Anatomy and Function
The lateral aspect of the knee is composed of 28 unique structures that act as static and dynamic stabilisers of the knee during varus and rotational motion. To properly appreciate the anatomy and function of these structures, one must first understand the local bony anatomy. The opposing bony surfaces of the lateral femoral condyle and the lateral tibial plateau articulate in a convex on convex manner, creating an inherently unstable region of the knee ( Fig. 9.1 ). , Animal model studies have demonstrated that injuries to this area, given the bony morphology, tend to heal poorly, leaving the knee susceptible to medial compartment osteoarthritis, lateral compartment gapping and medial meniscus injury.

There are three major static stabilising structures of the posterolateral corner (PLC): the fibular (lateral) collateral ligament (FCL), popliteus tendon (PLT) and popliteofibular ligament (PFL). These structures provide the primary resistance to both the varus forces of the knee and posterolateral rotation of the tibia about the femur.
Fibular (Lateral) Collateral Ligament
The FCL is the primary varus stabiliser of the knee and also has a significant role in stabilisation against early external rotation at early flexion levels. The femoral attachment is located slightly proximal (1.4 mm) and posterior (3.1 mm) to the lateral epicondyle in a small bony depression. This attachment site is approximately 18.5 mm proximal and posterior to the PLT attachment site when the knee is at 70 degrees; this relationship is important in anatomical reconstruction techniques. The proximal attachment site is best identified by making a longitudinal incision through the iliotibial band (ITB). Distally the FCL extends through the biceps bursa to reach the primary distal attachment site, which is located in a bony depression on the fibula head: 8.2 mm posterior to the anterior margin of the fibular head and 28.4 mm distal to the tip of the fibular styloid process. The remaining portion of the distal insertion blends with the peroneus longus fascia. On average, the FCL is 69.6 mm in length ( Fig. 9.2 ).

Popliteus Tendon
The femoral insertion of the PLT constitutes the most anterior femoral insertion of the PLC; as stated previously, the PLT insertion is located 18.5 mm from the FCL femoral insertion site ( Figs 9.3 and 9.4 ; see Fig. 9.2 ). The popliteus muscle originates on the lateral aspect of the femur and extends posterior and distally in an oblique fashion to insert at a broad attachment site on the posterior aspect of the tibia. This femoral attachment footprint is located posterior to the margin of the lateral femoral condyle articular cartilage and at the anterior fifth of the popliteal sulcus. It becomes tendinous in the lateral third of the popliteal fossa and intraarticular as it courses deep to the FCL. As the tendon passes through the popliteal hiatus, it becomes anchored to the lateral meniscus by three popliteomeniscal fascicles: anteroinferior, posterosuperior and posteroinferior fascicles. These fascicles form the border of the popliteal hiatus. The popliteomeniscal fascicles have been shown to play a significant role in anterior motion of the meniscus when loaded. The average total length of the PLT is 54.5 mm.


From full extension until approximately 112 degrees of knee flexion, the PLT rests proximal to the popliteal sulcus on the lateral femoral condyle. At more than 112 degrees of flexion, the PLT engages in the popliteal sulcus. Just medial to the posteromedial aspect of the fibular head, the PLT connects to the popliteus muscle belly at its musculotendinous junction.
Popliteofibular Ligament
The PFL, previously known as the arcuate ligament, has distinct anterior and posterior divisions and functions primarily to anchor the musculotendinous junction of the popliteus muscle to the fibular head (see Figs 9.2 and 9.4 ). The distolateral attachment of the anterior division is located on the anterior downslope of the medial aspect of the fibular styloid process. The posterior division attaches at the apex and posteromedial aspect of the fibular styloid process distally and has a larger width (5.8 mm) than the anterior division (2.8 mm). Further, the PFL and PLT form an 83-degree angle at their junction; this spatial relationship is important during anatomical reconstruction.
