© Springer Science+Business Media New York 2015
Steven A. Olson, MD and Farshid Guilak, PhD (eds.)Post-Traumatic Arthritis10.1007/978-1-4899-7606-2_1616. Potential Mechanisms of PTOA: Inflammation
(1)
Research Division, Tissue Engineering, Regeneration and Repair Program, The Hospital for Special Surgery, Department of Cell and Developmental Biology, Weill Cornell Medical College, Caspary Research Building, 5th Floor, 535 East 70th Street, New York, NY 10021, USA
Keywords
CartilageChondrocyteOsteoarthritisMechanotransductionNuclear factor-kappaBExtracellular matrixSynoviumInflammationCytokinesChemokinesMouse modelsIntroduction
Post-traumatic osteoarthritis (PTOA) is a subset of osteoarthritis (OA) that results in similar end-stage pathology, but the initiating factors and how the disease progresses are quite distinct, thereby impacting on design of therapeutic approaches [1, 2]. OA is whole joint disorder with cartilage destruction as its hallmark, but involving changes in all joint tissues including ligaments, tendons, menisci, subchondral bone, and synovial membrane and capsule and current therapies involve symptomatic relief of pain, physiotherapy, and ultimately total joint replacement after joint failure [3, 4]. In PTOA, severe cartilage damage may occur months or years following the initial injury. The early loss of proteoglycans may not be easily detectable, except by MRI, and since the component glycosaminoglycans are renewable up to the pivotal point when more severe damage of the aggrecan core protein and disruption of the collagen network occurs, targeting early events are critical for halting the irreversible progression of OA disease.
Following the joint trauma accompanied by damage of ligaments, tendons, or menisci, the objectives of arthroscopic surgery are not only to repair these tissues but also to correct the biomechanics of the affected joint. However, orthopedic surgeons performing these procedures are aware that the presence and extent of inflammation in the joint may affect their outcomes. Thus, surgeries are often delayed until the inflammation, which is characterized by joint swelling and synovial effusion, subsides usually with treatment with anti-inflammatory drugs. Although most investigations have focused on the cartilage as the target in the subsequent development of OA and cartilage may be one source of early diagnostic biomarkers in the synovial fluids of PTOA patients, it may not be the critical target for anti-inflammatory therapy immediately following trauma. In addition to synovial inflammation and effects of inflammatory mediators on cartilage, other tissues, including tendons, ligaments, and menisci, have important roles in maintaining joint biomechanics and contain cellular targets for inflammatory and mechanical signals [5].
A large number of studies have shown that there are different etiologies and time courses that result in the initiation and development of OA in patients that undergo operative procedures. Epidemiologic studies have established that there is a strong relationship between ACL disruption and the risk for subsequent development of OA [6–10]. Injuries to the ACL frequently occur in young patients, especially in athletes, leading to pain and functional impairment in young or middle-aged adults. The reported radiographic rates of OA after an ACL injury vary between 10 and 90 % at 10–20 years after the ACL injury [11, 12]. Studies of populations with meniscal injury have also been useful for identifying risk factors for PTOA [13]. Meniscal injuries are commonly seen in association with ACL injury [14, 15]. These subsets of PTOA are represented within a continuum of early, progressive and end-stage OA and include (1) anterior cruciate ligament (ACL) injury (<35 years of age); (2) acute meniscal injury (26–40 years of age); (3) degenerative meniscus (40–65 years of age); and (4) total joint replacement (>50 years of age).
