2 Physiology and pathology of the musculoskeletal system
Cases relevant to this chapter
16, 35, 37–48, 50–54, 57–60, 63–65, 67, 76, 81, 92, 95, 98
Essential facts
1. The function of the musculoskeletal system is to allow body movement and to protect and support vital organs.
2. Bone is a reservoir for calcium and phosphate in the preservation of calcium homeostasis.
3. Fractures are classified according to their pattern, causation or relation to surrounding tissues. Fracture healing occurs in five stages: haematoma, organization, callus formation, modelling, and remodelling (completion).
4. Chronic synovitis leads to tissue hyperplasia, oedema and excess synovial fluid production resulting in joint stiffness, pain and loss of full joint function.
Physiology
Types of bone
The skeleton contains two types of bone. These are cortical (or compact) bone, which makes up most of shafts (diaphysis) of the long bones, such as the femur and tibia, and trabecular (also known as medullary) bone, which makes up most of the vertebral bodies and the ends of the long bones (Fig. 2.1).
Bone formation, modelling and remodelling
Bone remodelling
The mechanical integrity of the skeleton is maintained by the process of bone remodelling, which occurs throughout life, in order that damaged bone can be replaced by new bone (Fig. 2.2). Remodelling of the bone occurs in response to alterations in type or amount of mechanical stresses (or to the lack of them). Remodelling can be divided into four phases: resorption, reversal, formation and quiescence. At any one time approximately 10% of bone surface in the adult skeleton is undergoing active remodelling, whereas the remaining 90% is quiescent.
Osteoclast formation and differentiation
Mature osteoclasts form a tight seal over the bone surface and resorb bone by secreting hydrochloric acid and proteolytic enzymes through the ‘ruffled border’ into a space beneath the osteoclast (Howship’s lacuna). The hydrochloric acid secreted by osteoclasts dissolves hydroxyapatite and allows proteolytic enzymes (mainly cathepsin K and matrix metalloproteinases) to degrade collagen and other matrix proteins (Fig. 2.3). Molecules that have been identified as being important in regulating osteoclast activity include carbonic anhydrase II (CA-II), which catalyses the formation of hydrogen ions within osteoclasts; TCIRG1, which encodes a subunit of the osteoclast proton pump, necessary to pump hydrogen ions into the space beneath the osteoclasts; CLCN7, which encodes a chloride channel necessary for transport of chloride ions into the space under the osteoclast; and cathepsin K, which degrades collagen and other non-collagenous proteins. Mutations in the genes that encode these proteins lead to osteopetrosis, which is a disease associated with increased bone density and osteoclast dysfunction. After resorption is completed, osteoclasts undergo programmed cell death (apoptosis), in the so-called reversal phase, which heralds the start of bone formation. It has recently been discovered that many of the drugs that are used clinically to inhibit bone resorption, such as bisphosphonates and oestrogen, do so by promoting osteoclast apoptosis.
Regulation of bone remodelling
Bone remodelling is a highly organized process, but the mechanisms that determine where and when remodelling occurs are poorly understood. Mechanical stimuli and areas of micro-damage are likely to be important in determining the sites at which remodelling occurs in the normal skeleton. Increased bone remodelling may result from local or systemic release of inflammatory cytokines such as interleukin-1 and tumour necrosis factor (TNF) in inflammatory diseases. Calciotropic hormones, such as parathyroid hormone (PTH) and 1,25-dihydroxyvitamin D, act together to increase bone remodelling, which allows skeletal calcium to be mobilized for maintenance of plasma calcium homeostasis. Bone remodelling is also increased by other hormones, such as thyroid hormone and growth hormone, but suppressed by oestrogen, androgens and calcitonin (Table 2.1).
Table 2.1 Stimulators and inhibitors of bone remodelling
Stimulators | Inhibitors |
---|---|
Systemic Hormones | |
Parathyroid hormone | Sex hormonesa |
1,25-Dihydroxyvitamin D | Calcitonin |
Parathyroid hormone-related protein | |
Growth hormone | |
Thyroid hormone | |
Sex hormonesa | |
Locally Acting Factors | |
Interleukin-1b | SOSTc |
Parathyroid hormone-related protein | Interferon gammab |
Tumour necrosis factorb | OPGb |
Insulin-like growth factors | |
RANKLb | |
Wntb |
aSex hormones stimulate bone turnover during skeletal growth, but inhibit turnover during adulthood.
bThese factors mainly affect bone resorption.
