© Springer Japan 2015
Kazuyuki Kanosue, Tetsuya Ogawa, Mako Fukano and Toru Fukubayashi (eds.)Sports Injuries and Prevention10.1007/978-4-431-55318-2_1414. Prevention of Anterior Cruciate Ligament (ACL) Injury
(1)
The Micheli Center for Sports Injury Prevention, Waltham, MA, USA
(2)
Division of Sports Medicine, Boston Children’s Hospital, Boston, MA, USA
(3)
Cincinnati Children’s Hospital Medical Center, Cincinnati, OH, USA
(4)
Sports Medicine Biodynamics Center and Human Performance Laboratory, Cincinnati, OH, USA
(5)
Department of Pediatrics, College of Medicine, University of Cincinnati, Cincinnati, OH, USA
(6)
Athletic Training Division, School of Allied Medical Professions, The Ohio State University, Columbus, OH, USA
Abstract
Along with the increase in number of female athletic participants, the number of resultant athletic injuries among female athletes has also rapidly increased. Anterior cruciate ligament (ACL) injury is more prevalent in physically active females as compared to their male counterparts in the sports of basketball and soccer. ACL injury was classified into three categorizations based on three common pathomechanics, and the relative distribution of ACL injury was analyzed between sexes. The most common mechanism of ACL injury was noncontact in nature, and such noncontact ACL injury was more prevalent in female athletes as compared to male athletes. Because a majority of ACL injuries occur in noncontact mechanism, especially in female athletes, a few clinical trials were conducted to examine the effectiveness of preventive neuromuscular training on ACL injury reduction in the female population. Recent meta-analytic reports have confirmed significant ACL injury reduction by female athletes who performed preventive neuromuscular training compared to those who did not or only maintained their routine warm-up. Numerous evidence supports the proposition that specific exercises incorporated into preventive neuromuscular training protocols lead to favorable biomechanical alteration in dynamic movements, which likely contribute to ACL injury reduction in female athletes. Future directions of ACL injury research include the development of an effective screening system in order to identify at-risk athletes. Also, a strategy to implement preventive neuromuscular training programs and to enhance compliance and adherence needs to be developed.
Keywords
ACL PreventionACL pathomechanicsACL prevention clinical trialsNeuromuscular trainingClinical feasibility14.1 ACL Injuries – Why We Need to Prevent Them
14.1.1 Historical Background
Since inception of Title IX of The Educational Assistance Act in 1972, the number of female athletes at the high school level has increased more than 11 fold (from 0.3 to 3.2 million); whereas male athlete participation has shown a much smaller increase from 3.7 to 4.5 million in the United States (National Federation of State High School Association 2012). Along with the increase in number of female athletic participants, the number of resultant athletic injuries among female athletes has also rapidly increased, and exposed potential sex specific risk factors.
Among many types of debilitating injuries, female athletes have shown particular susceptibility to knee joint injury, especially anterior cruciate ligament (ACL) injury. In high school basketball, it was reported that one in every 81 female athletes sustained an ACL injury (Messina et al. 1999; Gomez et al. 1996) and the total number of ACL injuries among female athletes was estimated to be approximately 38,000 annually in the United States as of 2001. In recent reports, the estimated number of ACL injuries ranged from 80,000 to 350,000 annually in the US (Cimino et al. 2010; Wojtys and Brower 2010). Epidemiological studies have demonstrated that during landing and cutting/pivoting sports, ACL injury occurs at a 4–6 fold greater incidence in females when compared to males in the sports of basketball and soccer (Arendt and Dick 1995).