Role of Inflammation in Cartilage Damage in PTOA
Injury to the ACL or meniscus alters the biomechanical dynamics of the joint, often in a young and otherwise healthy individual, and affects joint kinematics [15]. In this environment, the altered distribution of forces applied to the articular cartilage articular cartilage leads to altered mechanotransduction in the chondrocytes, activation of catabolic and inflammatory genes, deregulated matrix synthesis, and decreased repair capacity [2, 9]. While loss of articular cartilage inevitably occurs in PTOA, the cartilage responses are substantially different depending upon whether the trauma results in acute injury or chronic loading abnormalities. Traumatic injuries that result in intraarticular fracture are associated with focal loss of chondrocyte viability, due to either necrosis or apoptosis, and altered cartilage structure. In acute ACL injuries, cartilage lesions can appear macroscopically as chondral softening, chondral fractures, impaction lesions, creases, crack, or flaps and occur in the lateral compartment of the knee [16]. In chronic ACL deficiency, the joint kinematics may alter the tibiofemoral biomechanics and the normal pattern of loading on the articular surface of the knee, leading to recurrent episodes of instability. The severity of the cartilage defect graded by the Outerbridge score is correlated with age, BMI, and the chronicity of the ACL rupture [17]. While clinical outcomes are closely linked to the severity of the trauma and the subsequent cartilage degeneration, it is likely that collective damage to all joint structures and their cellular responses contribute to joint pathology and that cartilage damage may not progress rapidly without inflammation in the synovial compartment. For example, when cartilage explants undergo mechanical injury in vitro, the presence of synovial capsule shifts the chondrocyte responses to pro-catabolic pathways [18].
Although injury and traumatic joint loading are considered initiating factors in events that lead to PTOA, they are accompanied by the release of inflammatory mediators from the synovium and other joint structures [19]. The signaling events activated in chondrocytes and other resident cells are common to both mechanotransduction and cytokine stimulation [20]. A large number of studies have addressed interleukin-1 (IL-1) as a major inflammatory cytokine that drives the progression of the disease (see for review [21, 22]). Chondrocytes in OA cartilage, especially those in clonal clusters, have cell surface receptors that can respond to cytokines and chemokines produced in the synovium and other periarticular joint tissues and detected in OA synovial fluid. Although long assumed that IL1B mRNA induced in chondrocytes could result in secretion of IL-1β, the inflammasome complex consisting of NALP-3 and the IL-1β activator caspase-1 in OA cartilage, does not participate in activating the pro-IL-1β so that it can be secreted and act in an autocrine manner [23]. Many studies have shown that inflammatory cytokines stimulate expression of MMP-3, -9, and -13, which co-localize with type II collagen cleavage epitopes in regions of matrix depletion in OA cartilage and species-specific up-regulation of ADAMTS-4 and -5 in chondrocytes by inflammatory stimuli has been reported in OA cartilage [24–26].
Early studies showed that IL-1 is a potent inducer of the catabolic program, promoting cartilage matrix loss even at picomolar levels. Both in vitro and in vivo studies have demonstrated its ability to induce proteolytic enzyme synthesis in chondrocytes, driving enhanced production of matrix metalloproteinases (MMPs) and aggrecanases that degrade the cartilaginous matrix. IL-1 is also a primary mediator of the inflammatory cascade by stimulating articular cells to produce numerous downstream effector molecules, including nitric oxide (NO), phospholipase A2, prostaglandin E2 (PGE2), as well as other cytokines such as TNFα, IL-6,-8,-15,-18 and chemokines, including IL-8, CXC-2,-5,-10, and CCL-3,-5,-7. Release of these agents further stimulates cartilage matrix degradation, bone erosion, synovitis, fibrosis, and pain sensitivity.
A critical role of IL-1 in the development of OA has been demonstrated in vivo in several animal models of PTOA, in which transection of the ACL induces IL-1 synthesis by the synovium and articular chondrocytes and the knee joints predictably develop pathologies of OA [27, 28]. Administration of IL-1 receptor antagonist (IL-1Ra), a naturally occurring inhibitor of IL-1 signaling, is soon after ACL transection by repeated intra-articular injections can effectively suppress early degenerative changes in the articular cartilage of the tibial plateau and femoral condyles, block MMP synthesis, and reduce the number and size of osteophytes [29, 30]. However, it has not been possible to achieve and maintain sufficiently high intraarticular concentrations of recombinant IL-1Ra (Anakinra), which is required at 50-fold higher concentrations than IL-1. Thus, in vivo gene delivery of IL-1Ra, using a safe and effective form of adeno-associated virus, is currently under investigation in a large mammalian preclinical model, equine PTOA, as a proof-of-principle for clinical translation to human PTOA [31, 32].
