Chapter objectives
- •
Explain the fundamental basis of plyometric training, its origins, and its applications.
- •
Describe the mechanical and neuromuscular physiologic processes involved in the stretch-shortening cycle and how it applies to plyometrics.
- •
Describe the important clinical considerations surrounding the appropriate use of plyometrics in the orthopedic and sports medicine rehabilitation setting.
- •
Describe and apply important fundamentals for the use of plyometrics as a rehabilitation tool, including pretraining assessment, application of exercise prescription principles, and injury prevention.
- •
Design a basic plyometric training program at low-, medium-, and high-intensity levels.
- •
Use a variety of upper and lower extremity plyometric exercises as a part of the rehabilitation process.
Speed and strength are integral components of sports that are found to varying degrees in virtually all athletic movements. Simply stated, the combination of speed and strength is power. For many years, coaches and athletes have sought to improve power to enhance performance. Recently, rehabilitation specialists have implemented techniques to prevent injuries and improve power to optimize postsurgical and postinjury outcomes. Throughout the twentieth century and no doubt long before that, jumping, bounding, and hopping exercises have been used in various ways to enhance athletic performance. In recent years this distinct method of training for power and explosiveness has been termed plyometrics . Plyometrics is a form of strength training designed to develop explosive power in athletics. Plyometric exercises stress the rapid generation of (maximal) force, primarily during the eccentric (lengthening) phase of muscle action, and speeding the transition between the eccentric and concentric (shortening) phases. This rapid deceleration-acceleration movement produces an explosive reaction that increases both speed and power. The ultimate aim is to increase muscle performance to absorb and move an applied load throughout its functional range of motion and allow an athlete to translate strength into power more efficiently. The concentric phase of muscle activity during plyometric training has been estimated to result in 18% to 20% more force than a concentric contraction from a resting position.
Although the actual term plyometrics is relatively new, this particular form of training has been in existence for quite some time. Translated from its Greek origins, it literally means to increase measurement. Many studies have shown effective, measurable increases in power and jumping ability via plyometric training programs. However, whether improvements in strength and power with plyometric training directly translate into improvements in functional outcome measures in rehabilitation may be questioned in some cases. Plyometric training is often considered the missing link between weight training (strength) and athletic performance (power), with particular emphasis on the speed of activity. Plyometric training was first developed in the Soviet Union for its intense and very effective athletic development program during the 1960s. It came to the attention of the West during the 1970s and, by about 1980, had become a valuable tool in major athletic programs. The term was first applied in 1975 by American track and field coach Fred Wilt to describe the training methods used by Eastern European athletes at the time, which supposedly was the reason for differences between the performance of Eastern and Western athletes. During the 1980s, Donald Chu published the first articles on plyometric training methods in this country, and he has been a leader in this area ever since. In the early 1990s, George Davies and Kevin Wilk introduced plyometrics into rehabilitation programs.
In the early years of plyometric training, most drills focused on developing jumping ability. More recently, some drills have been designed to develop lateral movement qualities and others to improve upper body power, but plyometrics seems to have been focused traditionally on enhancing lower body power. In the past 10 years, plyometrics has been used not only for the lower extremity to increase strength and conditioning but also for the upper extremity as a rehabilitation tool and as part of injury prevention programs.
General theory
The premise behind plyometric training is that the maximum force that a muscle can develop is attained during a rapid eccentric contraction. However, it should be recognized that muscles seldom perform one type of contraction in isolation during athletic movements. When a concentric contraction occurs (muscle shortening) immediately after an eccentric contraction (muscle lengthening), the force generated can be dramatically increased. If a muscle is stretched, much of the energy required to stretch it is lost as heat, but some of this energy can be stored by the elastic components of the muscle. This stored energy is available to the muscle only during a subsequent contraction. It is important to realize that this energy boost is lost if the eccentric contraction is not followed immediately by a concentric effort. To express this greater force the muscle must contract within the shortest time possible. This whole process is usually called the stretch-shortening cycle (SSC) and is the underlying mechanism of plyometric training. Theoretically, the SSC increases power production based on two proposed models: (1) mechanical and (2) neurophysiologic. The mechanical model describes the series elastic component of the musculotendinous unit as the key element of plyometric exercise, whereas the neurophysiologic model involves potentiation of concentric muscle action by use of the stretch reflex. The reflex component of plyometric exercise is based on proprioception provided by the muscle spindles during the stretching (eccentric) action. Even though these two models can be viewed as separate descriptions of plyometric theory, they are in fact tightly interwoven in explaining the mechanisms involved in plyometric training.
Clinical relevancy
Overall, the final phase of rehabilitating an injured athlete introduces functional, sport-specific components of athletic performance. Depending on the athlete, this will typically involve increasing metabolic capacity, strength, power, speed, and agility. It is during this final phase that the use of plyometrics is most appropriate. In earlier phases of rehabilitation, the clinician has presumably addressed joint and soft tissue mobility, range of motion, flexibility, biomechanics, balance, proprioception/kinesthesia, endurance, and strength. The challenge of the final, sport-specific phase is designing rehabilitation progression that maximizes neuromuscular and skeletal adaptations without adversely affecting biologic healing. Sport-specific training means that the movement that the patient performs should match, as closely as possible, the movements encountered during competition without jeopardizing the patient’s health status. If the patient is a basketball player practicing for rebounding or a volleyball player interested in increasing vertical jump height (VJH), drop jumping or box jumping may be the right exercise. If, however, the patient is a quarterback 6 months after a Bankart repair who is trying to increase throwing velocity, upper body plyometrics may be far more appropriate. In the volleyball and quarterback cases, though, the strength and power generated from both the lower and upper extremities might be considered.
Of course, plyometric training is not appropriate for all patients. For instance, an injured gymnast who is trying to develop the shoulder strength to maintain static, slow-moving positions on the balance beam may not require any plyometric drills. In contrast, one study showed that plyometric training significantly improved VJH in elite volleyball players. Plyometric training is not generally a favored choice of exercise for increasing muscle mass or muscular endurance. Therefore, plyometric training is probably not appropriate for muscles that are predominately type I muscle fibers or that act as stabilizers. In fact, unless the athlete participates in a sport that requires explosive movements (e.g., volleyball, baseball pitching, sprinting, basketball, high jump), there is no compelling reason to introduce plyometrics into the rehabilitation program.
Applied anatomy/physiology and the biomechanics of plyometrics
Similar to other modes of therapeutic exercise, safe and effective interventions using plyometrics require that clinicians be familiar with applied neuromuscular physiologic principles. The use of plyometrics as a training method is primarily based on two fundamental dynamic qualities of muscle tissue: elasticity and contractility. The capacity of working muscles to generate greater (or maximal) force in a minimal amount of time depends on these tissue qualities, along with neuromuscular control, strength, and flexibility. In fact, most of the early physiologic research relevant to plyometrics was described as a muscle action termed the stretch-shortening cycle . Currently, the SSC is the physiologic theory that forms the basis of plyometrics.