14.1.2 Physical Consequences of ACL Injury
The ACL plays an integral role in knee joint stability. When the ACL is ruptured, an individual often experiences various functional difficulties including inability to decelerate, cut and pivot, along with the presence of pain and effusion in the knee joint. (Cimino et al. 2010) Because of these functional difficulties, most individuals with an ACL injury are forced to limit their physical activity level, which may lead to restricted participation in athletics. At the collegiate level, ACL injury results in the greatest loss of time from athletic participation when compared to ankle and traumatic head injuries (Hootman et al. 2007). In addition, once an athlete sustains a primary ACL injury, they may have up to a 24–29 % increased risk for a subsequent ACL injury if they return back to the sport. (Webster et al. 2014)
In order to restore knee joint stability and functionality, most patients choose to have ACL reconstruction surgery. However, ACL reconstruction surgery is not a solution that resolves all deficits or prevents long term morbidity. After ACL reconstruction surgery, return to play rate is approximately 65 % (McCullough et al. 2012), and female athletes are even less likely to return to previous athletic activities (Ardern et al. 2011). One study found 12 % of ACL reconstructed individuals experienced a subsequent ACL injury on their ipsilateral (reconstructed/involved) or contralateral (uninvolved) ACL within 5 years (Salmon et al. 2005). Another study also reported 24 % of ACL reconstructed high school age athletes who had an ACL reconstruction experienced a subsequent ACL injury within 1 year from return to play (Paterno et al. 2010). In short, the low rate of return to play and high risk for the second ACL injury are major concerns.
Over the long term, a high prevalence of pre-mature osteoarthritis (OA) has been reported 10–15 years after ACL reconstruction surgery (Oiestad et al. 2010, 2011). Several studies concluded that 50 % of those who had an ACL reconstruction surgery were likely to develop OA within 10 years (Maffulli et al. 2010; Lohmander et al. 2007). In addition, a longitudinal study that followed female soccer players with a reconstructed ACL reported a negative knee-related quality of life along with the OA development (Lohmander et al. 2004). The mean age of this cohort was 31 (range between 26 and 40) years old (Lohmander et al. 2004). At this point, no effective OA treatment has been introduced.
14.2 Common ACL Injury Pathomechanics and the Relative Distribution
14.2.1 Introduction
Because of the sudden impact and continuous negative health consequences of ACL injury, especially for the female population, a number of individuals and research teams have made a commitment to understanding the mechanism underlying the occurrence of ACL injuries. From their reports, the basis for several common ACL injury mechanisms have emerged. Identifying the mechanism of ACL injury is important because it provides a pathway in the development of an effective preventive intervention strategy. Hence, this chapter focuses on identifying common pathomechanics of ACL injury as well as providing distribution of the ACL injury based on the pathomechanics.
14.2.2 Pathomechanics Classifications
At a recent ACL research retreat (Research Retreat 2008) agreement was reached on a pathomechanic definition introduced by Hewett and colleagues (2007a). According to this definition, ACL injuries can be classified into three different schemes based on the type of contact mechanisms: Type I: Direct contact (an external force was directly applied to the injured knee and which is probably the proximate cause of injury), Type II: Indirect contact (an external force was applied to the athlete but not directly to the injured knee. The force is involved in the injury process but is probably not the proximate cause), and Type III: Noncontact (forces applied to the knee at the time of injury result from the athlete’s own movements and do not involve contact with another athlete or object). Previously published studies that investigated the pathomechanics of ACL injuries have typically utilized the above classification schemes (Arendt and Dick 1995; Agel et al. 2005; Boden et al. 2000; Myklebust et al. 1997; Walden et al. 2011). However, they have not made a distinction between direct and indirect contact ACL injuries (Arendt and Dick 1995; Agel et al. 2005; Boden et al. 2000; Myklebust et al. 1997; Walden et al. 2011). Rather, the two mechanisms (direct and indirect mechanisms) were combined and expressed as a contact ACL injury (Arendt and Dick 1995; Agel et al. 2005; Boden et al. 2000; Myklebust et al. 1997; Walden et al. 2011). In research focused on injury prevention, it is critical to differentiate between contact and noncontact mechanisms in order to adopt the most effective design strategy.
14.2.3 Direct Contact Mechanism
A contact ACL injury is defined as an external force application to the injured knee, which is likely to be the proximate cause of injury (Hewett et al. 2007a). Several studies reported that external force application is likely to come from other players rather than balls, goals, or floors (Agel et al. 2007; Dick et al. 2007). A recent descriptive laboratory study reported that an external force that induces knee abduction and anterior tibial translation increases ACL strain by 4.6 fold (Quatman et al. 2011). Also, anterior tibial translation with internal and external rotations increases ACL strain by 3.9 fold and 3.7 fold, respectively (Quatman et al. 2011). Therefore, direct external force applications to the knee joint, which cause knee abduction, and anterior tibial translation with internal and external rotations, likely elevate the risk of tearing the ACL.
