Electrodiagnostic studies
- 1
… are an extension of the clinical examination.
- 2
… are dynamic.
- 3
… can diagnose a brachial plexus injury.
- 4
… identify the location of injury: preganglionic or postganglionic; upper, middle, or lower trunk; supraclavicular or infraclavicular.
- 5
… comment on the severity of the injury.
- 6
… provide information regarding prognosis.
- 7
… identify evidence of early reinnervation before clinical improvement.
- 8
… aid in diagnosis of non-traumatic brachial plexus lesions.
- 9
… aid in treatment decisions.
- 10
… help in providing prognosis for recovery after surgical reconstruction.
Introduction
Electrodiagnostic (EDX) studies, such as nerve conduction studies (NCS) and electromyography (EMG), are readily obtainable evaluations that provide useful information regarding the function of peripheral nerves and muscles. These studies are extensions of the clinical assessment and must be guided by a thorough history and neuromuscular examination. EDX studies provide information regarding the location, severity, and prognosis of peripheral nerve lesions, thereby aiding the clinician in making appropriate decisions regarding additional diagnostic testing and further treatment recommendations.
EDX studies have been used to clinically evaluate peripheral nerve and muscle disorders for more than 60 years. Several different techniques have been described for assessing the neurophysiology of various peripheral nerves and muscle, and many advances in EDX testing resulted directly from improved technology and equipment. However, the basic principles of nerve and muscle physiology must continue to guide the use of modern technology; peripheral nerve and muscle fiber depolarizations generate electrical activity that can be recorded and measured. When stimulated by normal physiologic mechanisms or by externally applied stimuli (EDX testing), peripheral nerves depolarize and generate action potentials that travel along the course of the nerve. Therefore, nerve physiology can be assessed, recorded, and measured based on the type, location, and response of the particular nerve fibers stimulated (motor or sensory). Muscle fibers, both at rest and while contracting, also generate electrical activity that can also be observed and measured. Assessment of muscle-generated electrical activity provides valuable information, not only about the health and function of the particular muscle, but also about the innervating nerve.
Although many different EDX techniques are used to assess integrity and function of the various components of the peripheral nervous system, motor units, and muscle, this chapter will focus on NCS and needle EMG. These 2 techniques provide the most useful information when assessing lesions of the brachial plexus.
Electrodiagnostic examination – an extension of the clinical examination
When a patient has been referred for EDX testing to evaluate for a brachial plexus lesion, the physician performing the EDX examination must first complete a thorough history and perform a clinical neuromuscular examination of the patient. History and physical examination are crucial for developing a differential diagnosis to aid in planning and performing the appropriate EDX evaluation. The differential diagnosis will likely include the primary suspected etiology responsible for the patient’s symptoms, but it may also consider other potential diagnoses that reasonably account for the patient’s clinical picture. For example, a patient who presents with shoulder and arm weakness with sensory loss about the shoulder following a motorcycle accident accompanied by cervical spine trauma would be suspected of having sustained an upper trunk brachial plexus injury; however, the patient may also have more proximal C5 and/or C6 nerve root injuries or more distal injury to the suprascapular or axillary nerves. Some clinical findings may suggest a specific anatomic location for the site of injury; for example, the presence of a Horner’s sign (ptosis, meiosis, anhydrosis) implies a preganglionic nerve root injury. Therefore, EDX evaluation complements the physician’s clinical evaluation, and the astute EDX physician plans the patient’s study to evaluate for all possible diagnoses. EDX evaluation will attempt to confirm or reject diagnoses in the physician’s differential. The process is dynamic; as the study proceeds and information is acquired, the clinical neurophysiologist will add or eliminate diagnoses and adjust the remaining EDX evaluation accordingly. The EDX evaluation is complete only when the physician is able to confirm a diagnosis and eliminate other proposed etiologies from the patient’s clinical findings.
The timing of EDX testing and the type and distribution of EDX findings (both normal and abnormal) allows confirmation of a brachial plexus lesion, identifies its location within the plexus, determines its extent or severity, and comments on its prognosis and recovery. Upon completion of the study, the EDX physician prepares a report that includes (1) results of the various studies performed, (2) history and physical findings that aided in planning the study, and (3) a summary of EDX findings and an interpretation of these findings (the EDX diagnosis) for the referring physician. The referring physician can use these results to recommend further evaluation or treatment.