Lessons from Mouse Models
We have learned much about the molecular mechanisms driving cartilage destruction from studies of gene and protein expression in clinical material and in culture models derived from human tissues. However, it is not possible to study the time course of the disease in vivo in humans. Studies in animal models, in which the ACL or other knee ligaments are transected or injured, generally model aspects of PTOA that may be translated to humans. Although the focus has been on the biomechanical impact on cartilage damage [33], the availability of transgenic and knockout mice has permitted the examination of roles of inflammatory genes and associated pathways in promoting the initiation and development of OA. Among the models of PTOA in mice [34, 35], the surgical model of destabilization of the medial meniscus (DMM) has become the gold standard for studying the time course of cartilage destruction during disease development and progression. Notably, the DMM model has been used to demonstrate the importance of the key aggrecan- and collagen-degrading enzymes in cartilage destruction, including ADAMTS5 [36, 37] and MMP13 [38], but also the consequences of knockout of key inflammatory genes such as IL-1 [34]. However, depending upon the surgical technique, the contribution of the fat pad, which may or may not be left in the joint, the nature of the gene knockout or knock-in, and the time of tissue sampling, there is generally less synovial inflammation in this model than we would expect in PTOA in humans [39]. The dysregulation of genes in the acute phase response signaling category in both wild type and Adamts5∆cat mice at 1 week following DMM surgery suggests an early inflammatory reaction that is independent of aggrecanase activity [40].
Alternative to the surgically induced PTOA models are noninvasive loading models in which repetitive in vivo cyclic compression causes cartilage degeneration depending upon the peak load level [41, 42]. Also notable are the rapid subchondral bone changes [41], although whether synovial inflammation is a feature has not yet been thoroughly evaluated. However, whether the model has an inflammatory component may depend upon the genetic background, since the strain least susceptible to DMM-induced cartilage loss, DBA/1, is highly susceptible to autoimmune or inflammatory arthritis [34]. The C57BL/6 strain, used most frequently for generation of knockout and transgenic mice either alone or on a mixed background with 129/SvDv strains, has intermediate susceptibility, indicating its utility whether the genetic modification is expected to enhance or attenuate cartilage loss following challenge. Vincent and Saklatvala [43] pointed out the prominence of repair response genes in late OA in both surgical PTOA models human cartilage, which is reflected in ex vivo cartilage explant culture models [44], in contrast to the noninvasive cartilage injury models, which exhibit more obvious bone phenotypes. More severe mouse models result from intra-articular fracture, in which the joint pathology involves synovial inflammation, bone morphological changes, and increased circulating inflammatory cytokines and biomarkers, as well as chondrocyte death and cartilage degeneration within 7 days post-fracture [45].
Gene profiling studies of cartilage or whole joints from experimental PTOA models have begun to identify pathways that may be targeted for therapeutic development [46–49]. Age-related responses in joint tissues are accelerated in PTOA mouse models [49], as well as in spontaneous OA models such as the Str/ort mouse [50], and involve upregulation of genes of the senescence-associated secretory phenotype, including inflammatory cytokines (IL-6, IL-33, etc.), chemokines, MMPs, and immune and defense response genes [39, 51]. The molecular phenotypes resemble those reported in profiling studies of human cartilage [52, 53], in which both catabolic and anabolic gene signatures have been identified. Together, the findings to date suggest that the OA signature may be specific to the disease and unrelated to aging and lend credence to the possibility of identifying gene signatures in at-risk populations, including those susceptible to PTOA, prior to the onset of overt disease.
The prominence of the NF-κB pathway as a common thread among the different gene signatures has been emphasized in these preclinical PTOA models, suggesting a key regulatory role for stress and inflammatory signaling via canonical NF-κB signaling in human OA (see for review [22, 54]). The findings that mechanical stimuli modulate NF-κB signaling [20] provide an explanation for why NF-κB-related gene signatures may be upregulated in mouse models of PTOA in the absence of overt signs of inflammation such as synovitis and immune cell infiltration. Such profiling studies have revealed that inflammatory signatures are present before the appearance of overt OA and, in some models, are associated with increased numbers of activated T- and B-lymphocytes in the spleens of the mice destined to develop OA [50].