14.2.4 Indirect Contact Mechanism
In an indirect injury mechanism, an external force is applied to body parts besides the knee joint and results in an ACL tear. For instance, an external force applied to the trunk can create a loss of control over the trunk, which may generate an excessive ground reaction force (GRF) because of the asymmetrical dynamic motions. Since the produced GRF runs laterally approximately to the center of the femoral head, an external hip abductor torque is created. In order to compensate for the torque, the hip adductor needs to be activated, which is likely needed to bring the knee joint into a more abducted or valgus position (Hewett and Myer 2011).
Another potential indirect contact ACL injury mechanism involves a contact force on the thigh. During cutting, pivoting, and landing activities, if external force is applied to the lateral side of the thigh with the foot fixed, the knee is forced toward an abducted direction, which can result in an ACL tear. Similarly, if the external force comes from the anterior side of the thigh, the knee is forced into hyperextention. A hyperextended knee with a combination of anterior tibial translation and rotation (Quatman et al. 2011) is likely to cause a strain on the ACL, which may result in an ACL tear.
14.2.5 Noncontact Mechanism
Numerous studies have sought the underlying mechanism and risk factors for noncontact ACL injuries. Using a prospective cohort study design, Hewett and colleagues discovered several biomechanical differences between high school female athletes who suffered noncontact ACL injuries and those who did not (Hewett et al. 2005). The observed biomechanical risk factors were: elevated knee abduction moment, limited knee flexion, excessive GRF, and an asymmetrical landing pattern in the drop phase of a vertical jump. In addition to the knee kinematic differences, recent studies reported an influence of trunk neuromuscular control on knee injury (Hewett et al. 2009; Zazulak et al. 2007). A 3 year prospective study that initially aimed to find a trunk neuromuscular deficit involving low back pain found a link between trunk control and ACL injury (Zazulak et al. 2007). The study reported that the inability to control lateral trunk displacement accurately predicted future ACL injury. With a logistic regression model, trunk displacement, proprioception, and history of low back pain indicate future ACL injury risk with 91 % accuracy in female athletes, but not male athletes. Additionally, using National Basketball Association (NBA) and Women’s National Basketball Association (WNBA) players, a video analysis study reported that lateral trunk flexion and knee abduction angles were greater in cutting and landing tasks in female players who suffered ACL injuries as compared to male and control female players (Hewett et al. 2009).
Available evidence indicates that ACL rupture is a resultant product of kinetic (neuromuscular) and kinematic (biomechanical) events that occur during dynamic human movements. The following provides a potential explanation of the underlying anatomical structures and how they sequentially interact in the production of a noncontact ACL injury: upper body strength differences exist between males and females (Miyaguchi and Demura 2009; Evans et al. 2007). Because of limited upper body strength, females may present more lateral displacement of the trunk in cutting, pivoting, and landing activities. When the trunk is flexed laterally without sufficient control during dynamic movements, weight can be shifted to one leg. If one leg contacts the ground before the other leg, the GRF tends to be higher than when both legs make contact at the same time, since only one leg is available to absorb the GRF. The GRF, as the result of asymmetrical movements, runs approximately lateral to the center of the femoral head, which produces external hip abductor torque. The Newton’s third law states: For every action, there is an equal and opposite reaction. Thus, in order to balance the external hip abduction torque created by the lateral GRF, the hip adductor is activated. The activated hip adductor musculature brings the thigh an inward direction and potentially contributes to hip adduction and internal rotation of the femur. The increased hip adduction angles create increased knee abduction angles in weight-bearing activities (Ford et al. 2006). As a result, the ACL is strained and occasionally torn if the external knee abduction moment is beyond the ACL’s yielding point.