Additional Secondary Structures
Additional secondary structures help stabilise the knee in a static and dynamic manner. These structures include the anterolateral ligament, midthird lateral capsular ligament, coronary ligament, lateral gastrocnemius tendon, fabellofibular ligament, long head of the biceps femoris and ITB.
The anterolateral ligament (ALL) is a thickening located within the lateral capsule that comes under tension during internal rotation when the knee is at 30 degrees of flexion. The femoral attachment is located posterior (2.8 mm) and proximal (2.7 mm) to the FCL attachment; the tibial attachment is approximately midway between the centre of Gerdy tubercle and the anterior margin of the fibular head. Studies have shown that Segond fractures usually occur from the tibial attachment site of the ALL.
The midthird lateral capsular ligament is a thickening of the lateral capsule that attaches to the femur near the lateral epicondyle. It has a capsular attachment to the lateral meniscus and attaches to the tibia just distal to the lateral articular cartilage, between the posterior border of Gerdy tubercle and the anterior edge of the popliteal hiatus. Effectively the midthird lateral capsular ligament is comparable to the deep medial collateral ligament on the medial side of the knee.
The coronary ligament of the lateral meniscus is defined as the meniscotibial portion of the posterolateral joint capsule. The ligament connects the lateral meniscus to the lateral edge of the tibial plateau at a point distal to the articular cartilage. , The ligament begins laterally at the tibial attachment of the posterior cruciate ligament (PCL) and forms the medial border of the popliteal hiatus as it continues laterally to the lateral meniscus. , Visualisation of the meniscotibial capsular attachment can best be achieved through elevating the lateral meniscus with a probe on arthroscopy.
The lateral gastrocnemius tendon arises from the far lateral portion of the gastrocnemius muscle belly at or near the supracondylar process of the femur. The femoral attachment site is located an average of 13.8 mm posterior to the FCL attachment site and 28.4 mm from the PLT attachment. At the level of the fabella, or a cartilaginous analogue, it became adherent to the meniscofemoral portion of the lateral capsule proximal to the fabella. The lateral gastrocnemius muscle and tendon are potential landmarks for isolated FCL or complete PLC reconstructions because the interval between the lateral gastrocnemius (posterior) and soleus muscle (anterior) can be expanded using blunt dissection, after a common peroneal nerve neurolysis is performed. ,
The fabellofibular ligament is the distal thickening of the capsular arm of the short head of the biceps femoris. Of note, in a minority of cases the fabella is a sesamoid bone, and more often a cartilaginous analogue, that is found within the proximal lateral gastrocnemius tendon. The fabellofibular ligament originates at the fabella proximally and extends vertically from the fabella to just lateral of the lateral aspect of the fibular styloid process ( Fig. 9.5 ). In the absence of the fabella, the ligament is much less prominent, originating on the posterolateral femoral condyle and inserting lateral to the lateral fibular styloid process. , , ,

The long head of the biceps femoris originates at the ischial tuberosity of the pelvis and extends distally through the posterior and lateral aspects of the thigh until it attaches to the posterolateral and lateral aspect of the fibula using both a direct and anterior arm. The direct arm attaches laterally to the fibular styloid on the lateral aspect of the fibular head. The anterior arm attaches lateral to the FCL fibular attachment site on the fibular head. Between the two arms’ attachment sites lies the biceps bursa (FCL–biceps bursa), which is accessed to assess the distal FCL footprint. Additionally, three fascial connections contribute to the distal attachment: the reflected arm, lateral aponeurosis and anterior aponeurosis. ,
The short head of the biceps femoris originates medial to the linea aspera on the distal femur and courses distally and laterally. It has multiple attachments throughout its course, which include connections to the anteromedial side of the long head of the biceps tendon, posterolateral aspect of the joint capsule, capsulo-osseous layer of the ITB, lateral aponeurosis and both the direct and anterior arms of the long head of the bicep. The attachment to the direct arm is located at the fibular head between the fibular styloid and the distal FCL attachment site and is the most prominent attachment. Of note, the anterior arm of the short head of the biceps attaches 1 cm posterior to Gerdy tubercle. ,
The ITB is the most superficial layer of the lateral aspect of the knee. It originates at the anterolateral external lip of the iliac crest and extends distally to the anterolateral aspect of the tibia at Gerdy tubercle. Additionally, the ITB attaches distally via both the iliopatellar band, which is an anterior extension of the ITB that extends to the patella and the deep capsulo-osseous layers. The ITB consists of four layers, three of which comprise the superficial layer (iliopatellar band, deep fibres, capsulo-osseous) and cover the more posterior aspect of the vastus lateralis muscle. Significantly, the capsulo-osseous layer has been noted to form an anterolateral sling over the anterolateral aspect of the knee and contributes to alterations in the pivot shift manoeuvre in the setting of a concomitant anterior cruciate ligament (ACL) tear. This portion of the ITB was historically reconstructed in extraarticular ACL reconstructions. During an open PLC procedure, the ITB must be incised longitudinally to properly assess the FCL and PLT attachment site.
