | Breakage may occur | Adjustable loop devices may lengthen | Limited clinical studies | Limited clinical studies | Limited clinical studies |
Complicate revision surgery | May require complicated hardware removal | May require hardware removal | May require hardware removal |
26.5.1 Graft Motion: Location of Graft Fixation
The motion of the ACL graft within a bone tunnel has been shown to be detrimental to graft healing [52] and may lead to tunnel widening [27]. Two types of graft-tunnel motion have been described, the “bungee effect” due to longitudinal graft motion and the “windshield wiper effect” due to transverse graft motion in the tunnel.
Rodeo and others used micro-CT to measure graft-tunnel motion in a rabbit model. They found graft-tunnel motion was greatest at the tunnel aperture and least at the tunnel exit. There was an inverse correlation between graft-tunnel motion and healing in the femoral tunnel [52]. Biomechanical studies have shown that aperture fixation reduces anteroposterior translation compared with suspensory fixation in BPTB grafts [29] and in HT grafts [61]. However, a study of HT and BPTB ACL reconstructions in cadavers found no significant difference in graft-tunnel motion between aperture and suspensory fixation [11].
Clinical studies have not shown an advantage of aperture fixation over suspensory fixation and no correlation between tunnel widening and functional outcome. A prospective study comparing HT and BPTB ACL reconstructions showed that despite increased tunnel widening in the HT cohort compared to the BPTB cohort, there was no significant difference in functional outcomes [14]. In a level 2 randomized controlled trial, Lubowitz and colleagues compared aperture fixation of the femoral and tibial tunnels with a cannulated retrograde screw to suspensory fixation with cortical buttons in all-inside allograft ACL reconstruction. At 2 years follow-up, there was no difference in knee stability, functional outcome scores or radiographic analysis for tunnel widening [38]. A meta-analysis of stability after ACL reconstruction as a function of fixation type concluded that there is no stability advantage of aperture fixation compared with femoral suspensory fixation when using second-generation tibial fixation devices [50]. Based upon the currently available literature, the location of graft fixation has no correlation with clinical outcome (Figs. 26.2 and 26.3).



Fig. 26.2
Classification of ACL graft fixation by location

Fig. 26.3
Location of ACL graft fixation does not affect functional outcomes
26.5.2 Interference Screws
Interference screws achieve aperture intratunnel fixation by stabilizing the graft close to the joint line. Interference screws have a long history of clinical success for both BPTB and HT autograft fixation [48]. One concern with interference screws for soft tissue grafts is that compressing the graft may affect its mechanical properties and biologic incorporation. In a sheep model of ACL reconstruction with autograft Achilles tendon fixed with biodegradable screws, the site of failure of all the grafts at 6 and 9 weeks postoperatively was the screw insertion site [65].
The ultimate load to failure of interference screws has been reported in several studies and ranges between 390 N [42] and 790 N [31]. However, many different parameters may influence fixation by interference screws, including the length and diameter of the screw, the position of the screw, its divergence, the size of the gap between the graft and tunnel diameter, the relative difference between tunnel size relative to screw size, corticocancellous versus cancellous fixation, and the torque of insertion of the screw.
26.5.2.1 Length of the Screw
The significance of the length of the screw in interference fixation is uncertain. In a study comparing the strength of endoscopically inserted interference screws for BPTB ACL reconstruction, there was no significant difference between 20 and 25 mm screw lengths [10]. Black and colleagues showed no significant differences in displacement, load to failure and stiffness between 12.5, 15, and 20 mm long interference screws of the same diameter for BPTB fixation in porcine tibias [8].
For soft tissue fixation, Harvey and colleagues showed a trend toward better fixation with longer metal interference screws [24]. Another study found similar results with bioabsorbable screws [64]. However, Stadelmaier and colleagues showed that there is no difference in strength between the screws of different lengths when the screw was advanced, so the tip of the screw was flush with the tibial plateau [58].