14.2.6 Distribution of ACL Injuries Based on a Pathomechanical Classifications
Arendt and Dick reviewed 5 years of epidemiological data from the NCAA men’s and women’s soccer and basketball athletes and found that the most frequently observed ACL injury mechanism was noncontact (Arendt and Dick 1995). They concluded that 68.7 % of all ACL injuries were noncontact in nature based, on an analysis of the injury mechanisms. However, definite sex differences exist within the mechanisms. Although 55.4 % of all ACL injuries were noncontact in nature in male athletes, 74.5 % of such ACL injuries occurred in female athletes. More specifically, the data can be organized by sex and sports. In the sport of soccer, the number of contact and noncontact ACL injuries were about the same in males (34 contact vs. 32 noncontact ACL injuries), but females demonstrated a 1.7 times higher noncontact mechanisms as compared to contact mechanisms (31 contact vs. 53 noncontact ACL injuries). Similarly, in basketball, males had 1.9 times higher noncontact mechanisms relative to contact mechanism (16 contact vs. 30 noncontact ACL injuries), whereas females suffered ACL injuries involving noncontact mechanism at 4 times the rate of ACL injuries involving contact (34 contact vs. 137 noncontact ACL injuries).
Another study that analyzed the NCAA men’s and women’s soccer and basketball epidemiological data for 13 years (1990–2002) generally supported the above findings (Agel et al. 2005). This study reported 65.3 % of all the ACL injuries involved the noncontact mechanism. Comparing the mechanisms based on the sexes, 56.9 % of male soccer and basketball athletes suffered noncontact ACL injuries, and 68.4 % of the female athletes who competed in the same sports experienced noncontact ACL injuries. When the data was analyzed by sex and sports, the results were very similar to the above study done by Arendt and Dick. Male soccer players suffered slightly more contact than noncontact ACL injuries (72 contact vs. 66 noncontact ACL injuries). On the other hand, female soccer players had a 1.4 times higher noncontact ACL injury rate as compared to contact ACL injuries (115 contact vs. 161 noncontact ACL injuries). In the sport of basketball, male basketball players suffered noncontact ACL injuries 2.1 times higher than contact ACL injuries (37 contact vs. 78 noncontact ACL injuries). However, female basketball players suffered noncontact ACL injuries at a rate 3.1 times higher than contact mechanism (100 contact vs. 305 noncontact ACL injuries). Comparing noncontact ACL injury rates solely based on the sex, a combination of college female soccer and basketball athletes suffered ACL injury incidents 2.7 times higher than their male counterparts (253 total ACL injuries from male soccer and basketball athletes vs. 681 total ACL injuries from female soccer and basketball athletes).
Other studies have reported comparable findings. A prospective study which analyzed elite European male and female soccer players documented that 62 % of all the observed ACL injuries were noncontact in nature (Walden et al. 2011). A study examining the type of ACL injury mechanisms in women’s college basketball games reported that 64 % of all ACL injuries mechanisms occurred in noncontact situations (Agel et al. 2007). Another report in which retrospective interviews were conducted with ACL injured individuals noted that 72 % of their injuries involved no contact (Boden et al. 2000). For male and female European handball players, a study analyzed the number of ACL injuries based on the player’s position (Agel et al. 2007). According to the study, 84 % of all ACL injuries come from back and wing position players and 95 % of those ACL injuries were noncontact in nature.
14.2.7 Summary of Common ACL Injury Pathomechanics and the Relative Distribution
ACL pathomechanics are classified into three schemes: direct, indirect, and noncontact mechanisms. Based on NCAA epidemiological data and other sources, 60–70 % of ACL injuries involve a noncontact mechanism. When there is no contact, ACL is likely to be strained or torn by a resultant force created by human movements, which can be triggered by a loss of trunk control. The loss of trunk control, especially lateral flexion, can lead to asymmetrical movement patterns, such as sudden single leg landing or cutting, which cause an excessive GRF. Lower extremity muscles, in particular, hip musculature, attempt compensating the excessive GRF. However, if the force is not well attenuated, ACL bundles can be strained or torn. For the indirect mechanism, an external force application to body parts other than the knee joint may trigger the whole cascade of events similar to those described above and result in a torn ACL. External force application to a knee joint directly, which promotes knee abduction and anterior tibial translation with internal rotations, is also likely to cause a direct contact ACL injury.
