CHAPTER SYNOPSIS:
This chapter focuses on the issues surrounding and implications of primary total ankle replacements. It will also give the reader background information about the history and historical aspects of ankle replacements. Furthermore, it will highlight the currently available ankle replacement systems and point out the differences and similarities between the systems. In essence, this chapter will attempt the impossible—to bring together all the design elements of total ankle arthroplasty (TAA), to classify the current designs as either fixed or mobile bearing, and then to categorize, as much as is feasible, the various design features of all the ankle replacements. The classification will include tibial component design, including fixation into the tibia and talar replacement design and whether medial or lateral removal of bone is necessary from either the tibia or the talus for insertion. This chapter also discusses talar component fixation and the shape and mechanism of stabilizing the polyethylene component.
IMPORTANT POINTS:
- 1
Anatomic considerations—The ankle is not a hinge joint.
- 2
Ankle design considerations—Anatomically designed TAAs are better than nonanatomic ankles.
- 3
Whether fixed or mobile bearing polyethylene inserts are superior remains an unsolved question.
- 4
The instrumentation for inserting TAAs is critical to their survival.
- 5
Ligament balancing in TAA is important.
- 6
Cobalt chrome and titanium are the materials of choice in TAA.
- 7
The surgeon’s learning curve in replacement of the ankle joint is significantly long and flat.
Indications for Total Ankle Arthroplasty
- 1
Primary degenerative joint disease (DJD) of the ankle
- 2
Posttraumatic DJD of the ankle
- 3
Severe DJD of the ankle secondary to inflammatory naturopathies, e.g., rheumatoid arthritis
- 4
Ankle joint arthritis in combination with subtalar and midfoot arthritis
Contraindications for Total Ankle Arthroplasty
- 1
Current or recent ankle joint infection
- 2
Age—younger than 50 in nonrheumatoid patients
- 3
Diabetic patients without pulses
- 4
Peripheral neuropathy
- 5
Ligamentous instability with varus or valgus of greater than 20 degrees
- 6
Loss of motor function around the ankle
CLINICAL PEARLS:
- 1
Preoperative range of motion determines postoperative range of motion.
- 2
Never leave the operating room with uncorrected ligament balance issues.
- 3
Correct leg alignment problems before or at the time of the TAA.
- 4
Always remember the foot! A stable, plantigrade foot is paramount to support the TAA.
HISTORY/INTRODUCTION/SCOPE OF THE PROBLEM
The cause of ankle arthritis is primarily trauma. In a review of 390 patients, the diagnoses were posttraumatic ankle osteoarthritis in 78%, secondary arthritis in 13%, and primary osteoarthritis in 9%. Hence, ankle arthritis has no doubt been present since the beginning of humankind. With increasing sports activity among youth and increased motor vehicle accidents, the incidence is not likely to decrease. And despite continuing innovations in fracture care, the damage to the tibiotalar articular surface often cannot recover despite the best and most precise internal fixation. The standard of care for a long time has been ankle fusion. However, there are limitations of ankle fusion; these include loss of range of motion, a long healing period after fusion, and later arthritis in adjacent joints. Yet, something must be done for these people with disabling pain. In a Canadian study, it was found that patients with end-stage ankle arthritis had as severe, if not worse, physical and mental disability than patients with end-stage hip disease. As with hip and knee arthritis, it is reasonable to conclude that patients with end-stage ankle arthritis will clearly benefit from the design innovations of total ankle replacements (TARs) discussed in this chapter.
The ideal patient for total ankle arthroplasty (TAA) is a lightweight individual with a body mass index between 19 and 25 kg/m 2 , no deformity (i.e., varus or valgus <10 degrees), and low demand who has severe pain secondary to loss of cartilage in the ankle and has retained at least 70% of his or her normal range of motion. Patients with previous subtalar or talonavicular fusion or severe arthritis in these joints are also considered good candidates because pantalar fusions would be their only other recourse. Of course, this represents only one of seven patients. The other patients have relative contraindications, including high activity, body mass index greater than 25 kg/m 2 , varus or valgus greater than 15 degrees, partial areas of avascular bone, traumatic bone loss, osteoporosis, poor motion, diabetic patients with pulses, or patients with a remote history of infection. Patients who should not undergo this procedure include patients with a Charçot deformity, diabetic patients with no pulses, patients with dropfoot, patients with current infections or skin ulcers near their ankle, and patients with clubfoot, because their deformities are just too difficult to manage with a prosthesis.