Stretch-Shortening Cycle
The SSC can be defined as a phenomenon whereby the natural pattern of lengthening of active muscle produces energy that is stored in the musculotendinous unit for later use in a subsequent shortening, or concentric, contraction of the SSC. It is this eccentric-concentric coupling that forms the basis of the SSC. In an often-referenced study, Cavagna established that concentric muscle performance in an SSC is enhanced greater than that in a pure concentric-only action. In practical terms, plyometrics involves high-velocity eccentric contraction or prestretching of a muscle before an immediate reciprocal concentric contraction of that same muscle (group). The eccentric contraction stores energy that can be used to maximize the amount of power produced during concentric contraction of that muscle or muscle group. This storage of elastic energy in musculotendinous tissues contributes to the increased force produced in the subsequent concentric contraction phase and increased efficiency of movement. Muscles and tendons have an intrinsic stiffness that resists stretch and then reciprocates with a muscle contraction stimulated by the stretch reflex loop. Though an oversimplification, this phenomenon can be visualized as the action of a spring. Biomechanically, one can relate the SSC to the simple action of a person attempting to improve VJH by instinctively performing a partial squat before jumping in place. The simple act of walking or running uses the SSC with each stride, which begins with the loading response through an eccentric contraction of the quadriceps, soleus, and gastrocnemius muscles, followed by a concentric push-off action. Hence, the SSC of plyometric exercise is a natural motion, given the mechanical properties of the musculoskeletal system. The SSC has been studied by many investigators, and it is generally agreed that several important factors of neuromuscular anatomy and physiology should be considered: serial elastic components of muscles and tendons, proprioception, and gross musculotendinous architecture. The eccentric-concentric coupling of the SSC stimulates the proprioceptors of the muscle spindle, Golgi tendon organs (GTOs), and ligament receptors to facilitate recruitment of the motor units required to maximize the concentric power generated during plyometric activity ( Box 26-1 ).
Serial elastic and histologic components of muscle and tendons, i.e., myosin and actin sliding filaments/cross-bridge attachments, sarcomeres, fiber types, and metabolic properties
Proprioception mediated through muscle spindles and Golgi tendon organs and their contribution to stretch (or myotonic) reflex loops
Gross musculotendinous architecture, tendon stiffness, and elasticity
Absence or presence of pathology or degenerative changes in bone, ligaments, and musculotendinous units
Histologic Considerations in Skeletal Muscle
Histologically, the level of the fascicles is a fundamental component of the serial elasticity of muscle. Although much research has been conducted on the dynamics of the SSC at the histologic level, the behavior of human skeletal muscle during SSC exercises has not been directly investigated in vivo. It has been well documented in the literature that activated muscle stretched before shortening performs more forcefully. However, the exact mechanisms that mediate the stretch-enhanced performance have been a source of controversy. Kubo et al examined changes in fascicle length and tendon structure in humans during SSC exercises involving the gastrocnemius muscle at both fast and slow speeds with real-time ultrasonography. They found that both fascicle and tendon structures are lengthened at the dorsiflexion phase and shortened at the plantar flexion phase. The stretching ability depends on many factors, but the rate and magnitude of the stretch applied contribute to how much force a muscle can generate.
Though perhaps not as critical in terms of stretching dynamics, the fiber types used for plyometrics are worth reviewing. It is well documented that type II, or fast-twitch, fibers are capable of generating more force than type I, or slow-twitch, fibers can. This is primarily a function of the increased cross-sectional area, larger motor units, and high glycolytic capacity associated with type II fibers. The amount of force necessary during the eccentric and subsequent explosive concentric phases of SSC exercise necessitates the recruitment of type II fibers. It should also be noted that type II fibers are also readily fatigable. Although a detailed discussion of the metabolic properties of muscle fiber types is beyond the scope of this chapter, it is important for the clinician to be aware of the metabolic systems that contribute to optimal performance of muscle tissues during plyometric exercise. Type II fibers are classified further into at least two basic types: type IIa and type IIb. Fast-twitch type IIb fibers are those that rely primarily on the phosphate and glycolytic energy systems and are termed fast glycolytic fibers . Type IIa fibers are more like a hybrid between the slow oxidative type I fibers and the type IIb fibers and are termed fast glycolytic-oxidative fibers . Plyometric exercise should focus on adaptation of these fibers to overload with anaerobic training while still taking into consideration those type IIa fibers that use both the oxidative and glycolytic energy systems. Both the force generation and endurance qualities necessary in functional athletic activity require that the athlete develop both classes of type II fibers. Practically, consider the metabolic demands imposed on a basketball player jumping for consecutive rebounds over a 5-second period, and then consider that same task early and very late in the same game.
Neuromuscular Physiology: Muscle Spindles and Golgi Tendon Organs
Muscle spindles function primarily as stretch receptors, as observed clinically in the performance of standard reflex testing (e.g., the knee jerk). When the patellar tendon is tapped with a reflex hammer, the muscle spindles are stimulated, which causes an immediate concentric contraction of the quadriceps muscle group. This minimal latency time between the quick stretch and subsequent contraction is mediated at the level of the spinal cord as a monosynaptic reflex. Muscle spindles are sensitive to changes in velocity and are innervated by type 1a nerve fibers. These afferent nerve fibers conduct the impulse directly to the spinal cord, where they are immediately conducted via interneurons to alpha motor neurons, which stimulate muscle contraction. The brain is not involved in this spinal reflex loop, contributing to the speed at which the stretch-contraction cycle occurs.
Muscle spindles are located within extrafusal (skeletal) muscle fibers and consist of connective tissue surrounding intrafusal fibers in a capsular structure. Muscle spindles are innervated by myelinated afferent nerve fibers, which enter the capsule of and spiral around the intrafusal fibers. Based on the architecture of the muscle spindle, stretching of the skeletal (extrafusal) fibers also stretches the intrafusal fibers. This stretch increases the firing rate of the afferent fibers innervating the intrafusal fibers, thus “loading” the muscle spindle. When the stretch is released or lessened, the firing rate diminishes. Both primary and secondary afferent fibers are present, and these fibers contribute to the ability of the spindle to detect small changes in length. Thus, the muscle spindle is sensitive to changes in muscle length, as well as to the speed and magnitude of the stretch.
Although the muscle spindle reacts to stretch, it does not simply “turn off” when the muscle is no longer stretched; the fibers continue to send messages when the muscle has begun to concentrically contract and shorten. The central nervous system (CNS) regulates the loading through alpha motor neurons, which modulate spindle activity and thereby make the transition from stretch to contraction smoother. This modulation contributes to muscle tone and thus to the intrinsic stiffness of the muscle. Therefore, the muscle tends to act as a spring that enables the SSC to produce force with precision.