Females are 10–20 % more susceptible to noncontact ACL injuries than their male counterparts. Because the studies we reviewed lumped direct and indirect mechanisms together as contact ACL injuries, it was difficult to estimate the rate of direct and indirect ACL injuries separately. Nevertheless, noncontact situations appeared to account for a majority of the ACL injuries. Since the noncontact situation does not involve a contact and the external forces were created within the individuals own movements, altering the causative forces by an intervention program may be possible. Future studies need to focus on what type of interventions would alter the dynamic movements in a beneficial manner.
14.3 ACL Injury Prevention Clinical Trials
14.3.1 Introduction
The available data confirmed that approximately 60–70 % of all the ACL injury are noncontact in nature (Arendt and Dick 1995; Agel et al. 2005; Boden et al. 2000; Myklebust et al. 1997; Walden et al. 2011). NCAA female soccer and basketball athletes demonstrated a 2.7 higher overall ACL injury incidence rate as compared to male athletes participating in the same sports (Agel et al. 2005). Additionally, epidemiological data indicated that the prevalence of noncontact ACL injury mechanism in female athletes is approximately 10–20 % higher than their male counterparts (Arendt and Dick 1995; Agel et al. 2005). From the evidence, it is abundantly clear that young female athletes are the most susceptible to noncontact ACL injuries.
In order to explain the higher ACL injury incidence in adolescent female athletes, numerous research studies have been conducted from anatomical, hormonal, neuromuscular/biomechanical, and recently genetic (Naraoka et al. 2012; Posthumus et al. 2009; Lo et al. 1998) viewpoints. However, to establish prevention protocol, the focus needs to be on identifying modifiable risk factor(s) instead of non-modifiable risk factor(s) (Hewett et al. 2007b). While some studies did report an association between ACL injury and anatomical, hormonal, and DNA risk factors (Posthumus et al. 2009), those risk factors are not modifiable. Currently, the only risk factor considered to be modifiable is neuromuscular/biomechanical component. Therefore, in this chapter, we review the literature which covers the utilization of neuromuscular training (NMT) as an intervention method to reduce ACL injury in young female athletes.
14.3.2 Literature Search Method
A literature search was performed using PubMed and EBSCO host (CINAHL, MEDLINE, and SPORTDiscus) with a date range from 1995 to 2011 on May 31, 2012. The key words search was performed with the following words: “knee”, “anterior cruciate ligament”, “ACL”, “prospective”, “neuromuscular”, “training”, “female”, and “prevention.” The language was limited to English, and subjects were all human. The following inclusion criteria were applied: (1) the number of ACL injury incidents were reported, (2) a NMT intervention aimed to reduce ACL incidence was applied, (3) a control group was used, (4) a prospective controlled trial study design was employed, and (5) females were included as subjects.
14.3.3 Documented Clinical Trials from 1995 to 2012
Hewett et al. (1999)
This research team used a prospective cluster study design and provided 6 weeks of neuromuscular training, consisting of weight training, plyometrics, and flexibility, to a total of 43 teams (volleyball, soccer and basketball) from area high schools. Each neuromuscular training session lasted from 60 to 90 min and took place three times per week for 6 weeks. Certified athletic trainers and physical therapists gave technique instructions and the training sessions progressed through three phases: (I) Technique phase, (II) Fundamental phase, and (III) Performance phase. The 15 girls’ teams that received the intervention (6 weeks of neuromuscular training) consisted of 366 athletes: 185 volleyball (50.5 %), 97 soccer (26.5 %), and 84 basketball (23.0 %) players. An ACL incidence rate of the intervention group was 0.06 per 1,000 h of Athletic-Exposure (1,000 h AE) in the intervention group and 0.11 per 1,000 h AE in the control group.
Soderman et al. (2000)
This prospective randomized controlled trial provided 10–15 min of balance training utilizing dynadiscs and balance boards to a total of 221 soccer players for 6 months. After randomization, 121 athletes (seven teams) were assigned to the intervention group and 100 athletes (six teams) were assigned to the control group. The athletes in the intervention group were asked to perform the balance training with balance boards every day for the 1st month. After the 1st month, training was decreased to 3 days per week. This study reported an ACL incidence rate of 0.68 per 1,000 h AE in the intervention group and 0.12 per 1,000 h AE in the control group.