26.5.2.2 Diameter of the Screw
Increasing the diameter of the screw increases the fixation strength for both BPTB and HT ACL reconstruction. Kohn and Rose compared 7 and 9 mm metal interference screws in tibial and femoral tunnels for a 10 mm BPTB plug and showed superior fixation of the 9 mm screw on both sides [31]. The diameter of the screw may be more important for BPTB fixation strength when there is a larger gap between the bone block and tunnel wall. In a study of porcine tibias, no advantage was conferred by using a 9 mm screw compared with a 7 mm screw with gap sizes of 1–2 mm. When the gap was 3–4 mm, however, the 9 mm screw gave superior fixation [12].
For HT ACL reconstruction, Weiler et al. showed that tendons placed in tunnels of the same diameter and fixed by bioabsorbable screws of equal size had a lower strength of fixation than when fixed with screws oversized by 1 mm [64]. The downside to using an interference screw which is excessively large, however, is that it may lacerate the graft during screw insertion.
26.5.2.3 Position of the Screw
Central placement of an interference screw between the four limbs of a hamstring graft increases the area of bone-tendon contact available for healing in the tunnel [25]. A study of human cadaver knees conferred no advantage over eccentric placement, regarding load to failure and slippage at time zero, but did result in superior stiffness [54]. An experiment performed in a polyurethane foam model, however, showed no compromise in fixation with central compared with eccentric placement of the screw [55].
26.5.2.4 Divergence of the Screw
Angular deviation of the interference screw from the bone tunnel may occur during screw insertion, particularly on the femoral side. Divergent angles over 20° have been shown to reduce the strength of fixation in BPTB ACL reconstruction [25]. Screw divergence may be reduced by using a two-incision method using cannulated screws or by inserting the femoral screw through the same portal used to ream the femoral tunnel [25]. When using cannulated screws, the guidewire itself may diverge from the tunnel into the cancellous bone, a problem that may be corrected by using larger diameter guidewires. It is important to note, however, that no clinical studies have shown a correlation between screw divergence and laxity or clinical outcome [25].
26.5.2.5 Corticocancellous Versus Cancellous Fixation
Since cortical bone is stronger than cancellous bone, the pullout strength of the graft construct is increased by achieving corticocancellous fixation. In a study of calf bone, fixation with cancellous-only interference screw fixation demonstrated significantly lower load to failure and more slippage compared to corticocancellous fixation under cyclic loading [24].
26.5.2.6 Insertion Torque
26.5.2.7 Bioabsorbable Versus Metal Interference Screws
Metal interference screws promote early integration into bone with high initial fixation strength and a higher load to failure than bioabsorbable screws in biomechanical studies [45]. Metal interference screws have afforded positive clinical outcomes and low complication rates and prevented excessive laxity. Drawbacks to using metal interference screws include complicated hardware removal during revision surgery and the creation of artifact on postoperative MRI [41].
Bioabsorbable interference screws, on the other hand, dissolve after 2–3 years, simplifying revision surgery, and do not cause artifact of postoperative MRI. However, complications have been reported with bioabsorbable screws, including breakage during surgery, bone tunnel widening, intra-articular screw migration, and foreign body reactions [41].
Several randomized controlled trials have been performed comparing metal and bioabsorbable interference screws. A recent systematic review of overlapping meta-analyses found similar clinical and functional outcomes between metal and bioabsorbable screws for BPTB and HT grafts. However, prolonged knee effusion, femoral tunnel widening, and screw breakage were more common with using bioabsorbable screws [41].