The proximal tibiofibular joint ligaments are composed of both anterior and posterior ligaments that connect the proximal aspect of the medial fibular head to the lateral aspect of the tibia and provide stability to the knee joint. The anterior tibiofibular ligament attaches 15.6 mm posterolateral to Gerdy tubercle and 17.3 mm anteroinferior to the fibular styloid. The posterior tibiofibular ligament attaches 15.7 mm interior to the lateral tibial plateau articular cartilage and 14.2 mm medial to the fibular styloid. ,
Other Important Structures
The common peroneal nerve innervates the lower extremity and is supplied by the spinal nerve roots L4–S2. It emerges from a bifurcation of the sciatic nerve in the posterior thigh and courses along the biceps femoris and around the neck of the fibula until it splits into the superficial and deep peroneal nerve. The sensory division includes two articular branches, one recurrent articular nerve and the lateral sural cutaneous nerve. Motor function of the common peroneal nerve and its branches includes foot eversion, plantar flexion, toe extension and other intrinsic foot movements. The superficial peroneal nerve innervates the muscles of the lateral compartment of the leg: the peroneus longus and peroneus brevis muscles. The deep peroneal nerve innervates the muscles of the anterior compartment: tibialis anterior, extensor hallucis longs and extensor digitorum longus. The peroneal nerve is injured in 13% to 16.7% of PLC injuries, and the nerve injury is most likely secondary to the initial traction injury with a hyperextension or varus force and also because of hematoma formation and subsequent nerve compression ( Fig. 9.6 ).

The lateral inferior genicular artery emerges from the popliteal artery and courses extraarticularly along the lateral joint capsule. Along the lateral aspect of the knee, the artery winds anteriorly, coursing anterior to the fabellofibular ligament and posterior to the PFL. This artery travels either within or adjacent to the ALL. During PLC procedures this artery must be identified because it can serve as an aid in anatomical identification of important structures, particularly the fabellofibular ligament and the PFL, and because bleeding from this artery can cause hematoma formation and transient peroneal neuropraxia. , ,
Biomechanics
Role of Posterolateral Structures in Preventing Varus Rotation
As demonstrated in biomechanical studies of the knee, the FCL is the primary restraint to varus motion through all degrees of knee flexion, and, as such, during isolated sectioning of the FCL, a significant increase in varus rotation is observed. Notably, sectioning the other structures of the PLC in isolation does not cause significant changes in varus rotation when the FCL is intact. , Both Gollehon et al. and Grood et al. proved through sectioning studies that in the FCL-deficient knee, both the PLT and the lateral capsular structures play a significant role as secondary varus stabilisers. When both the PLT and lateral capsular structures were sectioned after the FCL, the varus opening became significantly larger than the isolated FCL-deficient knee. ,
Further, both cruciate ligaments have been noted to have secondary varus-stabilising capabilities. Sectioning studies show that, in the PLC-deficient knee, sectioning of either the PCL or the ACL resulted in significantly increased varus opening compared with an isolated PLC tear.