Heidt et al. (2000)
This research group employed a 75 min long custom-made speed and agility program which was given to 42 randomly selected high school age soccer players for a total of 21 sessions (1st session was an orientation) over 7 weeks. The randomly selected subjects in the intervention group commuted to a local fitness gym to perform the intervention program in the pre-season. Over the course of 4 months, the ACL injury rate in the intervention group was 2.38, and 3.10 % in the control group. This study did not record or report exposure data.
Myklebust et al. (2003)
This study involved a 3 year prospective cross-over protocol (the 1st year was an observational year, with the two subsequent years involving intervention) The study subjects consisted of 1,705 female handball athletes playing for the top three Norwegian handball leagues. A 15 min session of balance exercises with mats and wobble boards was implemented 3 days per week in the initial 5–7 weeks, which was subsequently reduced to once a week for the remainder of the handball season (approximately 5 months). During the study period, the ACL incidence rates were: control (Year 1) 0.14 per 1,000 h AE, intervention (Year 2) 0.13 per 1,000 h AE, and intervention (Year 3) 0.09 per 1,000 h AE.
Mandelbaum et al. (2005)
Using a prospective cluster cohort study design, the research team applied a neuromuscular and proprioceptive program to a total of 1,885 female soccer players (1,041 subjects in the 1st year and 844 in the 2nd year) and compared the number of ACL injuries to age- and skill- matched controls. The program was 20 min in duration and consisted of education, basic warm-up, stretching for trunk and lower extremities, trunk and lower extremity strengthening and plyometrics. The exercises were performed at a pace of two to three sessions per week. This investigation reported an ACL incidence rate of 0.04 per 1,000 h AE in the intervention group and 0.24 per 1,000 h AE in the control group over two competitive soccer seasons.
Olsen et al. (2005)
Utilizing a cluster randomized controlled trial design, a 15–20 min long structured warm-up program was implemented to improve neuromuscular control, balance, knee and ankle strength, and running, cutting, and landing technique for Norwegian handball players (808 subjects in the intervention group). The structured warm-up program had four different exercises (warm-up, technique, balance, and strength and power), and each exercise progressed through increasing the levels of difficulty. The structured warm-up program was performed over 15 consecutive sessions and then once a week during the competitive Norwegian handball season. During the study period, the ACL incidence rate in the intervention group was 0.03 per 1,000 AE, whereas in the control group the incidence was 0.10 per 1,000 h AE.
Petersen et al. (2005)
This prospective cohort study incorporated 10 min of injury prevention training into a team warm-up. The injury prevention training program consisted of improving awareness of injury mechanisms and prevention strategies, balance-board exercises and jump training and was executed three times per week in the preseason and once a week during the competition period. Lower extremity injuries were tracked in the 134 female handball players who performed the training program and compared with age- and skill- matched controls. The study reported an ACL incidence rate 0.04 per 1,000 h AE in the intervention group and 0.21 per 1,000 AE in the control group.
Pfeiffer et al. (2006)
This research team implemented a 20 min plyometric-based exercise program twice a week for high school female soccer, volleyball, and basketball athletes over a 2 year period. The plyometric-based exercise program, “Knee Ligament Injury Prevention” (KLIP), was developed by various healthcare practitioners and experts. A total of 577 athletes (43 teams) were placed in the intervention group, and 862 athletes (69 teams) in the control group. During the investigation, the ACL incidence rate was 0.08 per 1,000 h AE in the intervention group and 0.04 per 1,000 AE in the control group.
Pasanen et al. (2008)
This cluster-randomized controlled study provided 20–30 min of NMT to top-level floorball athletes several times during the 6-month season. The NMT was designed to enhance the athletes’ motor skills and body-control awareness with sport-specific maneuvers that consisted of balance, body control, plyometrics, and strengthening. Players worked in pairs and were instructed to give each other feedback during the training. In total, 256 athletes (14 teams) maintained their training routine. The study reported an ACL incidence rate of 0.19 per 1,000 h AE in the intervention group and 0.16 per 1,000 h AE in the control group.
