Pulsed electromagnetic fields in the treatment of nonunions—the Dutch experience
Introduction
Nonunion of long bones is a challenge for orthopaedic and trauma surgeons. The exact cause of a nonunion is unknown and various factors contribute to the development of a non-union. Many surgical treatment options have been published for the treatment of nonunions, including pulsed electromagnetic fields (PEMF). In this chapter the authors will provide valid clinical evidence of the effectiveness of PEMF stimulation in the treatment of nonunions of bone fractures gained in the Netherlands.
History and development
The first successful use of electrical stimulation in the treatment of delayed unions of bones was reported in the 19th century. For unclear reasons this treatment was neglected until the 1950s. The first successful use of electrostimulation was reported by Fukada et al in 1957 [1]. They demonstrated that mechanical loading of bone produced electrical potentials in bone tissue. Bassett et al (1962) [2] and Friedenberg et al (1966) [3] demonstrated that osteogenesis could be modulated by bioelectric potentials. Bassett et al [2] implanted iridium electrodes on dogs’ femurs and showed extensive callus formation around the cathode after a small direct current was induced.
A first successful clinical application of electrostimulation on a patient with nonunion of the medial malleolus was reported by Friedenberg et al in 1971 [4].
In the 1970s and 1980s there was little acceptance of PEMF treatment in delayed unions and nonunions. Some of the reasons were little research, no inclusion and exclusion criteria, the devices were 220 voltages with big coils, and the treatment was a clinical one. During the 1990s invasive, semi-invasive, and noninvasive techniques were developed. Research was done and there were several observational studies that demonstrated the positive effect of PEMF treatment in the treatment of delayed and nonunion with success rates of up to 90% [5–11]. To the authors’ knowledge four double-blind randomized control studies of electrical or electromagnetic stimulations versus placebo have been reported [12–15].
Gossling et al (1992) [16] published a literature review from 1977 to 1987, in which the results of the conservatively treated tibial nonunions treated with electrical stimulations were compared with the operatively treated tibial nonunions. Their conclusion was that, given the cost of potential morbidity after surgery, PEMF treatment of ununited fractures should be considered as an effective alternative.
Biological and biophysical aspects associated with the treatment of bone fractures and nonunions by means of electromagnetic induction
Various methods have been developed to introduce extremely low levels of electrical and electromagnetic fields into the fracture site using invasive, semi-invasive, and noninvasive electrical signaling techniques. These include: direct current (DC), capacitive coupling (CC), pulsed electromagnetic fields (PEMF), and combined magnetic fields (CMF). PEMF and CMF are also referred to as inductive coupling (IC).
Modern bone-growth stimulators are predominantly based on IC. These utilize single or dual coil configurations, placed at or around the fracture site, in order to set up time-varying magnetic fields at the fracture site. The resulting induced time-varying electrical fields are comparable to the mechanically generated electrical potentials.
Bone formation and bone healing process
Before looking at the effects of IC, a review is given of the bone healing and bone formation process.
There are three stages of bone healing:
Inflammatory stage
An initial inflammatory stage gives rise to the development of a hematoma. Inflammatory cells and fibroblasts in the bone contribute to the formation of granulation tissue, vascular tissue invasion, and migration of mesenchymal stem cells. The exposed (porous) cancellous bone provides a scaffold for this early process to take place.
Repair stage
Mesenchymal stem cells differentiate into osteogenic precursor cells. Further differentiation and mitosis result in metabolically active bone-forming chondrocytes and osteo-blasts. As vascular invasion progresses, secretion of osteoid from the osteoblasts results in an organic extracellular collagen matrix being laid down, which subsequently mineralizes to form a soft callus around the fracture site. The callus ultimately ossifies to form bridging woven bone across the fracture gap.
Remodeling stage
Finally, bone remodeling takes place over a period of months, in which the bone achieves its original strength, structure, and shape. This adaptation process is regulated by mechanical stresses exerted on the bone during this period.