Contributions of Synovial Inflammation to PTOA
As highlighted in Chap. 12, synovitis is frequently observed at the time of arthroscopy, as well as at various times following joint injury, and may be a major prognostic indicator of the rate of development of PTOA. MRI findings correlate with microscopic and macroscopic evaluations of synovitis and suggest that synovitis is often present soon after a traumatic event [55]. ACL rupture by itself may not have high impact, but the synovitis is often more severe, if it is accompanied or followed by meniscal injury, collateral ligament tear, cartilage damage, and bone contusion. The acute symptoms following joint injury include joint pain and swelling due to intraarticular bleeding, synovial effusion and inflammation [2, 9]. The low-grade synovitis following injury is associated with the infiltration of mononuclear cells, including activated B cells and T lymphocytes, and production of inflammatory cytokines [19, 56, 57]. Overall, the presence of synovitis correlates with more rapid progression to structural deterioration, suggesting a role for cross talk between cartilage and synovial tissues [58–64].
In cases of ACL injury accompanied by cartilage and meniscal damage or of traumatic meniscal injury with no radiographic evidence of OA, the synovium retrieved during arthroscopy is frequently inflamed and synovitis is most commonly observed in the suprapatellar region remote from the sites of injury [65]. Inflammation scores are associated with increased pain and dysfunction, and inflammatory infiltrates, including cells of the innate (macrophages) and adaptive (T and B cells) immune systems, can be observed within the synovial membrane. Importantly, microarray analyses show unique cytokine and chemokine profiles [66, 67], including increased IL-15 mRNA in synovial membranes of patients with degenerative menisci [66] and increased expression of genes encoding the chemokines, IL-8, CCL19, CCL21, and CCL5 and the chemokine receptor, CCR7, associated with synovial inflammation in patients undergoing arthroscopy for treatment of acute meniscal injury [67]. Generally, it is believed that the synovial changes represent a generalized early response to the injury and the presence of synovial inflammation increases the likelihood of cartilage degeneration and progression to PTOA. The damaged meniscus is an additional source of inflammatory cytokines, chemokines, and reactive oxygen species that could promote expression and activation of proteolytic enzymes and adversely affect cell survival and synthetic activity of chondrocytes and other joint tissues [68, 69].
Inflammatory Biomarkers in PTOA
Investigations of the biomarkers in synovial fluids, blood, and urine have attempted to map the disease process in OA patients in order to develop methodologies for diagnosis or response to therapy [70]. Distinct categories of biomarkers have been defined based on tissue origin and/or pathological process and include inflammatory mediators such as cytokines and chemokines, acute phase proteins, wound repair markers, extracellular matrix degradation products, and proteinases [9, 71–75]. Studies evaluating synovial fluids aspirated from the injured knees of patients with acute, subacute, and chronic ACL deficiency have shown an association between inflammation and increased concentrations of several cytokines. Following the acute ACL rupture, the initial burst of production of cytokines, such as tumor necrosis factor (TNF)α, IL-6, IL-8, IL-10, interleukin (IL)-1β, and IL-1ra, is similar to that observed in wound healing. The inflammation subsides but does not completely resolve, with persistence of a cytokine imbalance involving dramatically decreased concentrations of IL-IRa in patients with chronic ACL deficiency, suggesting insufficient levels of this protective cytokine to neutralize IL-1β [72, 76, 77]. Higher serum C-reactive protein (CRP), an acute phase reactant found in patients with cardiovascular disease and inflammatory arthritis, is present in patients undergoing arthroscopy in association with synovial inflammation [57, 71].