Timing of EDX studies
Most adult brachial plexus lesions are the result of trauma, and these injuries present with an acute alteration in motor and sensory function of the upper extremity. A brachial plexus injury can be strongly suspected following the patient’s initial evaluation in the emergency department. When should an EDX evaluation be done to further define and localize the lesion? Although it is technically possible to perform EDX studies soon after the patient has been injured, urgent EDX studies do not usually provide useful information. Time must elapse before EDX studies can yield a more complete and useful assessment of nerve function.
Three major classifications of nerve injury with increasing severity have been described: neurapraxia, axonotmesis, and neurotmesis. With any of these lesions, stimulating the nerve proximal to the site of injury yields no recordable response, thereby providing no information about the severity of injury. However, stimulating the peripheral nerve distal to a known site of injury can provide useful information. With neurapraxia, the nerve remains intact with anatomic continuity, but the injury alters nerve conduction; a conduction block occurs when the nerve action potential cannot pass the point of injury. When the cause of conduction block (swelling, contusion, etc.) resolves, the nerve will regain its ability to conduct with the return normal nerve function. Note that the nerve with a neurapraxic lesion is still capable of being depolarized by an external stimulus distal to the site of injury with apparently normal stimulus conduction distally.
Axonotmesis and neurotmesis both result in physical disruption or separation of the nerve axons. With axonotmesis, axons are disrupted, but the perineurium and epineurium are spared so the nerve remains in physical continuity. With neurotmesis, complete disruption of the entire nerve occurs and there is loss of physical continuity. With axon disruption in either axonotmesis or neurotmesis, Wallerian degeneration will occur. The distal portion of the axon that is separated from its cell body will begin to degenerate within 3 to 10 days; when the process of Wallerian degeneration is complete, the remains of the nerve distal to the injury will no longer be able to conduct an electrical stimulus. From time of the initial nerve injury until the completion of Wallerian degeneration, distal portions of the disrupted axons will progress from normal conduction, depolarization, and transmission to a complete lack of response to an applied external stimulus. Therefore, Wallerian degeneration can be followed and measured with NCS. Shortly after injury, NCS demonstrate normal or near normal response amplitudes distal to the site of injury, despite complete transection. Over time, amplitude of the response decreases and after approximately 2 weeks, NCS demonstrate no response from the distal portion of the damaged nerve with axonotmesis or neurotmesis.
Likewise, timing of EMG studies is also significant. Immediately following a brachial plexus injury, an EMG of clinically weak or paralyzed muscles in the arm can be performed. Evidence of voluntary motor unit action potentials (MUAPs) in the muscle, despite the lack of clinical movement, provides conclusive evidence of some degree of preserved axon/nerve continuity. However, EMG studies cannot distinguish between neurapraxia and axonotmesis/neurotmesis in the context of decreased voluntary motor function; both present with decreased or lack of MUAPs in the involved muscle. With appropriate time elapsed, EMG findings can begin to differentiate between the different types of lesions. Axon disruption implies altered axon transport: the axon transports substances from the nerve cell body down the axon to the distal nerve, which are required to support the neuromuscular junction. As axon transport is disrupted and/or eliminated, the neuromuscular junction becomes unstable. Subsequently, the muscle fiber resting membrane potential rises from the normal −90 mv to 0 mv. As this occurs, muscle fibers begin to depolarize spontaneously or in response to minimal mechanical stimuli, which can be captured by EMG. The time needed for this process to occur at the neuromuscular junction and muscle membrane depends on length of the axon from the site of disruptive injury to the muscle. The closer the site of injury to the muscle, the sooner these spontaneous electrical discharges (positive waves and fibrillations) are seen on EMG; the farther the site of injury is from the muscle, the longer the time elapsed before positive waves and fibrillations are apparent on EMG. Generally this process may vary from 10 to 21 days; rare cases have been reported in which positive waves and fibrillations have not been documented to occur for a much longer period of time following a known peripheral nerve injury.
With degenerative axon injuries, abnormal spontaneous activity will be observed by EMG after approximately 3 weeks. In contrast, with neurapraxic lesions, there is no axon disruption and abnormal spontaneous activity is not observed on EMG. Even with an extensive neurapraxic lesion involving all of the axons innervating a muscle, a preserved compound muscle action potential (CMAP) will be present in NCS, but EMG demonstrates normal insertional activity without the presence of MUAPs. EDX studies can distinguish between neurapraxic and degenerative axonal lesions; however, they can not distinguish between the 2 degenerative axonal lesions (axonotmesis and neurotmesis).