The role of growth factors
Bone formation and healing is regulated by various biochemical, biomechanical, cellular, hormonal, and pathological factors. The process can be inhibited by systemic factors such as smoking, diet, diabetes, and osteoporosis.
Bone-cell metabolism is constantly moderated by a range of hormones, local factors, and protein growth factors. In particular, parathyroid hormone (PTH), vitamin D, and calcitonin are the hormones having the greatest influence on the metabolism.
Growth factors and growth-factor receptors are secreted from osteoblasts and chondrocytes. Their main role in the bone formation/repair process is to stimulate cell growth, proliferation, and differentiation. The important growth factors associated with osteogenesis are:
Growth factors belonging to the transforming growth factor beta (TGF-ß) super family including TGF-ß1 and the bone morphogenetic proteins (BMPs) BMP-2, BMP-3, BMP-4, and BMP-7
lnsulin-like growth factor (lGF)
Platelet-derived growth factor (PDGF)
Fibroblast growth factor (FGF)
Vascular endothelial growth factor (VEGF)
TGF-ß1 controls cell proliferation and cell differentiation. The concentration of TGF-ß1 growth factor acts as a marker for the proliferation of osteoblasts. TGF-ß1 and BMPs can stimulate osteochondroprogenitor cells to differentiate into chondrocytes.
Specific BMPs can induce mesenchymal stem cells to differentiate into osteogenic precursor cells, which have the potential to undergo mitosis and differentiate into osteo-blasts.
Insulin-like growth factors, lGF-ll, and lGF-ll-binding proteins are secreted from osteoblasts. lGF-ll is the most abundant growth factor in bone and is the only one that can be used to bring about proliferation (cell growth) of cultured osteoblasts. Proliferation of the osteoblasts results from the attachment of lGFll to the binding proteins or receptors on the cell membrane surface. The resulting cascade of cell proliferation leads to the production of the extracellular matrix.
More than 35 years of clinical experience backed by controlled clinical studies, have shown that noninvasive, IC stimulation is a safe alternative and/or adjunctive treatment for delayed and nonunion fractures. The efficacy of this method is approximately 75–80%, which is comparable to the results of primary nonunion surgery.
Mechanism of action of electromagnetically induced electrical fields
Particularly during the last 15 years, researchers have performed numerous in vitro and in vivo well-designed controlled cellular, animal, and human studies in order to establish the effects of electromagnetic induction on bone formation and repair. The research into the mechanism of action has mainly been based on studying the influence of biophysical stimuli (electrical/electromagnetic signals) on the human bone cell line and in particular on mesenchymal stem cells, osteoblasts, osteoclasts, and chondrocytes [17]. In addition, the effects of electromagnetic induction on explanted callus, at various stages of growth, and nonunion fracture tissue have been researched.
Table 1.3.1-1 gives an overview of the adopted study model and the resulting effects of applying IC stimulation [18].
In general, these studies demonstrate that IC can:
Upregulate TGF-ß1 protein synthesis and mRNA expression [18–20].
Stimulate the secretion of growth factors (TGF-ß1, BMPs, lGF-ll, and IGF-IIBP) from osteoblasts [18, 20–24].
Upregulate mRNA expression [18].
Modulate Ca2+ binding to calmodulin [20, 25, 26].
Increase the production and secretion of prostaglandin E2 (PGE2). This results in an increase in bone remodeling or formation and bone resorption.
IC stimulation results in an increase in the proliferation of osteoblast cells mediated by the upregulation of growth factors [27]. IC can also enhance the production of growth factors that are upregulated following fracture trauma.