Steffen et al. (2008a)
Using a cluster-randomized controlled trial (113 teams, 2,100 players), this research team prescribed a 15 min structured warm-up program called “11”. The 11, which consisted of core stability, balance, plyometrics, and hamstring strengthening exercises, was applied to 1,073 young female soccer players (51 teams) for the first 15 consecutive sessions and once a week for the remaining seven and half months. The study documented an ACL incidence rate of 0.06 per 1,000 h AE in the intervention and 0.08 per 1,000 h AE in the control group.
Gilchrist et al. (2008)
In a randomized cluster controlled study, investigators applied the 20 min long program, previously reported by Mandelbaum et al. (Mandelbaum et al. 2005) to high level college female soccer teams. The intervention and control groups were paired and formed a cluster. The clustered pairs were purposefully allocated to different geographic regions throughout the US. Then, one cluster of each region was randomly selected for the study. Soccer players (583 players, 26 teams) classified in the intervention group performed the program three times per week for the entire fall soccer season (12 weeks). An ACL incidence rate in the intervention group was 0.20 per 1,000 h AE, whereas the ACL incidence rate in the control group was 0.34 per 1,000 h AE.
Kiani et al. (2010)
This prospective cluster control trial experiment (97 teams, 1,506 players) included a 20–25 min neuromuscular regimen consisting of a running warm-up, isometric contraction of lower extremity muscle groups, balance exercises with jump components, strengthening of lower extremities and core stability utilized by 777 young soccer players (48 teams) 2 days per week for the 2 month pre-season and once a week during 6 months of in-season sessions. This study reported an ACL incidence rate of 0 per 1,000 h AE in the intervention group and 0.08 per 1,000 h AE in the control group.
LaBella et al. (2011)
Using a randomized cluster controlled design, investigators applied a program called the “Knee Injury Prevention Program” (KIPP). A total of 737 athletes: 321 soccer (43.6 %), and 416 basketball (56.4 %) players practiced the KIPP, which was comprised 20 min of progressive strengthening, plyometric, balance, and agility exercises three times per week for one competitive season. Over the course of the study, an ACL incidence rate of 0.10 per 1,000 h AE was documented in the intervention group and 0.48 per 1,000 h AE was documented in the control group.
Walden et al. (2012)
This research team applied a cluster-randomized control design to a total of 4,564 young soccer players (230 teams) and intervened with a 15-min neuromuscular warm-up focused on the development of core stability, balance, and jump landing with proper knee alignment. Study therapists provided specific instruction for implementing the exercises to 1 coach and a player from each team before the initiation of intervention. Coaches were responsible primarily for instruction of exercise executions. A therapist made 2 unannounced visits to assist in the correction of training errors. A total of 2,479 young soccer players (121 teams) trained two times per week for the entire competitive season (7 months). During the course of the study, an ACL incidence rate of 0.05 per 1,000 h AE was documented in the intervention group and 0.11 per 1,000 h AE in the control group.
14.3.4 Summary of Prior ACL Injury Prevention Clinical Trials
A total of 14 clinical trials were reviewed. Recent meta-analytic studies utilizing these studies have demonstrated the effectiveness of neuromuscular training intervention for ACL injury reduction (Sadoghi et al. 2012; Gagnier et al. 2013). One study that incorporated all 14 clinical trials reported a significantly greater ACL injury reduction (56 %) in female athletes who performed preventive NMT compared with those who did not or continued traditional warm-up procedures (Myer et al. 2013). Although the prophylactic effectiveness of preventive NMT was identified, a large diversity existed in the preventive NMT programs including age, dosage, exercises, and sports. The aforementioned meta-analytic study pointed out influence of age on the prophylactic effectiveness (Myer et al. 2013). Future projects are warranted to find the most effective aspects within preventive NMT programs.