Timing is also important when seeking evidence for muscle re-innervation following nerve injury. Re-innervation occurs by either of 2 processes: collateral re-innervation or re-innervation by axonal regrowth. Collateral re-innervation occurs with incomplete nerve injuries when surviving axons extend terminal sprouts to innervate surrounding denervated muscle fibers; in adults, this process commences approximately 12 weeks after injury. Re-innervation by axon regrowth may occur spontaneously with axonotmetic injuries because nerve continuity is maintained due to intact connective tissue. After Wallerian degeneration, the proximal ends of disrupted axons regrow from the point of injury distally to re-innervate muscle fibers. This type of re-innervation also occurs after surgical nerve repair or nerve transfer. The timing of axon regrowth and muscle re-innervation is related to location of axon injury, distance to the muscle, and rate of axon regrowth. In adults, axon regrowth is reported to be approximately 1 mm per day (or an inch per month); therefore, the practitioner can anticipate when evidence of voluntary motor unit activity can be observed in the target muscle by making a simple measurement along the course of the nerve.
Nerve conduction studies
NCS evaluate both the motor and sensory fibers of a peripheral nerve; either a sensory nerve action potential (SNAP) or CMAP is recorded. Stimulating and recording techniques have been described for most of the major peripheral nerve branches in the upper extremity. Some techniques are facile and are reproducible from examiner to examiner; others are quite technically challenging to perform and not reliably reproducible. When performing NCS, body surface temperature of the extremity should be maintained at 32°C or higher, because cooling slows nerve conduction artificially, thereby affecting the recorded responses. Active and reference recording electrodes are placed over the course of the sensory nerve for sensory NCS ( Figure 17.1 ). When studying motor nerve fibers, the active electrode is placed over the motor point of the muscle belly innervated by the relevant nerve, and the reference electrode is placed more distally over the muscle tendon ( Figures 17.2, 17.3 ). For both motor and sensory NCS, the nerve is stimulated at a standard distance (which varies from one EDX laboratory to another) from the recording electrode; a grounding pad is placed on the skin between the stimulating and recording electrodes. The intensity and/or duration of the applied electrical stimulus is increased until a supramaximal response is achieved, indicating that all axons that can be stimulated have been stimulated. Amplitude and latency (time from onset of stimulus to initiation of the response) of the response are recorded.



Typically, both onset latency and peak latency of the sensory response (SNAP) are recorded ( Figure 17.4 ). Onset latency is a reflection of the fastest conducting myelinated fibers, whereas peak latency is an approximation of the average conduction velocity of all the sensory fibers stimulated and recorded. Conduction velocity is directly proportional to diameter of the nerve fiber; therefore, the largest diameter myelinated fibers are the fastest. In sensory NCS, both the stimulus is applied and the response recorded directly from the same nerve; therefore, latency is a direct measure of the sensory conduction velocity, which can be calculated from latency and the distance between stimulation and recording sites. Sensory nerve conductions can be performed using either orthodromic (direction of physiologic nerve depolarization) or antidromic (opposite direction of normal depolarization) stimulation, which should provide similar results. Orthodromic sensory stimulation is more commonly used.