CURRENT KNOWLEDGE
The author of this chapter was able to find 21 ankle replacement systems in use or in late stages of development at the time this chapter was written, as follows:
- 1
Agility (DePuy, Warsaw, IN) ( Fig. 10-1 )
FIGURE 10-1
Agility including custom Agility with stem.
(Courtesy of DePuy, Warsaw, IN.)
- 2
Ceramic, polyethylene-lined TNK (Kyocera Corp, Kyoto, Japan)
- 3
Scandinavian Total Ankle Replacement (S.T.A.R.® Ankle), (Small Bone Innovations, Inc., Morrisville, PA) ( Fig. 10-2 )
FIGURE 10-2
S.T.A.R.® Ankle
(Courtesy Small Bone Innovations, Inc., Morrisville, PA.)
- 4
Hintegra (New Deal SA, Vienne, France) ( Fig. 10-3 )
FIGURE 10-3
Hintegra.
(Courtesy of New Deal SA, Vienne, France.)
- 5
Salto (Tornier S.A.S., Saint Ismier, France) ( Fig. 10-4 )
FIGURE 10-4
Salto.
(Courtesy of Tornier S.A.S., Saint Ismier, France.)
- 6
Buechel Pappas (Endotec, South Orange, NJ)
- 7
Mobility (DePuy, Warsaw, IN) ( Fig. 10-5 )
FIGURE 10-5
Mobility.
(Courtesy of DePuy, Warsaw, IN.)
- 8
BOX (Finsbury, Leatherhead, Surrey, UK)
- 9
ESKA (ESKA Implants GmbH & Co., Lubeck, Germany)
- 10
AES (Biomet, Dordrecht, the Netherlands)
- 11
Zenith (Corin, Cirencester, England)
- 12
Albatross (Groupe Lepine, Lyon, France)
- 13
Ramses (Fournitures Hospitaliers, Mulhouse, France)
- 14
CCI Evolution (van Straten Medical/Argomedical, Doets, Germany)
- 15
German Ankle System (R-Innovation, Coburg, Germany)
- 16
Alphamed Ankle Brace (Alphamed Medizintechnik Fischer GmbH, Lassnitzhöhe, Austria)
- 17
INBONE (INBONE Orthopaedics, Boulder, CO) ( Fig. 10-6 )
FIGURE 10-6
INBONE.
(Courtesy of INBONE Orthopaedics, Boulder, CO.)
- 18
Salto-Talaris (Tornier, Stafford, TX) ( Fig. 10-7 )
FIGURE 10-7
Salto-Talaris in situ.
(Courtesy of Tornier, Stafford, TX.)
- 19
Eclipse (Kinetikos Medical Inc., Carlsbad, CA; recently acquired by Integra Life Sciences Holding Corporation, Plainsboro, NJ)
- 20
TARIC (Implantcast GmbH, Buxtehude, Germany)
- 21
Zimmer Total Ankle (Zimmer Corp., Warsaw, IN)
The design of TARs has been a confluence of intuition, experience, and engineering. All too often the former has taken precedence over the latter. Part of the problem is that although the ankle joint may seem like a hinge, it is not in a line perpendicular to the tibia. The axis of rotation does not remain constant during range of motion, despite a relative congruency of this joint. Allowing for rotational forces must be accomplished, while maintaining the stability of the joint and its components. From a practical standpoint, understanding this fact and creating an anatomic ankle replacement by combining the best design features of all ankle replacements would seem desirable so that even the occasional-use surgeon would find the prosthesis easy to use. In that way, surgeons could approach the current published success rate of 5-year survival of 86% without first having to operate with 30 prostheses. While the details of each of the ankle designs discussed in this chapter are too extensive to be covered in one chapter, the reader is referred to two articles that describe the design of several ankles in detail.