Although the muscle spindle is sensitive to stretch, the GTOs provide complementary information to the CNS about muscle activity. Specifically, length and the degree of tension are monitored by GTOs. GTOs are encapsulated collagen structures typically located at the musculotendinous junction. Each GTO is innervated by nerve fibers that wrap around the collagen bundles. As the collagen bundles are stretched, they straighten and the nerve fibers fire more rapidly. GTOs are sensitive to small changes in muscle tension. Because GTOs surround collagen and not extrafusal muscle fibers, they are not as sensitive to stretch since collagen has a stiffer molecular structure than muscle fiber does. Thus, most of the stretch is absorbed by the muscle fibers and the muscle spindles. This makes GTOs more sensitive to active muscle contraction that stresses the musculotendinous junction to a greater degree.
Gross Musculotendinous Structure
On a gross anatomic and clinical level, muscles and tendons resist stretch as force increases. Muscle stiffness can be defined as change in force over change in muscle length. This inherent stiffness is the resistance to stretch by the fibers of the active muscle and tendons before the changes in activation modulated by the muscle spindles and GTOs described earlier occur. Benn et al described this property of stiffness as the stretch work used in completion of the SSC. Several authors have studied the relationship between SSC performance and muscle stiffness. Goslow et al found that cat tendons with a high degree of stiffness may transfer energy more rapidly to attached muscles, thereby resulting in earlier activation of the stretch reflex and thus more rapid contraction of the muscle. Wilson et al concluded that in humans, a stiffer musculotendinous unit may result in an increased rate of concentric contraction and more rapid transmission of force to the working limbs. In another study these authors concluded that decreased musculotendinous stiffness actually enhances SSC performance in a bench press exercise because more elastic energy can be stored in a less stiff musculotendinous unit. Other studies have concluded that increased musculotendinous stiffness may be more important than the ability to store more elastic energy in terms of enhancing SSC performance in activities such as sprinting. Given these equivocal results, the principle of specificity in terms of exercise mode and the muscles involved may be an important distinction in determining optimal musculotendinous stiffness.
To this point, one can see that the histologic structure of muscle and tendons can both enhance and hinder movement and force-generating capacity. Although the inherent elastic components of the musculotendinous unit can store energy for use in generating force and powerful movement, gross structural aspects may be present that limit the ability of a muscle to maximize the SSC. Neural factors and recruitment of fibers, as well as the metabolic capacities of muscle and the appropriate exercise conditioning, all have an impact on performance.
Finally, Brownstein and Bronner formulated a classification system based on three types of musculotendinous units ( Table 26-1 ). Their theory centers around gross muscle structure (i.e., length of the muscles and tendons).
Type | Example | Characteristics |
---|---|---|
Muscles with long fascicles and relatively short tendons | Gluteus maximus | This type of muscle is usually located proximally and tends to be large in size. These muscles are typically powerful movers with a large muscle fiber cross-sectional area. They are capable of moving the limb through a wide range of motion and can absorb a significant amount of energy. For the gluteus maximus, stepping off a box and into a squat position smoothly is partially a function of eccentric contracting (lengthening) of the gluteal muscles. |
Muscles with long, thick, inelastic tendons | Gastrocnemius (although this muscle type is generally located more proximally) | The tendons have a high degree of stiffness and provide strong control of the distal segment. |
Muscles with short fascicles and long, slender tendons | Tibialis anterior | These muscles can store large amounts of elastic energy when stretched rapidly because they are less stiff. Such muscles shorten very little and are more efficient on the length-tension and force-velocity curves. They tend to have more slow-twitch, highly oxidative fibers and are therefore metabolically efficient. |
Fundamentals of Plyometrics
Plyometrics is defined by the SSC and is an inherent part of the functional aspects of athletic movement. The primary basis of plyometrics is to use both the serial elastic (mechanical) and neurophysiologic components described earlier to combine speed and strength in the production of power. Plyometrics has been described as stretch-shortening drills or reactive neuromuscular training. There are basically three phases of a plyometric exercise and the SSC.
The eccentric phase (sometimes called the preloading or setting phase) refers to the early moments in the movement in which the muscle spindles are loaded and stretched during an eccentric contraction, such as stepping from a box onto the ground and squatting as one lands to absorb the ground reaction force. This is when storing of elastic energy takes place. The time interval for this phase depends on how much stretch facilitation is desired for the subsequent phases. For rehabilitation, this phase will most likely be dictated by the range of motion and amount of shock absorption that the athlete’s body is capable of withstanding or the amount of eccentric loading that the agonist musculature and passive restraints (e.g., ligaments) can tolerate. From a tissue protection standpoint, this is why so much clinical research has focused on the landing phase of athletic movement. In this area, the primary concern is the potentially poor neuromuscular strategies used during athletic movement. Numerous studies on prevention of injury to the anterior cruciate ligament (ACL) and rehabilitation are concerned with the biomechanics of the landing, or eccentric, phase. ,
The amortization phase refers to the time between the end of the eccentric contraction and initiation of the concentric, explosive reaction force that accelerates the body or working limb in the desired direction. This phase should be as short as possible because with a long interval there is a risk of losing much of the elastic energy as heat within the muscle. Successful performance of a plyometric drill depends on the ability of the musculotendinous unit to effectively absorb and exploit the stored elastic energy. The rapid stretching (eccentric loading) must be immediately followed by a rapid, explosive concentric contraction to maximize the force generated. The quicker that an athlete can overcome the yielding eccentric force and produce a concentric contraction, the more power that can be produced. The concentric phase represents the cumulative effect of the eccentric and amortization phases through a powerful concentric contraction ( Box 26-2 ).
Eccentric phase
Preloading or setting period
Muscle spindles “loaded” via stretch/eccentric contraction of agonists
Amortization phase
Interval between the eccentric and concentric phases
Should be short enough to fully use the elastic energy stored in the stretched muscle-tendon complex
Concentric phase
Concentric muscle contraction of agonists
Maximal power generation with explosive movement
It is important to note that many clinicians and strength/conditioning coaches would suggest that most plyometric and athletic injuries occur on landing and not during the jumping (concentric) or takeoff phase. Although this might be true, especially for noncontact ACL injuries, for example, the clinician should nonetheless be cognizant of the potential for muscle, tendon, and soft tissue injury during takeoff when designing plyometric programs for rehabilitation.
Clinical considerations for plyometrics
Although all athletes may not necessarily need to produce explosive strength to excel at a given sport, most athletes undergoing rehabilitation need to regain strength and proprioception after an injury. Even though plyometrics has traditionally been used in the realm of sports that require strength, speed, and power, such as sprinting and other track and field events, all competitive athletes may be able to reduce the risk for future injury by maximizing their dynamic restraint system. Joint stability depends on both passive and dynamic restraint structures. Passive structures, such as the arthrokinematics of articulating surfaces, ligaments, joint capsule, menisci, and labrum, provide support to the musculoskeletal components. The dynamic restraints that provide joint stability include the muscles and neural controls associated with movement. It is often the passive restraints that are damaged in sports and daily activities involving high-velocity movements and perturbations in dynamic balance. Unfortunately, even though healing of passive restraints can be addressed through physical therapy, these structures are not easily modified by active conservative treatment on the part of the athlete because the healing process is more passive (modalities, passive range of motion, and manual therapy). Thus, protective and rehabilitative efforts have focused primarily on modifying the dynamic neuromuscular elements, including joint capsule mechanoreceptors. To this end, proprioceptive training, active range of motion, flexibility, and strengthening interventions are typically used to empower the patient to maximize the ability of the dynamic restraints to contribute to stability and prevention of injury. Any displacement that occurs too quickly for reflex reactions to protect a joint requires that the mechanical properties of the musculotendinous unit resist the displacement. Active muscle response to any perturbation that might compromise joint stability is thus an important consideration in rehabilitation for prevention and athletic performance. Plyometric exercises can assist the clinician in addressing these biomechanical demands.