26.5.3 Screw and Sheath Devices
Combination screw and sheath devices have been designed for HT grafts to separate the grafts, secure concentric placement of the screw, and provide homogeneous friction between the tendon and the bone [1]. Biomechanical testing of these devices has shown varying results. A porcine study of six hamstring tendon graft fixation devices showed the screw and sheath device to have the highest load to failure (1,332 N) and lowest residual displacement [32]. In contrast, another biomechanical study of five different tibial fixation devices in a calf model showed the screw and sheath device to have the second lowest load to failure at 543 N [16]. In a bovine study, Smith and colleagues compared the radial force and pullout strength of two interference screws with two screw and sheath devices and found superior performance of screw and sheath devices for both measures [57]. Another biomechanical study in a porcine model comparing three interference screws and five screw and sheath devices showed no significant differences for ultimate failure load and cyclic displacement between the two fixation types, although the highest ultimate failure loads and least amount of cyclic displacement were observed for combination devices [1].
Clinical studies of screw and sheath devices are limited. One prospective clinical study comparing screw and sheath fixation, bioabsorbable interference screws, and cross-pins randomized into four groups showed no statistically or clinically relevant difference between the groups at 2 years of follow-up [23].
26.5.4 Cross-Pins
Cross-pin fixation achieves intratunnel fixation using biodegradable pins which pass through the substance of the graft. Cross-pins have been used for both BPTB and HT grafts on both the tibial and the femoral side and have the advantage of simplifying revision ACL reconstruction. Over 50 % of cross-pins may break; however, this has not been shown to affect clinical outcomes [3].
The load-to-failure of cross-pin fixation was 868 N and 994 N in two different biomechanical studies using porcine knees [31, 42]. Zantop and colleagues compared cross-pins with bioabsorbable interference screws in an ovine model of ACL reconstruction with an autograft Achilles tendon. At 6 weeks, the stiffness of the cross-pin group had improved by 52 % compared with the interference screw group, which decreased by 67 %. The strength of the interference screw constructs decreased by 81 % at 6 weeks, while the cross-screw group deteriorated only 48 % [68]. A biomechanical study of four different femoral soft tissue fixation devices in a porcine model, however, showed increased slippage of cross-pins and interference screws compared with cortical buttons and transfixation devices [2].
A small number of clinical studies have shown equivalent results using cross-pins compared to other ACL graft fixation devices. In a prospective, randomized trial of double-bundle ACL reconstruction with hamstring autograft, cross-pins were compared with cortical buttons. At a mean follow-up of 30 months, there was no statistically significant difference in subjective or objective outcomes, except for the KT-1,000 value, which was 1.30 mm in the cross-pin group versus 1.95 in the cortical button group [28]. Three studies have compared cross-pins with bioabsorbable interference screws and found no significant differences at 1, 2 and 5 years after surgery [18, 60, 63]. Bjorkman and colleagues showed no difference at 5 year follow-up between cross-pin fixation and metal interference screws in clinical or radiographic outcomes [7].
26.5.5 Transfemoral Suspensory Fixation Devices
Soft-tissue grafts may be fixed within the femoral tunnel by wrapping the grafts 180° around a metal or bioabsorbable transfixation pin. Bioabsorbable pins have the advantage over metal pins for revision ACL reconstruction; however, they also may break in 20 % of patients, can migrate out of the bone leading to iliotibial band irritation, and may cause femoral tunnel widening [13]. Complications have been reported during graft passage and fixation using this device [35].