Of the 21 ankle prostheses 6 have fixed polyethylene (PE) components and 15 have non-fixed or mobile-bearing PE components. There is reference in the literature to first- and second-generation prostheses, as well as third generation. For design purposes we are only going to consider two generations. The first-generation prosthesis is a cemented prosthesis; some are anatomic like the early Townley device, which captured the talus only, and others like the Mayo ankle, which attempted to mimic the convex and concave surfaces of the normal ankle. The second-generation devices are those listed in the Summary and include all the current two- and three-piece designs and even one resurfacing component.
While the mobile-bearing design seems quite appropriate to avoid plasticware, which was common in the early two-piece design, in reality, over time, heterotopic bone typically forms around the mobile-bearing, restraining its motion. However, that study just looked at anteroposterior (AP) movement. Another study compared the translational and rotational motion occurring between the components of the Salto implant, which revealed that the rotational motion played a more dominant role in the kinematics of the prosthesis. In the Salto-Talaris ankle, the instrumentation is designed to allow the tibial component to find its correct rotation before being drilled into its permanent position. Theoretically, this allows the PE to be placed in the least constrained position before its position is made permanent. In reality, the medial lateral distance is so small and the prosthesis is so wide, there is very little, if any, motion, especially with the smaller prostheses. No head-to-head study of fixed versus mobile bearing has been completed to date, although there is a U.S. Food and Drug Administration–sponsored study under way that compares the Mobility device (mobile bearing) with the Agility device (fixed bearing).
However, the use of the mobile bearings is not without unique problems such as fracture, edge loading, and impingement. In a metaanalysis of 1318 TARs in Sweden, 56 (4.2%) complications were reported with the mobile bearing. A total of 39 cases were revised, of which 20.7% were revised due to failure of the mobile bearing. Medial impingement is also seen with the mobile-bearing design. This occurs when the talar component glides medially with no constraint from the tibial component. Placing the tibial component in varus accentuates the problem. However, many of the complications related to mobile bearing are not inherent to the design. Instead they are related to incorrect indication for TAA, incorrect soft tissue balancing, incorrect positioning of components or implantation in ankles with hindfoot malalignment, and ankle instability.
The good news in the design of TARs is that the weight-bearing load across the normal ankle and an anatomically designed TAA, despite the small difference in internal work at the ankle, showed no significant difference in mechanical loading. And while long-term clinical results will not be forthcoming for the newer prostheses for many years, the increasing use of wear simulators will enable us to project the design that is most favorable for long-term success. For example, in one study using an industrial robot that enables complex motion under predefined conditions (RX 90; Stäubli, Bayreuth, Germany) the force, torque, and motion differences between the specimens before and after implantation of the prostheses were lower with the German Ankle System than with the Hintegra (unpaired t -test, each P ≤ .05). Yet, even with that kind of instrumentation, it will be important as well to consider the knee’s involvement with TAR.
Although there is much more to be learned regarding TARs, it is known that a nonanatomic design (Agility) leads to excessive peak pressures in normal patient activity that may exceed recommended contact pressures (10 MPa) and the compressive yield point (13 to 22 MPa) for PE. This is consistent with the clinical follow-up of this design radiographically, which commonly reveals periprosthetic lucency, lysis, and component migration or subsidence.
One of the intangibles that are impossible to evaluate unless you have the opportunity to work with many different designs is the instrumentation designed for insertion. You could have the most suitable ankle design available, but if the instrumentation causes you to put the TAA in malalignment, it will not work well. However, it does not seem to make a difference thus far in design on the incidence of some of the intraoperative complications like malleolar fracture. Those kinds of complications are typically related to user experience.
Whether the alignment jig is fixed to the tibia proximally and distally and whether fluoroscopy is used to confirm positioning can make a big difference in alignment. Then, too, there is intuitive reason to believe that computer-assisted navigation for ankle replacement insertion will be as valuable for TAA as it is for total knees. However, getting manufacturers interested in that kind of technology has been slower to come about, probably because of the lower number done on TAA versus TKAs and hence the lower potential market value of developing such a system.
Initial fixation also seems to be important. Keeping the prosthesis from moving allows bony ongrowth. Although there are other conditions to be considered, this factor was brought out in one study that showed an unfavorable patient score at 6 months when there was inadequate fixation of the components at the time of the initial surgery. And no matter how good the design may be, tibial component displacement from the neutral position can lead to imbalance. In one study, implantation with the Agility created atypical ligament length change in one or more of the periankle ligaments. In particular, anterior/posterior displacement of the components significantly changed the lengthening behavior of all four tested ligaments; the anterior talofibular ligament was sensitive to transverse plane displacements, whereas the tibiocalcaneal ligament was sensitive to coronal plane displacements.