Generally, plyometrics involves ballistic and repetitive movements. Although these exercises and drills are designed to optimize the SSC and improve athletic performance, clinicians need to be cautious in deciding when a patient is ready for safe and effective use of plyometrics. Specifically, a patient must have achieved a certain level of range of motion, neuromuscular control, proprioception, balance, strength, and flexibility before undertaking plyometric exercises. The evaluation process should always consider the treatment goals established for a given patient at the onset of therapy ( Box 26-3 ).
Sufficient resolution of pain to participate in higher-level exercises and activities
No inflammation or joint effusion before or after exercise
Normal range of motion with respect to the uninvolved side
Normal joint alignment and mobility
Soft tissue flexibility, including both contractile and noncontractile structures, within normal limits
Adequate strength for full weight-bearing activity on the involved limb unilaterally if the lower extremity or strength for functional use of the upper extremity, including full weight bearing on the upper extremities if appropriate for function
Normal reflexes
Normal motor control
Balance and proprioception/kinesthetic sense within functional limits of the uninvolved side (e.g., consider the concept of time to stabilization)
Applications in Rehabilitation
Plyometrics is used widely in a less intense manner for the rehabilitation of many athletic injuries. In contrast, some patients progress sufficiently to allow the use of medium- and high-intensity plyometrics before discharge from formal rehabilitation. In addition to increasing conditioning, plyometrics can facilitate improved functional motor patterns, reflexes, and proprioception, all of which are crucial in the attempt to return an athlete to competition ( Box 26-4 ).
Improved proprioception during dynamic movement
Improved speed, strength, and power
Improved reaction time
Increased bone mineral density
In the past 15 years or so, the use of lower extremity plyometrics has received increased attention as an adjunctive modality for the prevention of noncontact ACL injuries. Although research in this area might be considered somewhat limited, it seems that plyometric-oriented exercise programming is an important component in reducing ACL injuries in females. A growing body of evidence is linking ACL injuries to poor neuromuscular control in injured athletes. It is possible that some athletes may have poor technique during jumping, landing, stopping, or turning that may lead to injury. Neuromuscular control must be developed in all three planes of motion—frontal, sagittal, and transverse—to decrease stress on the ACL and move it to the muscles and tendons. Proper plyometric training can decrease the force and torque placed on the knee. Proper technique increases the load placed on the muscles and tendons and removes it from the joint and ligaments. The principles of plyometric training—functional motor patterns, reflexes, and proprioception—are instrumental in the prevention of knee injuries. These same principles can be applied to an upper extremity that is functionally weak and perhaps unstable for competitive athletic loads or has lost position sense.
In fact, Hewett et al examined the effects of a plyometric training program on landing mechanics and leg strength in female athletes involved in jumping sports. The plyometric program was designed to decrease landing force by helping the athletes improve neuromuscular control over the lower extremity during landing. It was also designed to increase VJH. The authors reported that peak landing force during a volleyball block jump decreased by 22%. Horizontal force acting on the knee during landing was reduced by approximately 50%. Hamstring-quadriceps peak torque-strength ratios increased 26% in the nondominant leg and 13% in the dominant leg. Hamstring power increased 44% in the dominant leg and 21% in the nondominant leg. Mean vertical jump increased by 10% overall. This study, as well as others since, has linked the preventive aspect of plyometrics and therapeutic exercise. It suggests that a properly performed plyometric training protocol may help prevent knee injury in female athletes involved in jumping sports, such as volleyball, by increasing knee stabilization during landing and teaching athletes muscular control. Plyometrics may also help correct torque imbalances between the hamstrings and quadriceps and can help increase VJH.
Hewett et al prospectively monitored two groups of female athletes, one trained before sports participation and the other untrained, and a group of untrained male athletes throughout the high school soccer, volleyball, and basketball seasons. Fourteen serious knee injuries occurred in the 1263 athletes tracked through the study. Ten of 463 untrained female athletes sustained serious knee injuries (eight noncontact), 2 of 366 trained female athletes sustained serious knee injuries (zero noncontact), and 2 of 434 male athletes sustained serious knee injuries (one noncontact). The untrained female athletes had a 3.6-fold higher incidence of knee injury than the trained female athletes did and a 4.8-fold higher incidence than the male athletes did. The incidence of knee injury in the trained female athletes was not significantly different from that in the untrained male athletes. A significant difference was seen in the incidence of noncontact injuries between the female groups. In this prospective study a decreased incidence of knee injury was demonstrated in female athletes after a specific plyometric training program.
Although the jumping aspect of plyometric training is important for conditioning, it is the landing of each jump that is vital in the theoretic prevention of knee and lower extremity injuries. Obviously, rehabilitation and conditioning that focus on landing technique only do not involve the entire SSC since there is no concentric phase. Nonetheless, good technique on landing is crucial to avoid a knee going into hyperextension and external rotation, the point of no return. A key concept is that the athlete should land softly and quietly while using the knees and hips as shock absorbers. Another is that the shoulders should be over the knees when the athlete lands. During plyometric training at any intensity, the athlete should be constantly reminded to land softly. Another key concept for prevention of injury and conditioning is that hyperextension should be avoided during all activities such as turning, landing, stopping, cutting, or slowing down. When landing from a depth jump exercise the athlete should “stick” and hold the landing. This is accomplished when balance is maintained for several seconds after landing with no additional steps taken and minimal trunk sway in any direction. Ideally, no foot movement should occur after landing ( Box 26-5 ).
“Stick” the landing with minimal trunk or hip-knee sway
Hold the landing for 2 to 5 seconds (as appropriate)
Land softly and quietly
Keep the shoulders over the knees when landing and do not allow the knees to shift anterior to the toes in the sagittal plane
Avoid hyperextension during all activities
Clearly, proper execution of plyometric exercises requires dynamic stabilization during the amortization phase and landing, as well as during the concentric phase. Some authors have suggested that the components of plyometric landing be measured by time to stabilization (TTS). The intensity of plyometrics, especially jumping modalities, has been studied via electromyographic (EMG), kinematic, and kinetic analysis, but the specific characteristics of many plyometric exercises have not. Ebben et al demonstrated that TTS can be used for progression of plyometric intensity and that it has moderate to high reliability for jumping conditions in both male and female college-aged athletes. Although the average clinician does not have access to the force platform equipment to precisely measure TTS, the concept is important in evaluating a patient’s ability to perform and progress through a plyometric program. Briefly, TTS has been used to evaluate ankle stability under varying conditions, including a jump task from bilateral takeoff to single-leg landing, as well as step-down from a box 20 cm high. An athlete’s or any patient’s ability to perform and progress through plyometric exercises should be evaluated with consideration of these concepts.