Brighton et al [25] suggest that the signal transduction biochemical pathways involved in osteoblast proliferation are as follows: time-varying magnetic fields probably penetrate the osteoblast cell membrane to set up an induced time-varying electrical field within the cytosol. This in turn causes the release of intracellular calcium ions, which leads to an increase in activated cytoskeletal calmodulin and subsequently to cell proliferation. Activated calmodulin is known to promote nucleotide synthesis and cellular proliferation. This pathway has also been shown to increase the level of TGF-ß mRNA in osteoblasts [20].
Extrapolation of the results from cellular and animal studies to the in vivo environment may be questionable as many more upregulating and downregulating signals are involved. However, animal studies and human clinical studies as well as the extensive clinical use of IC have established the enhanced healing effects.
Relating these findings to the bone-healing process, it becomes evident that the inductively coupled electrical fields have an enhancing effect on the bone formation and fracture healing process at different stages of healing.
During the early stages of repair of bone formation and the inflammatory stage of the healing process, growth factors play a crucial role in skeletal development and bone healing. They are capable of inducing mesenchymal stem cells to differentiate into bone cells. It has been shown that inductively coupled electrical fields may enhance the signaling effect of BMP2 and BMP4 on mesenchymal cells resulting in differentiation into bone cells [23, 28, 29]. From this point of view it would be beneficial to apply IC stimulation at the fresh fracture stage. It is also an argument for applying IC stimulation as an adjunct to bone-graft surgery particularly when allograft bone and nonosteoinductive bone substitutes have been used. The upregulation of BMP signaling is also a reason to apply IC in order to enhance bone fusion as adjunct to spinal fusion surgery.
Later on, in the repair stage of healing, IC results in an increase in growth factor secretion from osteoblasts and chondrocytes. The resulting upregulation of TGF-ß1, lGF-ll protein synthesis, and mRNA expression results in osteoblast proliferation, increased synthesis of extracellular matrix protein and gene expression, as well as the enhancement of chondrogenesis and endochondral calcification. As can be seen by IC stimulation of explanted fracture callus cultures, which results in an increased level of lGF-ll [20].
For delayed union and nonunion fractures, it has been shown that cells derived from human tissue from both hypertrophic and atrophic nonunions react to IC by an increase of TGF-ß1-released and subsequent cell proliferation [30]. The widespread clinical application of IC at this stage has been extensively studied and its efficacy substantiated.
Reference has been made above to IC as the means of electromagnetic stimulation. Studies have shown that the degree to which growth factors and binding proteins are upregulated, depends on the signal waveform, pulse and burst frequencies, amplitude as well as the duration (dose) of stimulation. Systems with wave forms were shown to be physiologically effective typically generate peak time-varying magnetic fields of 0.1–20 Gauss, inducing peak electric fields of 1–150 mV/cm at the target site. Stimulation time for PEMF vary from 3 hours per day to 24 hours continuously. Stimulation time for combined magnetic fields (CMF) is 30 minutes per day.
Brighton et al′s study [25] into the signal transduction pathway found that osteoblast-like cell cultures when exposed to PEMF and CMF did not show an unlimited increase in DNA level with time. DNA increase stopped after 30 minutes of exposure. This suggests that the increase in DNA using these methods of stimulation could be limited by the amount of Ca2+ available in the intracellular calcium stores.