Finally, critical in the design of an ankle replacement is the size of the prosthesis. Only recently have detailed studies been done that suggest how large the prosthesis should be to cover the bone. Fortunately, it appears that bones at the ankle joint have similar shapes; that is, the three dimensions are in direct proportion to each other. Yet, this same study also noted that not one of the internal dimensions correlated with the only one possible external measurement—the malleolar width. We also know that tibial coverage of the talus is 88.1 degrees (SD = 6.7 degrees), the tibial tilt is 83 degrees (SD = 3.6 degrees), the width of the distal tibia is 33.6 mm (SD = 3.6 mm), the radius of the ankle joint is 18.6 mm (SD = 2.3 mm) with an anterior offset of the center of rotation of 1.7 mm (SD = 2.1 mm), and the height of the talus is 28.2 mm (SD = 4.0 mm). This kind of information helps manufacturers decide how large and how small to make the largest and smallest components available and in what dimension they should increase in size.
Currently, no ankle replacements mimic the articular geometry at the bearing surface interfaces or match the native bony bed with the implant’s external dimensions. A new design might be helped by high-resolution three-dimensional computed tomography images. These measurements include the width of the superior talar dome surface (measured at the anterior, middle, and posterior portions) and the arc radius of the talar dome. The results yielded an average anterior width of 29.9 ± 2.6 mm, a middle width of 27.9 ± 3.0 mm, and a posterior width of 25.2 ± 3.7 mm. The talar dome radius was 20.7 ± 2.6 mm. The width linearly decreased from anterior to posterior ( P < .001). A significant gender difference was found in both the width and the radius ( P values < .05), except at the middle width ( P = .07). Yet, the intuitive sense that creating an anatomic prosthesis would be the best design is then confounded in sophisticated studies employing a doubly concave meniscal bearing in the BOX ankle. Here, the designers demonstrate significant motion of the mobile-bearing PE; 5.6 mm posteriorly during the simulated stance and corresponding anteroposterior displacement of the talar component of 8.3 mm. The predicted pattern and the amount (10.6 degrees) of internal-external rotation of the ankle complex were found to be in good agreement with corresponding in vivo measurements on normal ankles. A peak contact pressure of 16.8 MPa was observed, with majority of contact pressures below 10 MPa. For most ligaments, reaction forces remained within corresponding physiologic ranges. Perhaps as long as the bone surfaces are covered with physiologic anatomic components, the meniscal bearing may be allowed to be different.
Also seen in the BOX ankle is an attempt to establish a more natural relationship between the implanted components and the retained ankle ligaments. According to the BOX designer’s research, physiologic ankle mobility is reproduced best with a design featuring a spherical convex tibial component, a talar component with radius of curvature in the sagittal plane longer than that of the natural talus, and a fully conforming meniscal component. Their preliminary observations in trial implantation and in a few patients suggest that while reproducing physiologic ankle mobility, the new design is capable of maintaining complete congruence at the two articulating surfaces of the meniscal bearing over the entire motion arc, with the prospect of minimizing wear of the PE. Also, a working model two-dimensional tool was used to simulate the mechanics of the intact and replaced ankle joints. Based on previously validated mathematical models, elementary objects were used to model ligaments, articular surfaces, retinaculum, and muscle–tendon units. The performance of several pairs of prosthetic articular surfaces was also analyzed.
According to the results of these simulations, rolling as well as sliding motion occurs in the natural ankle, governed by a ligamentous linkage. Elongation of the tibiocalcaneal and calcaneofibular ligaments was found to be 1.5% and 4.8%, respectively. A 13% change in lever arm length occurred for both the tibialis anterior and gastrocnemius muscles during ankle flexion. Unlike the currently available three-component designs, the BOX TAA with its convex-tibial ligament–compatible prosthesis was found to be able to restore the original mobility and physiologic function of the ligaments. This prosthesis combined freedom from restraint with congruity of the components throughout the range of flexion.