With the exception of research studying ACL injuries and prevention, there is a relative dearth of controlled studies in the rehabilitation literature on plyometrics. In contrast, many studies in the strengthening and conditioning literature have examined the effectiveness of plyometrics in improving parameters of athletic performance, especially power and VJH. Numerous studies have shown improvements in VJH, but a few studies have failed to demonstrate significant improvement in VJH. Nonetheless, in terms of rehabilitation in particular, relatively little information is available on the efficacy and evidence-based guidelines for plyometric rehabilitation. Clinicians must therefore combine knowledge of basic science, the available studies on ACL rehabilitation, and outcomes of plyometrics in healthy subjects to optimize results in the athletic population.
As most clinicians would suspect, the literature suggests that the effects of plyometric training in healthy subjects differ depending on training level, gender, age, sport activity, and familiarity with plyometric training. Even in healthy athletes it is generally believed that an individual should have a reasonable amount of flexibility, strength, and agility before starting a plyometric training program. Though perhaps not as important as for other exercise modes used in the athletic training room or clinic, it is critical that specific assessment and testing be conducted before the use of plyometrics. Plyometrics is demanding physically and requires that the individual concentrate on controlling movements both statically and dynamically.
According to some authors, the risk for injury from plyometrics is low, but few studies have actually addressed injury rates associated with plyometric training in healthy individuals. Even though no studies of injury rates have been performed in patients undergoing rehabilitation and using plyometrics, some studies have examined fatigue, inflammation, muscle damage, and recovery times in healthy subjects after SSC exercise. Gollhofer et al found that repeated SSC muscle activity induces fatigue effects associated with a decrease in neural input to the muscle and reduced overall muscle performance. It has been suggested that during fatigue from SSC exercise, the repeated stretch loads might reduce the reflex contribution to the SSC. Avela and Komi studied a group of experienced endurance runners and concluded that fatiguing SSC exercise reduces stretch-reflex sensitivity, which was associated with decreased muscle stiffness. The authors postulated that this would impair the athlete’s ability to use the stored elastic energy in the muscle-tendon complex. As discussed previously, plyometric training has a significant eccentric component, and the muscle-damaging effect of eccentric exercise modalities has been well established in the litereature. A recent study demonstrated acute inflammatory responses and reduced jump performance after an intense bout of plyometric training. Other studies have shown evidence of muscle damage by studying serum markers for creatine kinase and collagen breakdown. Overall, some research suggests that plyometric exercise may cause more delayed-onset muscle damage than concentric exercise does, but not as much as eccentric exercise alone does.
Lower extremity plyometric exercises are of particular concern for injury to the feet, ankles, lower part of the legs, knees, hips, and lumbosacral spine. As is the case during most athletic events, injuries are more likely when an individual is fatigued, typically toward the end of an event or exercise session. Sprained ankles and knee injuries are commonly associated with lack of control because of excessive fatigue. Inadequate conditioning, lack of adequate warm-up, poor-quality athletic shoes, inappropriate training surfaces, and low levels of skill may predispose an athlete or patient to injury. Borkowski reported that a preseason plyometric training program in collegiate volleyball players did not cause injuries but actually significantly reduced in-season muscle soreness. Thus, it is clear that proper assessment and testing of athletes before a plyometric program is started and diligent application of the exercise prescription principles of frequency, duration, and intensity are extremely important.
Basic Pretraining Testing
In addition to the physical assessments and strength guidelines mentioned previously, several basic static and dynamic tests can be used to determine a patient’s ability to begin a plyometric training program. Voight and Tippett proposed that an individual be able to perform a 30-second one-leg stance with the eyes open and closed before starting a plyometric program. Involved versus uninvolved legs should be compared. Voight and Draovitch recommended that the stork balance test be performed for 30 seconds and that a single-leg half-squat also be evaluated before any jumping plyometric exercises are begun. Although it is important to consider the athlete’s ability to perform a single repetition of a dynamic movement, such as a single-leg squat, single-leg heel raise, or standard push-up with proper biomechanics, pretraining testing should closely monitor multiple repetitions and sets of exercise to test for local muscle endurance.
The clinician should be creative in using functional testing to determine whether a patient is prepared to begin a plyometric program. Vertical jumps in place and horizontal long jumps on a shock-absorbing surface are two simple tests that may provide feedback about a patient’s status. Particular attention to the involved (injured) side with unilateral testing can prove invaluable in avoiding reinjury or progressing too abruptly by adding a plyometric component to a rehabilitation or training program. Any pain or instability observed during these tests may provide clues to the patient’s tolerance of plyometrics. Having the patient perform step-ups and step-downs from progressively increasing heights will also provide some indication of the patient’s tolerance. Lateral shuffle and carioca (crossover) drills likewise provide the clinician with ways of testing whether an affected lower limb is prepared to handle plyometrics. Obviously, jogging and running are perhaps the first dynamic movements performed to assess the ability of the affected limb to bear full body weight dynamically. Before incorporating any high-intensity, or shock, plyometric drills such as box jumps or depth jumps, the reader is encouraged to consult sources to determine the height of the box that should be used for an individual’s ability. Concerning plyometric training in healthy athletes, five methods are used by various authors to identify the optimal drop height that will increase countermovement jump performance on an individual basis. The two most common methods are the maximum jump height and the reactive strength index. The clinician is reminded that these methods are intended for use in healthy athletes and should be used as a potential guideline; there is no substitute for sound, practical clinical judgment.
Strength and Conditioning Level
In terms of strength, it is generally accepted that a patient should have a sufficient base of strength-training experience. It is important that a sufficient base of strength training may simply be based on movement patterns that are free of dysfunction. For patients with an ankle sprain, perhaps this is simply the ability to perform three sets of 15 repetitions of unilateral heel raises on the involved ankle at full range of motion off the end of a step (with the heels dipping slightly below parallel or to 10° of dorsiflexion). When plyometrics is used for rehabilitation, a great deal of subjective clinical judgment is necessary on the part of the clinician. The reason is that except for perhaps ACL rehabilitation, evidence in the literature supporting the use of various plyometric exercises for rehabilitation is limited. Indeed, it is agreed that plyometric training principles are beneficial for healthy athletes, although there is much room for subjective judgment in terms of how these drills and exercises should progress. Even though plyometrics can be a form of functional training for rehabilitating both the upper and lower extremities, the ballistic nature of most of these exercises makes them inappropriate for the early stages of rehabilitation. One major disadvantage of plyometric training is that joint excursion is difficult to control because of the nature of the activity.