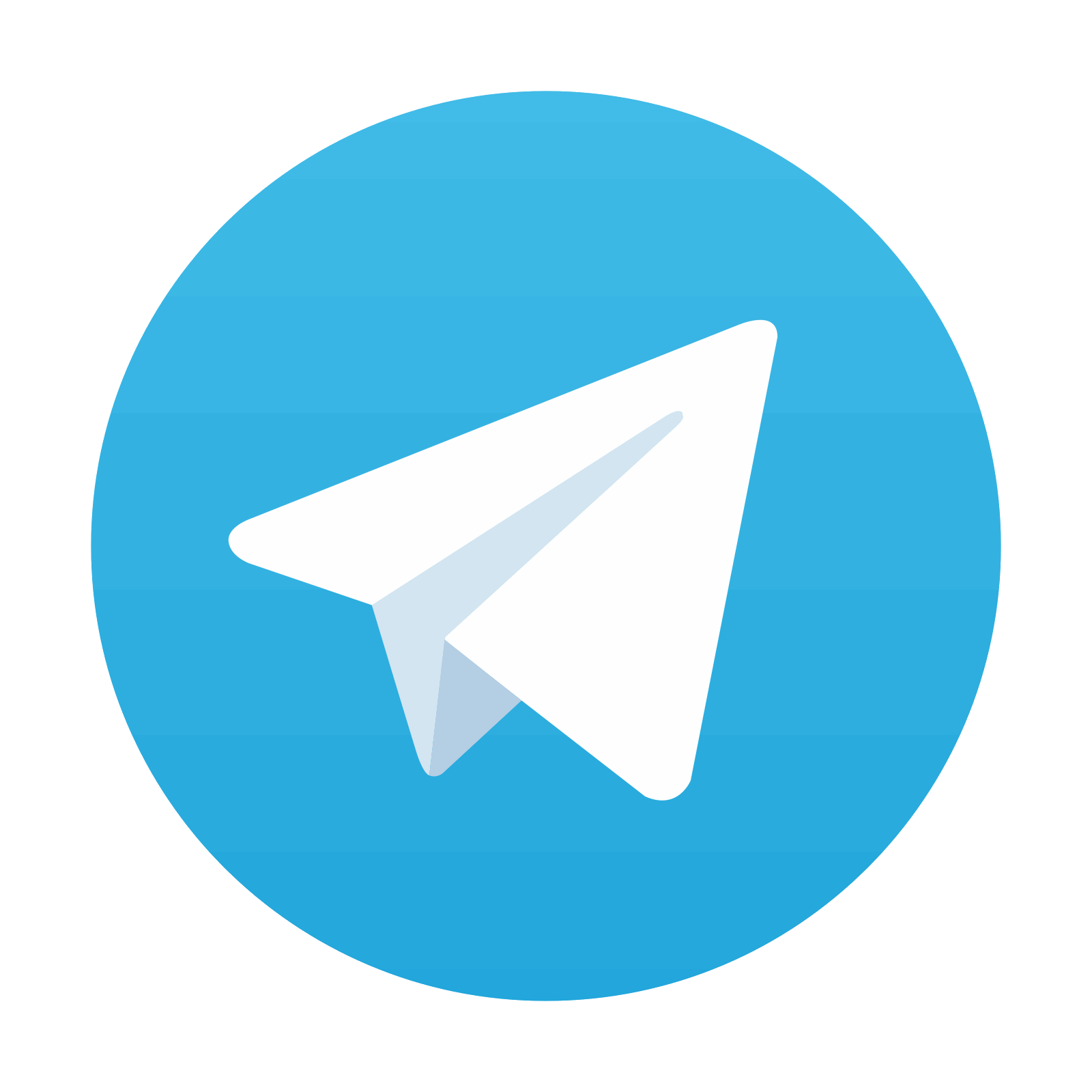
Stay updated, free articles. Join our Telegram channel

Full access? Get Clinical Tree
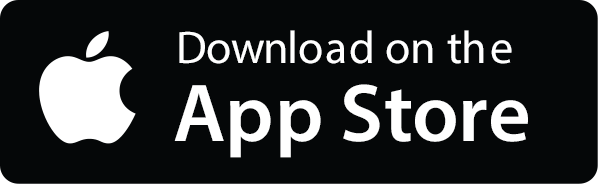
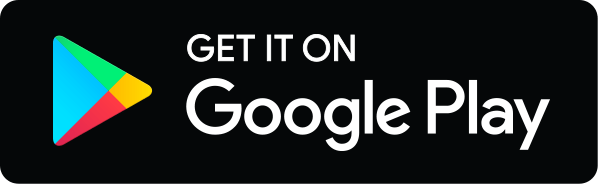