In addition, when developing the BOX TAA, a computer-assisted planning tool was used to assess the geometry of the articular surfaces and of main ligaments, defined from digitization on scanned lateral radiographs of the joint. From the expected movement arc and from component dimensions, a mechanical model of the ligaments was used to select the optimal size and the position of the BOX ankle replacement. The planning was based on the minimization of ligament change in length and of bone stock to be removed. The three-component prosthesis allows complete congruence over the entire range of flexion. A convex shape for the tibial arc was preferred because of the better degree of entrapment of the meniscal bearing. A 5-cm convex–tibia arc radius gave 2-mm entrapment together with 9.8 mm of tibial bone cut. Ligament elongation imposed by full congruence of the articular surfaces was less than 0.03% of the original length. It seems clear that anatomically shaped prostheses are here to stay. Finally, design considerations that seem to have little, if any, merit at the present time include diagnoses and gender.
All the prostheses that articulate with the PE are cobalt chrome. Those components that hold the PE can be made of titanium, cobalt chrome, or, in one case, ceramic (TNK). The textured surfaces of the prosthesis are covered with a titanium spray or have a Spongiosa-type surface (ESKA). No textured surface has yet to be proved superior to another. Hydroxyapatite coating of one prosthesis, the STAR, did not seem to make a difference in the clinical result. It appears that the titanium spray is sufficient.
Surgical Approach
The patients are placed supine on the operating table with a sandbag underneath the trocanter to keep the leg from excessive external rotation. The patient’s heels are at the end of the bed. The operating room lights are arranged in a linear row perpendicular to the patient just above the surgeon’s head to allow visualization into the depth of the ankle. A C-arm fluoroscopic unit is conveniently and sterilely draped to aid in ensuring that the cutting guides are in the proper position. I prefer the monitor to be on the opposite side of the patient with the C-arm on the affected side. Most of the total ankle surgery performed uses an approach the ankle through a 4-inch anterior skin incision that extends down to the interval between the tibialis anterior and the extensor hallicus longus. Care is taken to avoid cutting the retinaculum over the tibialis anterior to avoid excessive pressure from this tendon on the retinacular repair at the time of closure. Beware of the superficial peroneal nerve. The periosteal tissue is then peeled off the tibia and the talus. Deep retractors, once thought to be deleterious, are used immediately to avoid repetitive skin traction from assistants. Repetitive skin retraction leads to vascular channel blockers, which causes wound skin necrosis. Once the jigs are in place, the bone is cut and removed. Great care is taken to avoid osteotomes which can fracture malleoli. Instead, a small reciprocating saw and a small oscillating saw, as well as a larger oscillating saw, are available to cut bone instead of splitting it. The ankles are all inserted without cement. In the end with the prosthesis inserted, if the ankle cannot be dorsiflexed to 5 degrees with the prosthesis in place, and it is deemed undesirable to remove additional distal tibial bone, a gastrocnemius recession or a percutaneous triple hemisection of the Achilles tendon is performed. The wound is closed in layers with absorbable suture beginning with the capsule, then the retinaculum, next the subcutaneous tissue, and finally the skin, where I prefer 3-0 nylon suture to staples. A gentle compressive dressing is applied at surgery together with a nontight fiberglass cast. Patients are discharged the following day from the hospital, ideally with a popliteal block catheter that they may remove at home when the anesthetic fluid has run out. Once home, patients are instructed postoperatively to keep their “toes above their nose, get up once an hour during the day (to change positions and avoid blood clots) and not to put weight on the foot.” At 3 weeks, the cast is removed, the stitches are taken out, and patients are placed in a removable boot with light weight-bearing and early range of motion. At 6 weeks, patients may be full weight-bearing in the removable boot and thereafter advance to shoes with an elastic compression hose to avoid swelling. Formal physical therapy is not ordered routinely.