Although it is not necessary or suggested that strength-training programs focus solely on eccentric contractions, it is important that sufficient eccentric strength be established before a plyometric training program is begun, especially for an injured athlete. Studies have shown that force production is increased during eccentric contractions, thus necessitating tolerance of higher loads and preferential recruitment of fast-twitch fibers; in addition, high eccentric loads may reduce neural inhibition and lead to greater generation of concentric force. During most athletic pursuits, as well as activities of daily living, movement in the opposite direction, an eccentric motion, precedes movement in the intended direction. In most movements, the eccentric contraction is responsible for decelerating the moving limb. Similarly, the eccentric phase of the plyometric exercise absorbs the energy by decelerating the limb and allows storage of elastic energy. Thus, the clinician should incorporate eccentric work during repetitions of various therapeutic and functional exercises. Emphasis on the eccentric phase of the motion just before the concentric work more closely matches true human movement patterns. Note that the focus on eccentric contraction is not intended to minimize the importance of training with faster concentric contractions as well.
No discussion of strength would be complete without mention of the need for establishing a strength base by using closed kinetic chain exercises before a plyometric exercises program is begun. Indeed, practically all lower extremity plyometric exercises are of the closed chain variety, like the functional movements that they mimic. In the case of jumping and landing, the entire kinetic chain, from the ankles through the knees and hips, as well as the vertebral column, needs to absorb full body weight and maintain stability. As discussed earlier, the serial elastic component is important, but the muscle synergy and neuromuscular coordination required for smooth landing and subsequent explosive movement during athletic activity and plyometrics are probably better served by enhancing closed chain strength.
Strength and power (speed strength) are intrinsically necessary components of plyometric training. Chu and Cordier recommended the power squat test as a good closed chain exercise to determine whether a patient has an adequate speed-strength base for lower extremity plyometrics. The exercise is performed with 6% of the person’s body weight. Five squat repetitions are done in 5 seconds, and the depth of each repetition should be close to 90° of knee flexion. If the patient cannot perform the exercise in the allotted time with proper technique, the clinician should continue to emphasize strength training and delay initiation of the plyometric program. For the upper extremities, it has been suggested the athlete be able to perform five repetitions of the bench press at 60% of a one-repetition maximum (1RM) in 5 seconds. For strength training to improve vertical leap performance, Weiss et al concluded that training programs to enhance moderately fast squatting power may improve performance as long as body weight, especially body fat, is not increased. In a related finding, McBride et al concluded that training with light-load jump squats results in increased movement velocity capability and that velocity-specific changes in muscle activity may play a role in this adaptation. Guidelines are not as clear for the upper extremity. Anecdotally, we recommend that the patient have full range of motion, rotator cuff strength at least 75% as strong as that on the uninvolved extremity, and greater than grade 4 of 5 for the prime movers on a manual muscle test.
According Potash and Chu, as well as other authors, for shock- and high-intensity lower extremity plyometrics, it is recommended that a healthy athlete have a 1RM squat of 1.5 times body weight. For high-intensity upper extremity plyometrics, the bench press 1RM is suggested to be at least the athlete’s body weight for larger athletes (body weight > 100 kg). For smaller athletes (body weight < 100 kg), it has been suggested that the bench press 1RM be 1.5 times body weight. Another method suggests that an athlete be able to perform five clap push-ups in a row. Clearly, this requirement is not necessary for plyometrics performed in the early and middle stages of rehabilitation, but it underscores the diligence that is important in evaluating a patient before initiation of plyometrics. By definition, plyometric training involves maximal voluntary contractions. Therefore, plyometrics should be incorporated during the end stages of rehabilitation, when the clinician is preparing an athlete for return to sport or for any patient to achieve maximal functional capacity.
The focus on making sure that a patient has the strength foundation necessary to engage in plyometrics should not overshadow the need for proper endurance and conditioning. Though not an endurance activity per se, a plyometric training session does require a measure of glycolytic endurance because anaerobic or strength/power exercise is more likely to increase lactate levels in the muscle, which decreases the pH of the muscle. The process of removing lactic acid and metabolites from muscle tissue (i.e., recovery) is an oxidative process. Thus, an athlete should have a reasonable measure of endurance to safely avoid fatigue and risk for injury during a plyometric training session. A plyometric program uses successive sets/repetitions and rest periods, but as the duration of the routine increases (e.g., to 15 to 30 minutes), the athlete will encounter fatigue. Similar to what occurs during an athletic event, there is a need for anaerobic endurance. Specifically, the athlete encounters short bouts of explosive anaerobic activity, with short rest periods, and then must continue to attempt to achieve that performance level for several minutes or even hours. Take as an example a tennis match or a basketball or football game. The same explosive movements are required at the end of the match or game as are necessary in the beginning of the competition. Gollhofer et al found reduced EMG activity during the eccentric phase of SSC exercise with fatigue in healthy subjects. Similarly, Nicol et al concluded that the EMG response of calf muscles to passive stretch was a smaller after submaximal SSC exercise. Strojnik and Komi also found that fatiguing submaximal SSC exercise on a sledge jump apparatus decreases the contractile characteristics of the quadriceps femoris muscle.
Special Clinical Considerations
Anthropometrics
Simply because a patient is an athlete does not mean that the rehabilitation program should progress to high-intensity or even high-volume plyometrics programming. In general, high-intensity drills and high-volume plyometric programs may not be appropriate for larger athletes (> 100 kg), particularly if the athlete’s body fat level is high. A football lineman, for example, may be at increased risk, especially because his role in competition does not require jumping and leaping movements as much as the role of a running back or wide receiver does. By the same token, an endurance athlete with a low body fat level but low muscle mass might also be at risk for injury with high-impact plyometric exercises. Ugarkovic et al studied anthropometric and strength variables as predictors of jumping performance in elite junior basketball players and concluded that these measures alone are not the best predictors to assess movement performance in homogeneous groups of athletes. They suggest that these factors and especially sport-specific movements and power be used in a comprehensive evaluation. Nonetheless, body structure, body fat measures, and particularly structural abnormalities and previous injuries must be considered. If body fat measurements are not readily available, it is suggested that the body mass index (BMI) be considered as a starting point. The reader is also advised to keep in mind that the BMI is a less appropriate measure in the athletic population because it is not a measure of body fat; that is, it does not take into consideration lean body mass. Vertebral abnormalities, as well as problems with the knees, hips, and ankles, are of special concern for lower extremity drills. Previous shoulder, elbow, wrist, hand, and cervical or thoracic injuries should be considered when contemplating the use of upper extremity plyometrics.
Age and Gender
Pediatric athletes
Prepubescent children (< 12 years) and adolescent athletes (12 to 17 years) represent a special population regarding plyometrics. School-age children play games such as leap frog, hopscotch, and jump rope to their own level of comfort without any formal instruction—these activities are plyometric by their very nature and are perfectly normal for healthy children. Although it is generally considered inappropriate for children younger than 12 to engage in formal plyometric training, any activity that includes jumping activities on safe surfaces is probably healthy for growing bones and muscles as long as overuse injuries are avoided. The fact that many young children (< 12 years) participate in organized sports such as gymnastics, soccer, hockey, basketball, and football speaks to the safety of low-level, informal plyometric activity. In terms of rehabilitation, we have used low-intensity plyometric drills at low volume for gymnasts, dancers, soccer players, swimmers, and other childhood athletes who intend to return to their sports following physical therapy.