Role of PLC in Preventing Anterior Translation
In a healthy knee the PLC has a minimal role in the primary prevention of anterior tibial translation, but the functional diversity of the PLC structures becomes apparent in the ACL deficient knee. In an ACL-deficient knee, the medial meniscus and the PLC function as secondary stabilisers, with the PLC acting to prevent anterior translation of the tibia, primarily in the early degrees of flexion. Kanamori et al. reported that in the ACL-deficient knee, the in situ forces on the PLC structures increased by 123% at full extension and 413% at 15 degrees of flexion. These biomechanical studies have been supported in the clinic by Noyes et al., who found that ACL deficiency and varus malalignment result in increased laxity on the PLC structures noted during manual testing. This conclusion is clinically important because patients who present with a significant amount of anterior tibial translation, 3+ or 4+ on the Lachman test, should cause an increased clinical suspicion for ACL tear in addition to concomitant PLC involvement.
Role of the PLC in Preventing Posterior Translation
Although minor, the PLC plays a role in restricting posterior tibial motion at all angles of the knee, with the largest amount of posterior tibial translation occurring close to full extension. , , , Its prominent role as a secondary posterior stabiliser can be observed in PCL sectioning studies; in PCL deficient knees, sectioning of both isolated PLT and combined PLT plus another PLC structures resulted in significant increases in the amount of posterior tibial translation. Thus, in a patient with 3+ posterior drawer test or more, or posterior translation of greater than 12 mm on bilateral PCL stress radiographs, concurrent PLC injury should be suspected.
Role of PLC in Preventing Internal Rotation
Several studies have shown that sectioning both the isolated FCL and other combined PLC structures results in a significant increase of internal rotation in the joint. , , According to the literature, in the ACL-deficient knee, sectioning PLC structures caused significant increase in internal tibial rotation, most significantly when the knee is near full extension.
Role of the PLC in Preventing External Rotation
It is well documented that the PLC plays an integral role in preventing external rotation of the knee. The greatest amount of external rotation is at 30 degrees of flexion, where the knee averages between 13 and 17 degrees of external rotation. At increased degrees of knee flexion, the role of the PLC becomes even more prominent. When the PLC was sectioned in isolation and the knee was flexed to 90 degrees, there was a 5- to 7-degree increase in external tibial rotation. This finding is clinically significant because these sectioning studies provide the biomechanical justification for the dial test, a commonly used clinical examination manoeuvre used to diagnose PLC injuries. Further, in an isolated PLC injury, results of the dial test should yield decreased external rotation of the tibia on the femur at 90 degrees compared with the dial test at 30 degrees of knee flexion ( Fig. 9.7 ). However, in a combined PLC cruciate ligament injury the dial test will reveal significantly increased external rotation at both 30 degrees and 90 degrees.

Biomechanical Failure of the PLC Structures of the Knee
The individual failure characteristics of the three major static stabilisers of the PLC have been described in the literature. In two separate studies the mean ultimate tensile strengths of the FCL (295 N and 309 N), PFL (298 N and 180 N) and PLT (700 N) were determined. , Further, these structures were tested for combined tensile strength (FCL, 750 N; PFL, 425 N). With this analysis, surgeons are better able to determine which graft type to use for reconstructions; viable options for grafts include semitendinosus tendon (maximum failure load, 1216 N), central quadriceps tendon (maximum failure load, 1075 N) and Achilles tendon allograft (maximum failure load 3055 N). ,
The Effects of Deficient PLC Structures on Cruciate Ligament Grafts
Several studies have demonstrated that there is a significantly increased force on both cruciate ligaments in PLC-deficient knees. Specifically, sectioning studies involving ACL reconstructed knees have provided valuable insights. After sectioning the FCL during varus loading at both 0 degrees and 30 degrees of flexion, as well as coupled loading of varus and internal rotation moments at 0 degrees and 30 degrees of flexion, a significant amount of increased force on the ACL graft was noted. The increase in force on the graft was maintained throughout the sectioning of the PFL and the PLT. In a patient with deficiencies of all three of the static stabilisers of the PLC, experiencing a 6% adduction moment of the knee, and of average weight and height, LaPrade et al. calculated a 444 N force on the reconstructed ACL graft. Comparing this value to the accepted literature on the failure strength of a new patellar tendon graft (416 N) after reconstruction, one can see that the force from the normal adduction moment in a PLC-deficient knee exceeds the maximal failure load of a newly reconstructed graft. This conclusion strongly suggests that in the setting of combined ACL and PLC injuries, the PLC must be addressed at the time of ACL procedure.