Assessment of motor nerve conduction requires stimulation of a nerve and recording the response (CMAP) from a muscle innervated by the nerve. Supramaximal CMAP amplitude is the summated electrical response recorded from all of the individual contracting muscle fibers. Both the amplitude and latency of the CMAP are recorded. Latency reflects the time from stimulation to onset of the response of the fastest fiber. Unlike sensory nerve conduction, motor latency does not correspond directly to motor nerve conduction velocity, but motor latency reflects a number of events including time of motor axon conduction, neuromuscular junction transmission, and depolarization of the muscle fiber membrane. Determination of motor nerve conduction velocity requires stimulating the motor nerve at 2 different sites, recording the latencies for each stimulation site, and measuring the distance between the 2 stimulation sites ( Figure 17.5 ). Conduction velocity is then obtained by dividing distance between stimulation sites by the time obtained after subtracting the distal (stimulation site) latency from proximal (stimulation site) latency:
CV motor = D between distal and proximal stimulation sites ( L proximal − L distal )
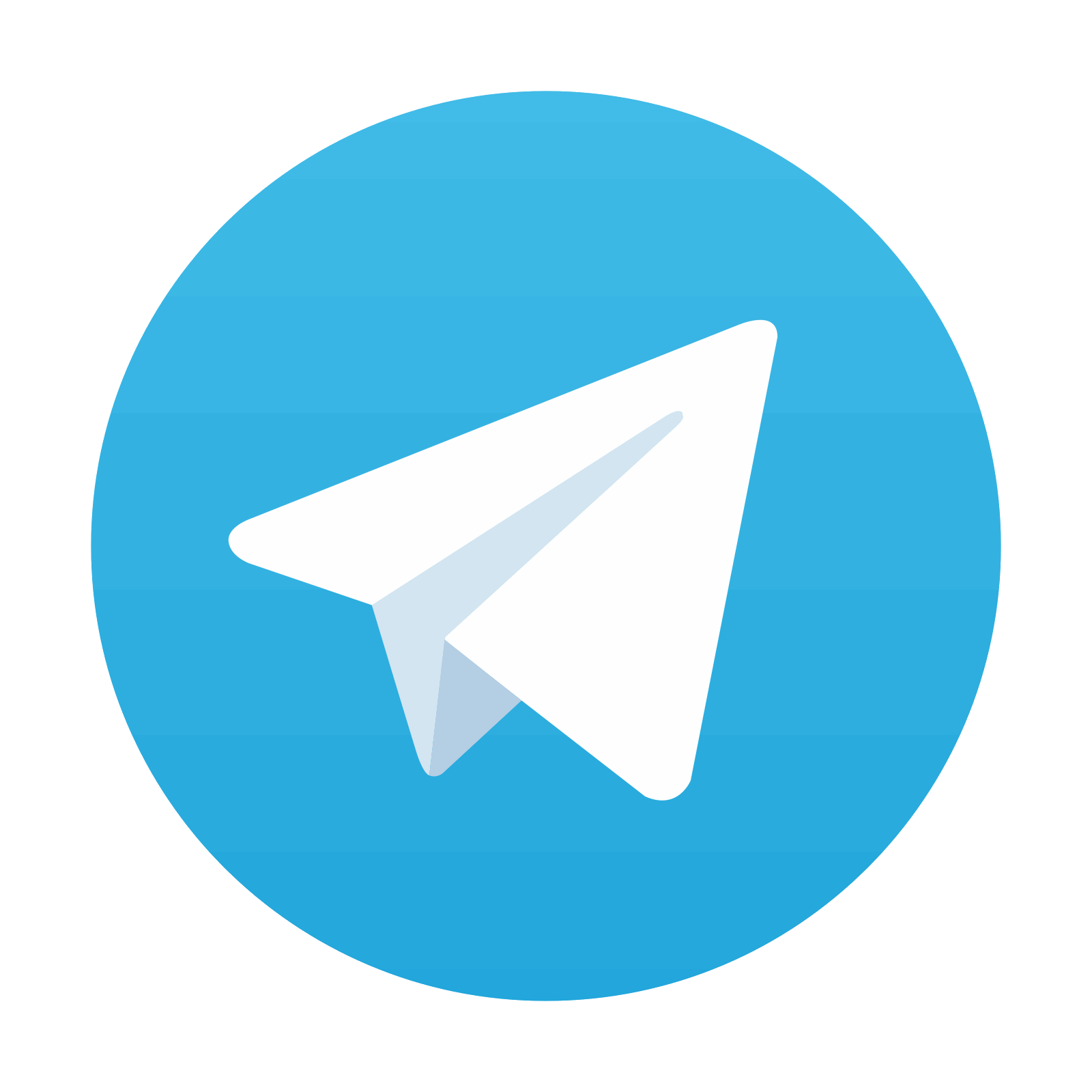
Stay updated, free articles. Join our Telegram channel

Full access? Get Clinical Tree
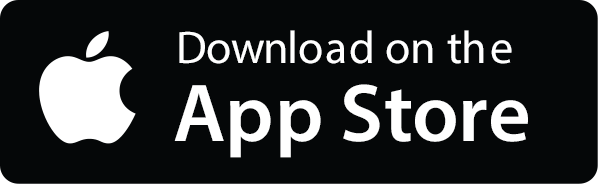
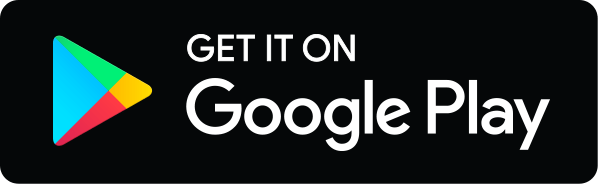