Other candidate biomarkers released in association with joint trauma include the damage associated molecular patterns (DAMPs), also known as alarmins, which can interact with the Toll-like receptors (TLR)-2 and -4 and receptor advanced glycation end-products (RAGE). DAMPs include extracellular matrix products and other ligands such as high mobility group box-1 (HMGB1), S100A8 (MRP8, calgranulin A) and S100A9 (MRP14, calgranulin B), and serum amyloid A (SAA). They can induce cellular inflammatory responses via NF-κB and are indicators of innate immunity that may drive synovitis in OA [78]. Other candidate biomarkers that may also be effectors of the disease include the complement proteins [71, 79] and uric acid, which is a danger signal associated with inflammasome activation [80]. Correlation of the transcriptomes of synovium and cartilage with synovial fluid proteomes from different OA phenotypes and with clinical and outcomes data has provided the opportunity to identify potential biochemical markers for monitoring the effects of joint injury on the clinical course and, importantly, for gaining insights into the mechanisms associated with development and progression of disease [81].
The observations of persistent and evolving disturbances in the cytokine profiles suggest that, in addition to the effects of the adverse biomechanical environment, biological processes also contribute to the development of OA changes after ACL or meniscus injury and indicate a potential role of synovitis in the pathogenesis PTOA. Understanding the mechanisms involved in the initiation and perpetuation of this inflammatory process in PTOA could provide more sensitive and specific tools for monitoring patients after ACL or meniscus repair and also lead to the development of more specific and effective therapeutic approaches to improve the outcomes. Early targeted therapies against events associated with mechanical and inflammatory stress and injury include antioxidants, which prevent release of reactive oxygen species due to cell death, mitochondrial dysfunction, and cytoskeletal disruption, mitogen-activated protein kinase inhibitors, activators of ATP-AKT pathway signaling, and blockade of alarmins/DAMPs [82].
Significance
Joint trauma affects all joint tissues to some degree, but once cartilage is degraded, it is particularly refractory to repair by resident cells. While regenerative medicine techniques are not sufficiently advanced to substitute for end-stage joint replacement, the joint damage that occurs following injury is amenable to early intervention to prevent subsequent development of PTOA. Arthroscopic procedures are routinely employed in young individuals following ACL or meniscal damage, and this population could provide a window of opportunity for therapy targeted at preventing synovial inflammation and cartilage damage during the days and weeks after injury. There is a critical need for rigorous clinical and laboratory studies to define the factors that are responsible for joint deterioration associated with injury of the meniscus or ACL. Animal models of acute or chronic joint injury have been informative for defining the factors involved in the initiation and perpetuation of PTOA and could lead to the identification of new therapeutic targets. Inflammatory and catabolic biomarkers associated with synovitis and cartilage damage may have diagnostic or predictive value for defining outcomes pre- and post-arthroscopy and long-term risk for the development of PTOA. They could also help to identify patient cohorts for evaluating new therapies targeted at inflammation and the associated tissue damage.
References
1.
Anderson DD, Chubinskaya S, Guilak F, Martin JA, Oegema TR, Olson SA, Buckwalter JA. Post-traumatic osteoarthritis: improved understanding and opportunities for early intervention. J Orthop Res. 2011;29:802–9.PubMedCentralPubMedCrossRef
2.