In a subsequent study the static stabilising structures of the PLC were sectioned to assess the subsequent changes in tension on a reconstructed PCL graft. The largest increase in force on the graft occurred with both varus moments and with coupled posterior drawer force and external rotation torque. These loading states caused a significant amount of force on the graft when the knee was loaded at 30 degrees, 60 degrees and 90 degrees of flexion. As with the conclusion related to ACL and PLC injuries, in the setting of a combined PCL and PLC injury, the PLC must be addressed at the same time as the PCL to avoid excessive force on the newly constructed, vulnerable graft.
Mechanism of Injury
Possible mechanisms of injury to the PLC include a posterolateral-directed force to the anteromedial tibia with the knee in hyperextension (most common), knee hyperextension, severe external rotation of the tibia while the knee is partially flexed and knee dislocation. Most commonly these injuries occur in the setting of athletic trauma, motor vehicle accident or falls. According to the literature, 13% to 25.7% of PLC injuries occur in isolation, and the majority of injuries occur in combination with other ligaments; the most commonly associated injuries are concomitant tears of the PCL, followed by ACL. , , Other injuries that are associated with PLC tears are fibular head fractures, biceps femoris tendon avulsion, lateral capsular and ITB avulsions and common peroneal nerve injury, particularly in the setting of knee dislocations.
According to a systematic review that examined 134 acute PLC injuries, 70.9% had concomitant ACL and PCL injuries, 14.2% had ACL injuries, 6.7% had PCL injuries and 8.2% were isolated PLC injuries. An additional systematic review that examined concomitant injuries associated with chronic PLC injuries found that 5.7% had combined ACL and PCL injuries, 59.6% had PCL injuries, 23.6% had ACL injuries and 11.7% were isolated PLC injuries.
Presentation of Injury
Patients with acute PLC injuries most commonly present with pain over the posterolateral aspect of the knee, perceived side-to-side instability near extension, posterolateral rotary instability, difficulty walking on uneven ground, ecchymosis and swelling and foot drop. Chronic injuries present with instability with side-to-side activities, an inadvertent hyperextension or a varus thrust gait, difficulty maintaining full extension and limited ability to resume sports. The literature indicates involvement of the peroneal nerve in 16.7% of patients with isolated PLC injuries and 13% to 16% in combined PLC–cruciate ligament injuries. , , Localised swelling and pain can limit the diagnostic capabilities of the clinical examination. Further, these injuries have classically been overlooked or misdiagnosed on initial presentation; most commonly they are misdiagnosed as lateral meniscus tears or PCL injuries.
Physical Examination
On presentation of a possible PLC injury, a thorough physical examination should include the Lachman test, pivot shift test, dial test at 30 degrees and 90 degrees, posterolateral drawer test with knee at 90 degrees of flexion and 30 degrees of external rotation, increased heel height distances compared with the contralateral knee, and varus stress examination at 0 degrees and 30 degrees. When the presentation is suspicious for a multiligament knee injury, it is essential to also perform the external rotation recurvatum test (measured via heel height differences in centimetres) and the reverse pivot shift test. , ,
The varus stress test should optimally be performed at 20 to 30 degrees of flexion and serves as the major diagnostic test for posterolateral instability. The examiner should place the fingers over the lateral joint line while the distal femur is stabilised. A varus stress is then applied to the knee while the examiner is holding the patient’s foot and ankle, using the fingers to assess the amount of joint gapping. Just like the medial side of the knee, it is important not to grab the distal tibia when applying this test and to apply the stress through the foot and ankle so one can determine the amount of increased rotational instability and determine the true amount of lateral compartment gapping. A positive varus stress test at 0 degrees of knee flexion is usually indicative of a severe PLC injury, most commonly the FCL, the meniscotibial portion of the midthird lateral capsular ligament, the PLT and possibly the superficial layer of the ITB. Moreover, when this test is positive, there is likely a component of cruciate ligament injury because the stabilisation effects of both the PCL and ACL significantly oppose varus stress in this position.