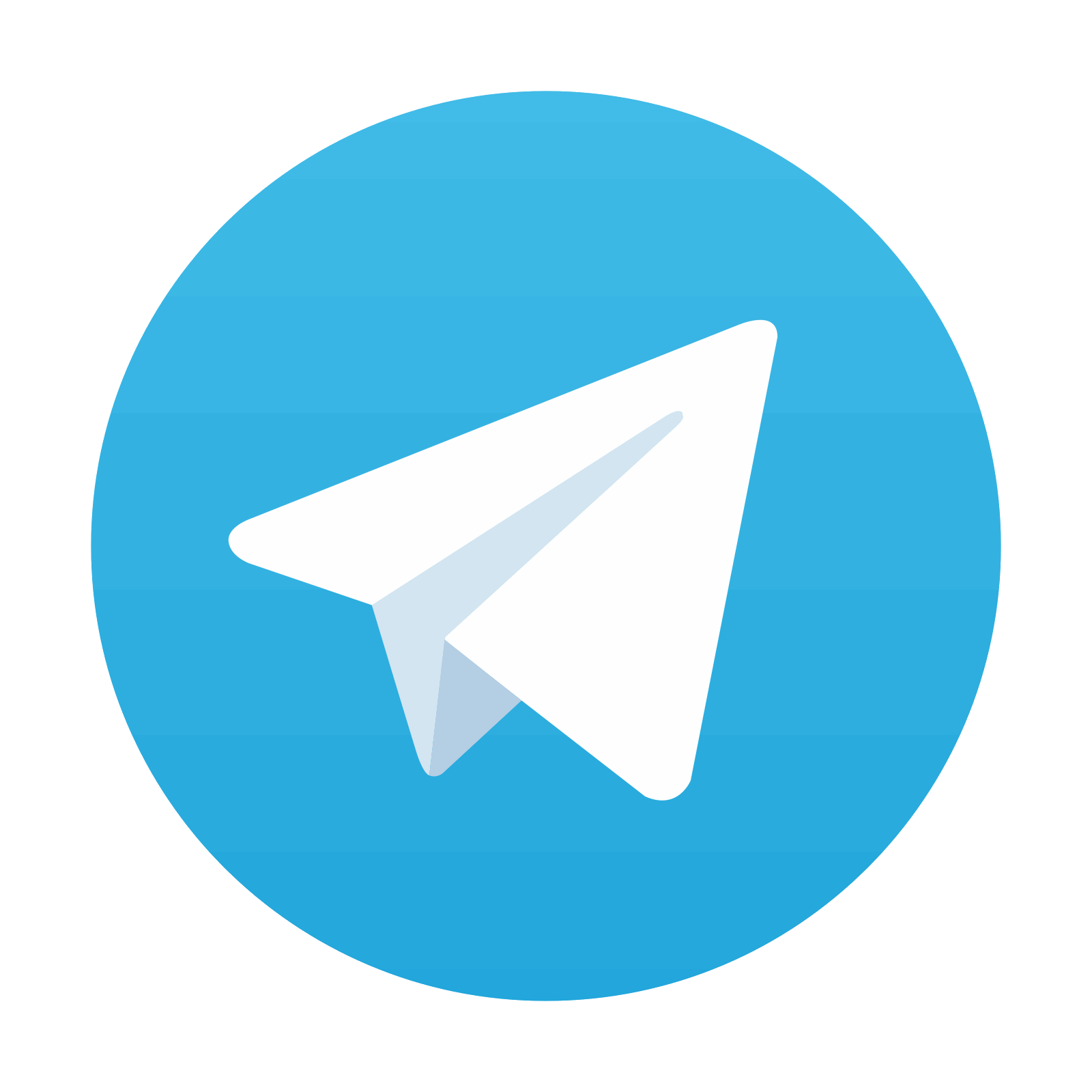
Stay updated, free articles. Join our Telegram channel

Full access? Get Clinical Tree
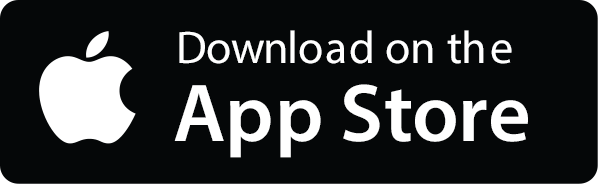
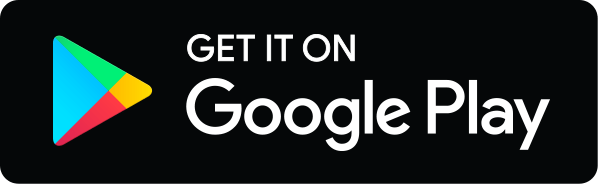