In terms of strength and conditioning, properly designed and supervised plyometric training programs have been used in the adolescent population with safety and effective results. Potdevin et al trained pubescent swimmers for 6 weeks with a plyometric program and observed improved swimming performance as a result of improved dive and turn movements. Rubley et al recently conducted a low-frequency, low-impact plyometric program in female soccer players and observed increased lower body power in terms of kicking distance and VJH. Other studies have also used plyometric training in high school basketball players and soccer players.
Weight-bearing exercise with high load intensity is known to have osteogenic effects. Children who participate in activities associated with higher loads have been shown to exhibit higher bone mass than have children who participate in activities with lower loads. Witzke and Snow investigated the effects of 9 months of plyometric jump training on bone mineral content, performance, and balance in adolescent girls. They found that moderate- to high-intensity plyometric training improved trochanteric bone mineral content, leg strength, and balance in these adolescent girls.
In summary, pubescent athletes can engage in low- to medium-intensity plyometrics, although it is critical that they have the strength and coordination to tolerate these drills safely without incurring injuries to the epiphyseal (growth) plates and overuse injuries to tendons and other soft tissue. High-intensity (shock) plyometrics such as box jumps are not generally recommended for adolescents. Although sequential age should be considered in terms of maturity, each child should be evaluated for maturity in terms of strength, flexibility, balance, and coordination. Finally, pediatric participants must be psychologically mature enough to follow the instructions of the supervising clinician or strength and conditioning coach.
Gender
Regarding gender differences, Aura and Komi found that female subjects better use the prestretch phase of SSC at low intensity levels whereas men show greater potentiation of elastic energy at higher prestretch levels. However, males exhibited higher work because of elasticity. They suggested that there may be fundamental differences in neuromuscular function between males and females. In a more recent study, an SSC index derived from upper extremity tests showed significantly higher values in men than in women, although individual differences were more variable in women. The authors concluded that men may have superior ability to use the SSC in the upper extremities. Based on the work of various authors, it is clear that the neuromuscular patterns exhibited by females during jumping tasks make a strong case for the clinician to use diligence in designing and supervising female athletes during both rehabilitation and training programs involving plyometrics.
Masters athletes (middle aged and older)
Generally speaking, middle age begins at 35 years, and most sports organizations offer “masters” athlete categories beginning at approximately 40 years of age. Whether one is working with a weekend warrior or a competitive amateur masters athlete, it is important to evaluate the patient’s functional status and goals for rehabilitation. When one considers the anatomic and physiologic changes that are inherent in the aging athlete, the use of plyometric exercises comes with special clinical considerations. First, a through medical history is critical in determining how previous injuries, surgeries, and medical conditions might have an impact on design of the program. Second, it is important to consider the decrease in muscle fiber cross-sectional area, preferential loss of type II muscle fibers, reduction in the number of motor units, changes in neuromuscular recruitment, and the subsequent reductions in strength and especially power that accompany the aging process. Rates of skeletal muscle protein synthesis and therefore rates of recovery decline with age. In addition to changes in skeletal muscle and muscle strain injuries, the clinician needs to consider the potential for damage to articular cartilage, knee menisci, intervertebral disks, and tendons, which is more likely in this population. As with plyometric programming for any age group, attention to appropriate levels of exercise intensity, frequency, duration, and volume are especially important in older athletes. Again, understanding each patient’s goals in the context of the plyometric program is important. For instance, a 50-year-old man or woman with a history of meniscus injury who would like to improve running performance in a 5-km event might benefit more from hop and bounding-type exercises than from 24-inch box jumps and high-impact plyometrics.
Warm-Up, Stretching, and Flexibility
The importance of warm-up is well documented in the literature, and it is related to many positive effects on athletic performance, including faster relaxation and contraction of both agonist and antagonist muscles, improved rate of force development, and improvement in strength, power, and reaction time. In contrast, stretching has recently come under scrutiny in the literature. Specifically, static stretching has been associated with acute negative effects on muscle power, torque, force, and maximal strength, as well as jump, sprint, and agility performance. Dynamic stretching, however, has not been shown to elicit decrements in performance and has, in fact, been shown to improve running peformance. It is important to also note that a metaanalysis of stretching studies concluded that the evidence is not sufficient to recommend eliminating preexercise stretching and that no studies have examined populations who are at increased risk for injury. Overall, the evidence that warm-up and stretching reduce risk for injury is equivocal because studies often combine warm-up, stretching modes, and prestretching and poststretching practices. Ultimately, it is probably important for athletic activities that require increased range of motion (e.g., high hurdles, gymnastics) or high-intensity SSC movements to include appropriate flexibility regimens. Because of the eccentric, or lengthening, nature of the SSC and the subsequent maximal concentric contraction, it seems important that athletes should possess a level of flexibility conducive to handling the demands of the specific plyometric exercises being performed.
For plyometric warm-up, whole-body movements should be used to raise the heart rate, increase muscle and soft tissue temperature, decrease the viscosity of synovial fluid, and increase overall body temperature enough to generate mild perspiration. The warm-up and stretching should be activity specific and incorporate the dynamic movements associated with that activity. Assuming that no joint structures are limiting range of motion, improved flexibility through appropriate stretching of muscles and soft tissues should aid in the safe performance of demanding plyometric exercises. Prolonged static stretching before and during a plyometric routine may have a negative impact on performance, so this should be considered carefully. Dynamic, ballistic stretching is probably warranted since plyometric activity is ballistic by definition. Additionally, proprioceptive neuromuscular facilitation stretching may be an appropriate adjunct to improvement in flexibility as well. Detailed discussion of stretching and flexibility is beyond the scope of this chapter, and the reader is encouraged to review Chapter 6 .
Balance and Proprioception
Proprioception describes the collection of sensory afferent nerves that enhance awareness of posture, movement, joint position, limb velocity, changes in equilibrium and weight, and resistance of objects in relation to the body. Kinesthesia is also important and represents the ability to perceive the extent, direction, and weight of movement. Conscious and unconscious perception of these factors is critical fore safe and effective return of the athlete to competition. Research has shown that during jumping, the leg extensor muscles are activated before the feet contact the ground. Komi described this phenomenon as the preactivation or preinnervation phase. Melvill-Jones and Watt suggested that this preactivation is mediated by higher CNS processes before the person lands and that the correct timing and sequence of the (eccentric) contractions to absorb the force have been learned from previous experience. According to Avela and Komi, many studies have confirmed this theory, and a clear relationship exists between the duration and amount of preactivation and the height of the drop as a result of the person’s jumping. Komi et al showed that preactivation rises with increasing running speed. Despite these studies, there is evidence that the vestibular and visual systems also play a role in this process. Finally, it has been shown that the preactivation phase is important in preparing the muscle to resist the high impact force and in preparing for the subsequent push-off after contact. These studies suggest that plyometric training and the motor learning that takes place as a result of repetitive practice can play a critical role in developing proprioception. The speed-strength components of the drills should better prepare a patient to handle these circumstances during functional activity.