The external recurvatum test is performed by lifting a supine patient’s great toe and observing the relative amount of genu recurvatum present. The amount of relative knee hyperextension present should be compared with that in the contralateral normal knee and measured with a goniometer or heel-height differences. In addition to an increase in knee recurvatum in the setting of a PLC injury, studies have reported an increase in external rotation and genu varus during this physical examination. The literature demonstrates that increased recurvatum of 2.5 cm suggests a combined PLC or FCL and ACL injury.
The reverse pivot shift test is performed with the knee flexed to approximately 80 to 90 degrees, with a valgus and external rotational force applied. In this position a positive test would be identified if the tibia became subluxed posterolateral. The knee is then extended; if the tibia is posterolaterally subluxed, the ITB will reduce it as it goes from a flexor to extender of the knee and a visible reduction of the tibia on the femur can occur. Of note, a positive reverse pivot shift test can be found in 35% of normal physiologically lax knees, highlighting the importance of performing the clinical knee examination on the contralateral knee for comparison and also considering the physical examination finding in the setting of the injury as a whole.
Less commonly used in the clinical examination for diagnosis of a PLC injury is the reported standing apprehension test. The patient stands with weight on the injured leg and knee slightly flexed, while the clinician applies a medially direct force to the anterolateral portion of the femoral condyle. A positive test is indicated by the patient feeling a giving-way sensation. ,
The physical examination for timely diagnosis of PLC injuries is a crucial area for further improvement. Properly identifying these injuries at the initial visit will result in significant improvement in surgical outcomes.
Imaging
Acute PLC injuries are difficult to identify on standard anteroposterior and bent knee patellofemoral (sunrise) radiographs. Consequently, it is essential to obtain bilateral varus stress radiographs for a reliable diagnosis. These images must be analysed for the objective amount of side-to-side difference in lateral compartment gapping. The literature indicates that varus stress radiographs are both a reliable and reproducible method to evaluate for the presence and the severity of PLC lesions.
Varus stress radiographs should be performed with the knee at 20 degrees flexion. Lateral compartment gapping is determined by measuring the shortest distance between the subchondral bone surface of the most distal aspect of the lateral femoral condyle and the corresponding lateral tibial plateau ( Fig. 9.8 ). LaPrade et al. have reported that an isolated, complete FCL tear can be identified by a spatial difference of 2.7 to 4.0 mm, whereas a side-to-side difference of greater than 4 mm corresponds to an associated grade 3 PLC injury. It should be noted that a 2016 biomechanical study concluded that an isolated FCL tear could be identified by a side-to-side increase of 1.99 mm on varus stress radiographs and 2.71 mm increase in an ACL-deficient knee.