Lotz MK, Kraus VB. New developments in osteoarthritis. Posttraumatic osteoarthritis: pathogenesis and pharmacological treatment options. Arthritis Res Ther. 2010;12:211.PubMedCentralPubMedCrossRef
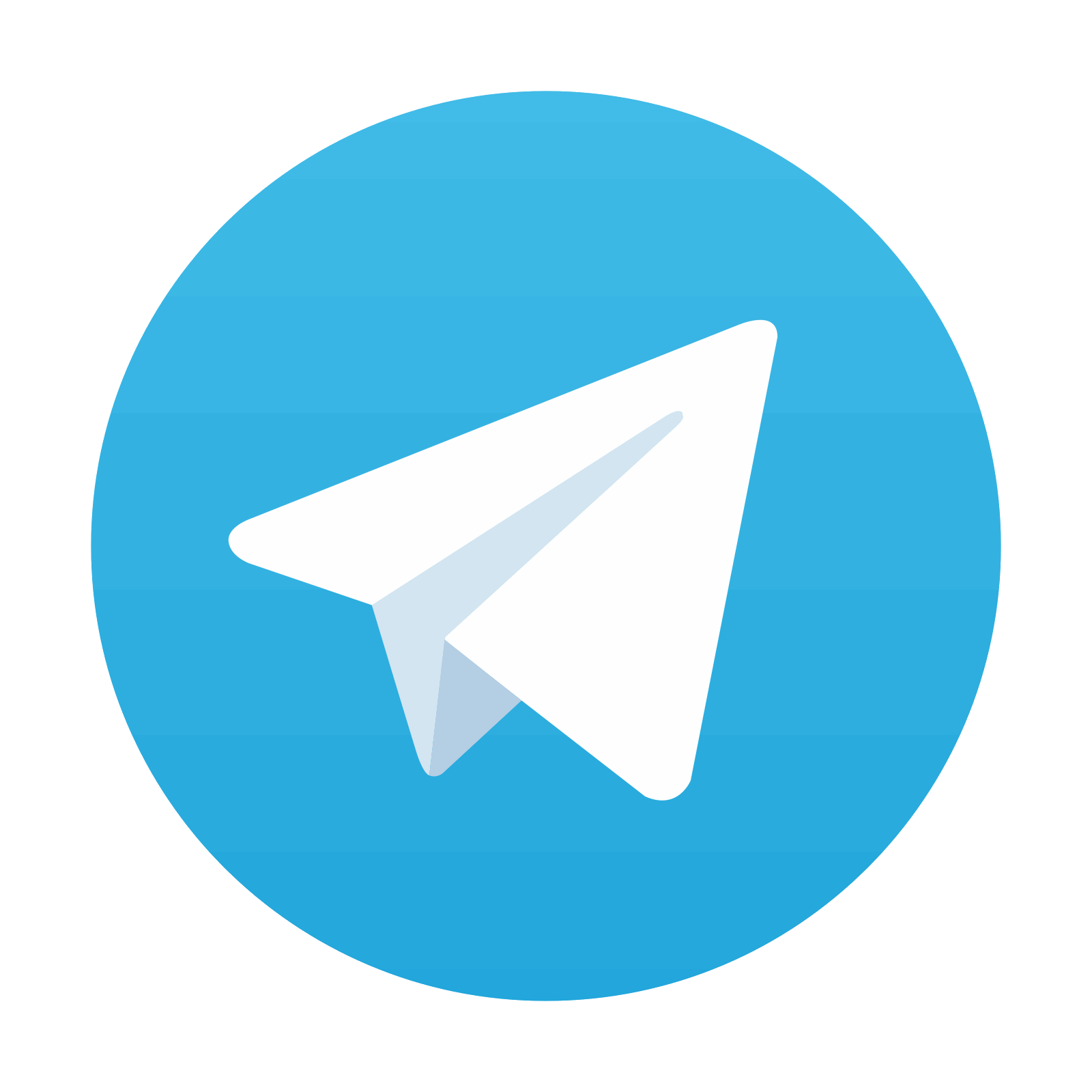
Stay updated, free articles. Join our Telegram channel

Full access? Get Clinical Tree
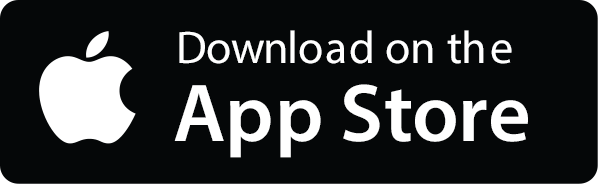
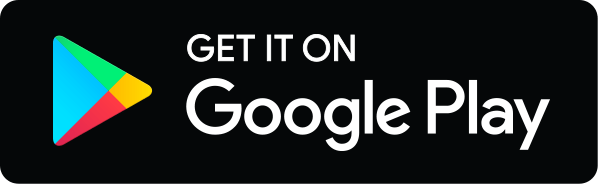