As discussed earlier, the concept of TTS is important to consider in plyometric programming, especially with bilateral versus unilateral SSC exercise. For example, plyometric drills, especially in rehabilitation, should progress from bilateral to unilateral modes. There is evidence that the feet touch down at different times, thus suggesting an asymmetry in the landing, with one leg absorbing more energy than the other. In fact, these authors have found bilateral differences in both average and maximum force when drop jumps are performed at less than 0.40 m, which suggests that the feet are placed on the ground at different times; interestingly, symmetry of landing was better with a higher depth jump (0.6 m). Clinicians should, at least grossly, monitor TTS, landing, and takeoff asymmetry when implementing balance and proprioception training as it relates to plyometric exercise even when the intensity is low, as might occur in the rehabilitation setting.
Joint stability depends partially on proper neuromuscular control. Presumably, the clinician has addressed proprioception during the intervention leading up to initiation of a plyometric training program. Nonetheless, the plyometric program should be viewed as a higher-level extension of this component in rehabilitation for a patient to achieve return to maximal functional status. It should be noted that healing and strengthening of the static and dynamic restraints do not necessarily prepare a patient for the demands of athletic endeavors. Indeed, the unanticipated changes in joint positions encountered during athletic events are something that the athlete must be prepared to tolerate. Plyometric training is the next logical step in properly preparing the athlete for return to sport. Although running and changing direction are important aspects of proprioception, these conditions in the athletic training room or clinic do not adequately mimic the functional requirements during competition or even practice drills. Besier et al studied anticipatory effects on knee joint loading during running and cutting maneuvers. They concluded that performance of cutting maneuvers without preplanning may increase the risk for noncontact knee ligament injury. The authors suggested that training should involve drills that familiarize athletes with making unanticipated changes in direction and that plyometrics should be included, as well as helping athletes focus on visual cues to increase the time available for preplanning a movement.
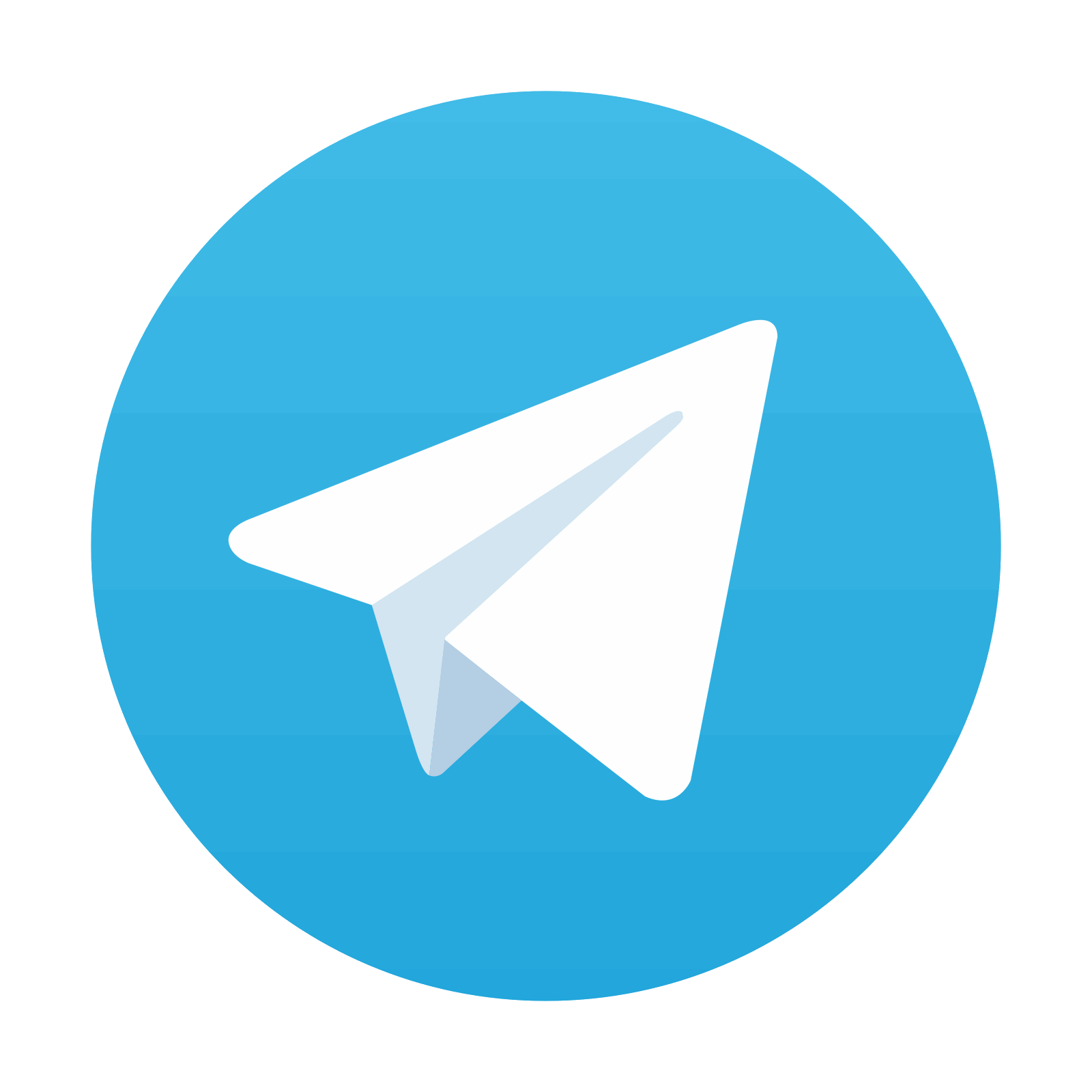
Stay updated, free articles. Join our Telegram channel

Full access? Get Clinical Tree
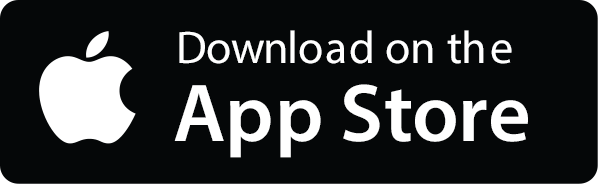
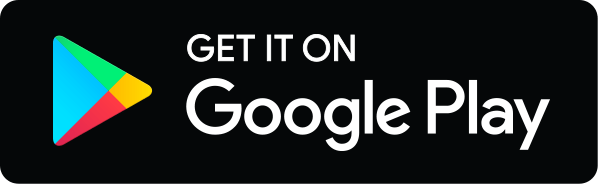