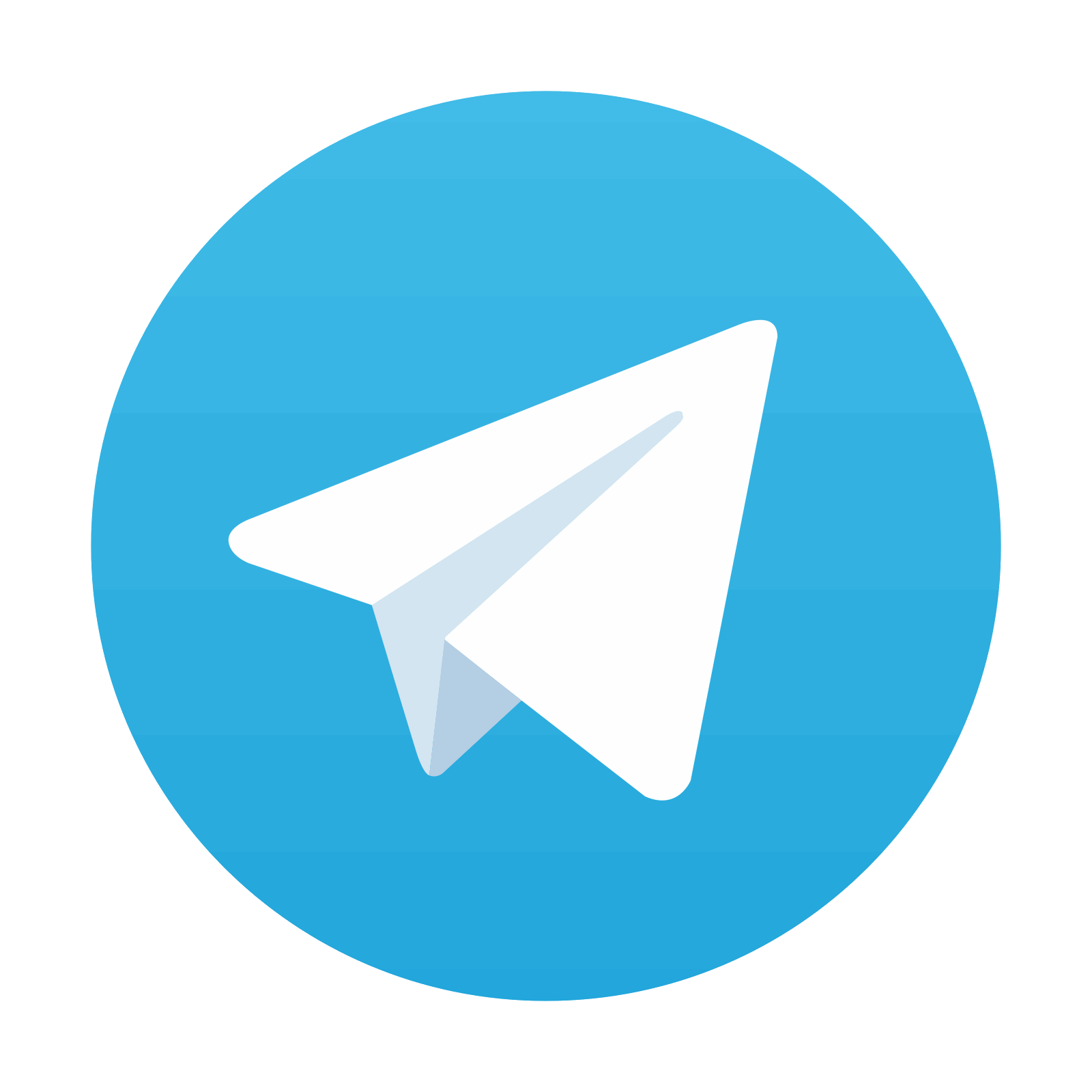
Stay updated, free articles. Join our Telegram channel

Full access? Get Clinical Tree
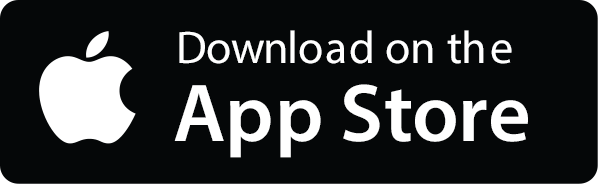
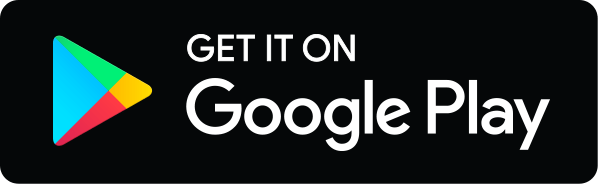