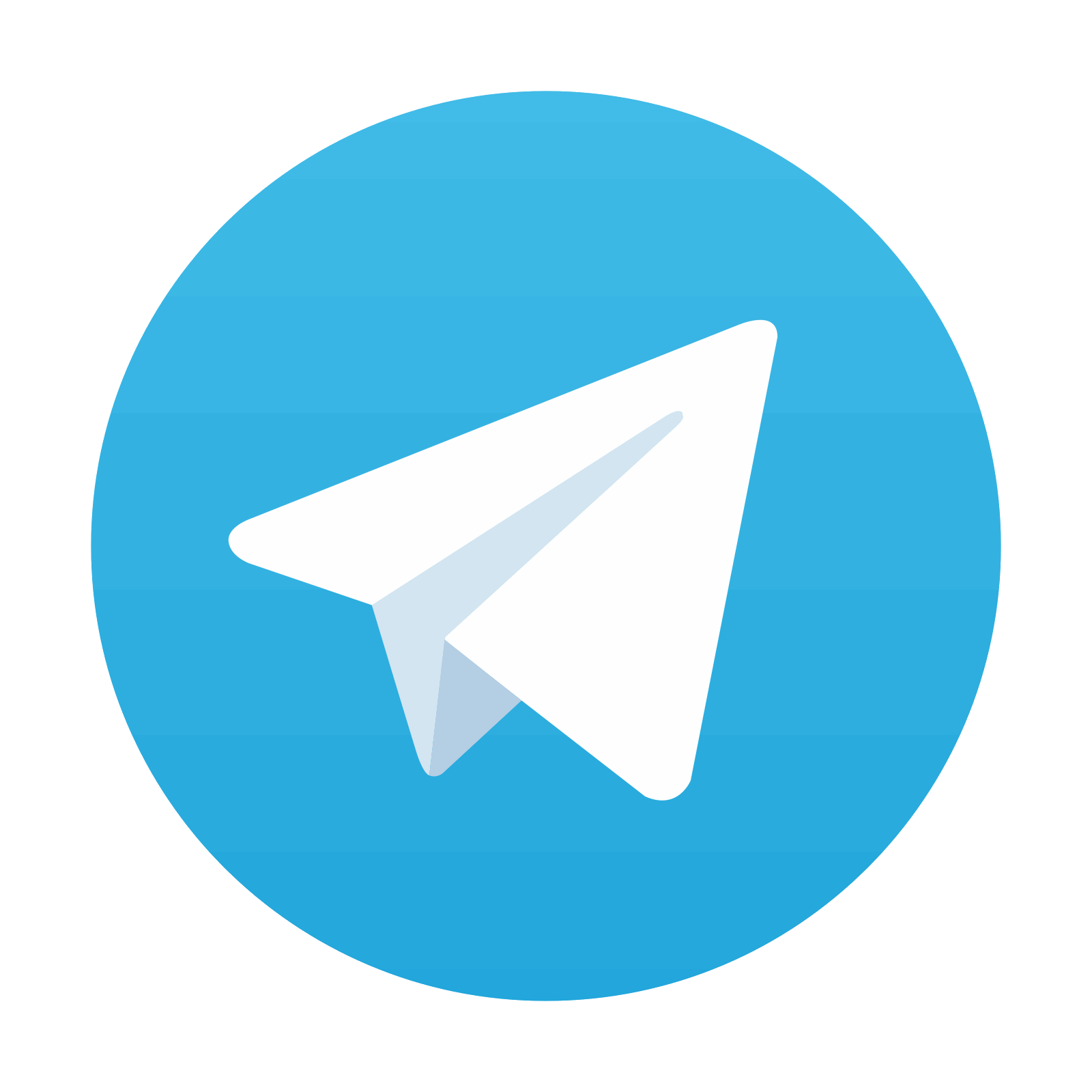
Stay updated, free articles. Join our Telegram channel

Full access? Get Clinical Tree
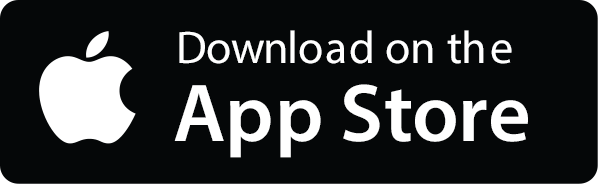
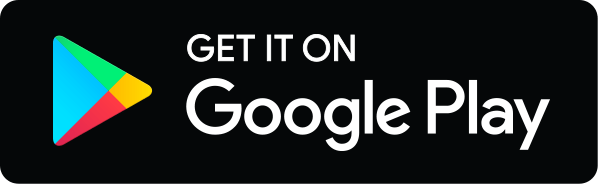