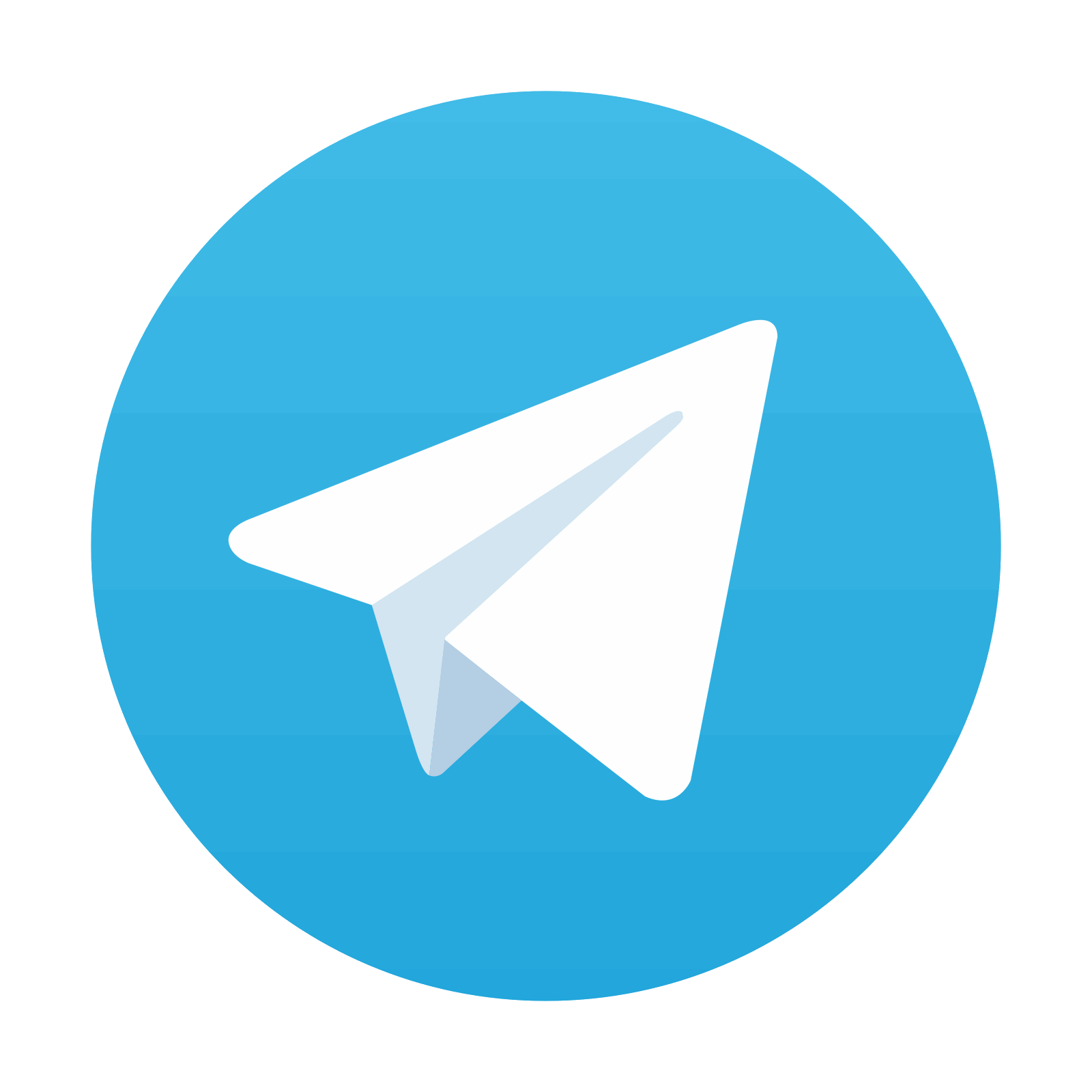
Stay updated, free articles. Join our Telegram channel

Full access? Get Clinical Tree
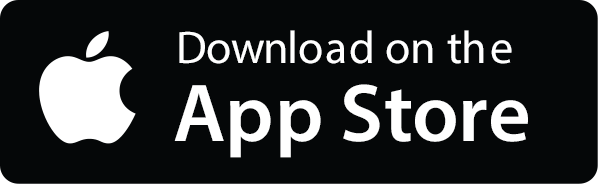
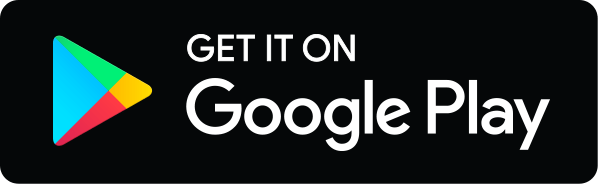