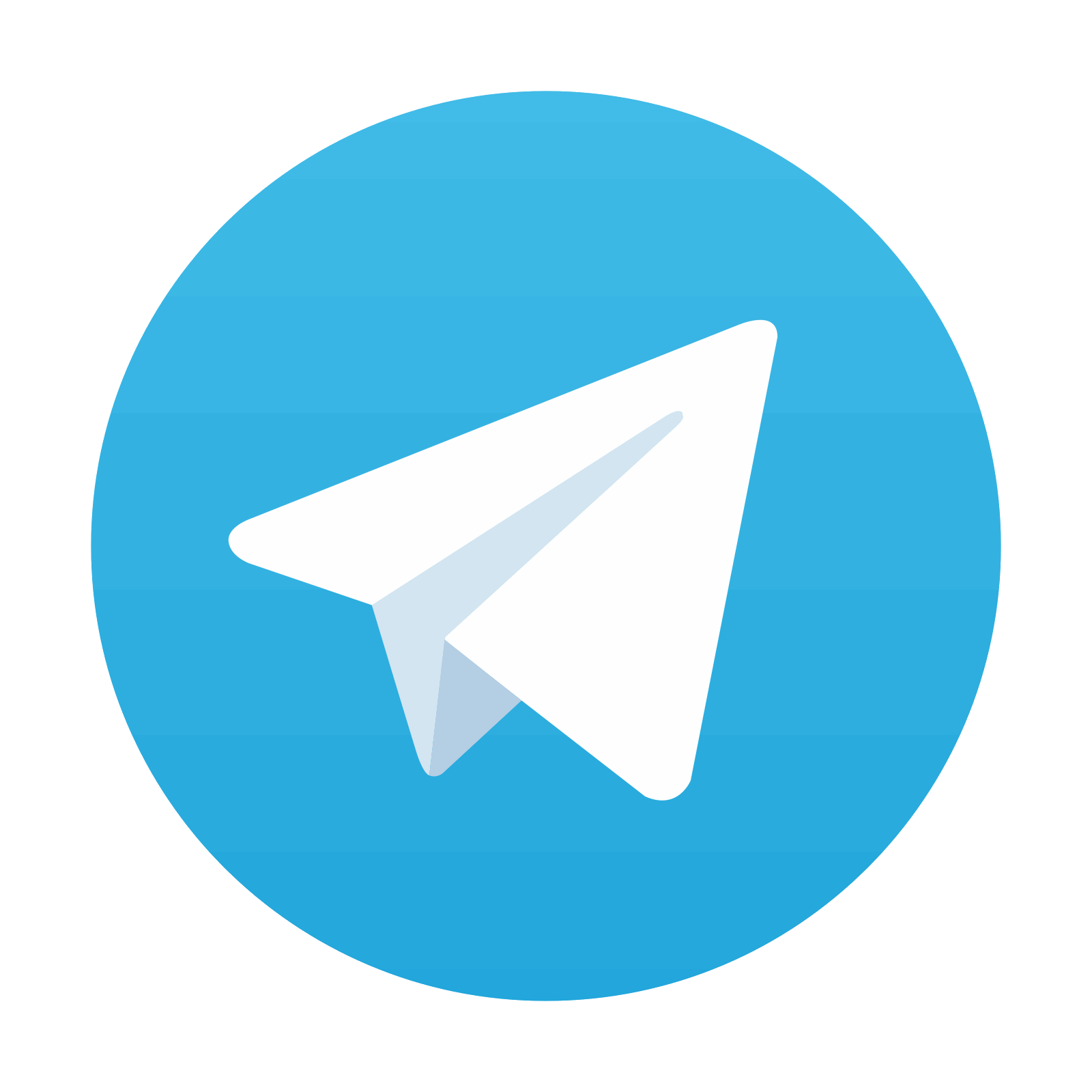
Stay updated, free articles. Join our Telegram channel

Full access? Get Clinical Tree
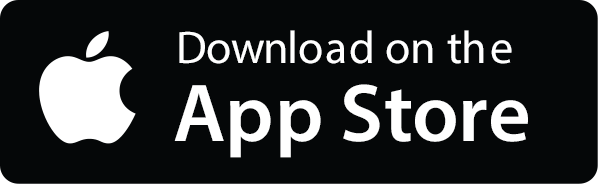
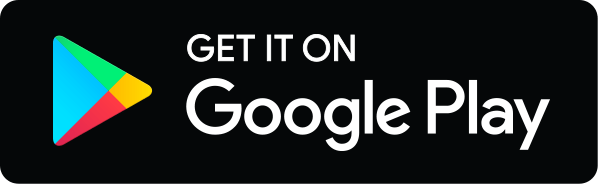