Nutrient artery: blood supply of the inner 2/3 of the cortical bone
Periosteal arterial vessels: blood supply of the outer 1/3 of the cortical bone
Venous system = capacity vessels = venous pooling
Veins are 6–8 times greater than arteries
Blood flow into centrifugal / centripetal direction possible
Blood flow depends on local and systemic factors (e.g. intramedullary pressure, systemic pressure)
Restitution of the intramedullary circulation after 11–12 weeks post-nailing
The nutrient artery and its branches: The nutrient artery enters the bone at the diaphysis [4, 5]. Within the intramedullary cavity the nutrient artery divides into ascending and descending branches [4]. It nourishes the inner two-thirds of the diaphyseal blood supply [4, 5]. The vessels perforate the inner cortex and supply branches of the Haversian canal. The arterial blood flow is in a centrifugal direction.
Metaphyseal/epiphyseal arteries: These arteries enter the bone cortex at the proximal and distal metaphysis/epiphysis and have in-built connections with intramedullary branches [4, 5]. A better blood supply has been described in the proximal shaft of the femur when compared with the distal end [6].
The intramedullary venous vessels have been named “capacity vessels” [4, 9, 10]. Their thin and flexible walls are known to have large blood capacity. In addition, their diameter is six to eight times greater than that of the arteries and there are more veins in the intramedullary cavity than arterial vessels [11]. Numerous venous sinusoids communicate with each other and central venous sinuses are extremely distensible, which allows venous pooling. Venous outflow is dependent on local and systemic factors and is regulated by active and passive mechanisms [10]:
Passive mechanisms are variations of the systemic blood pressure and changes of circulation within the adjacent musculature [10]. Moreover, pressure changes induced by the respiratory system or obstruction of outflow also affect the direction of venous blood flow [12]. Under physiological conditions, there is a centripetal venous flow within the cortical bone. However, blood flow into centrifugal and centripetal direction is possible [12].
Active mechanisms are muscle contractions that cause a rise of intramedullary pressure and affect the direction of the blood flow [10]. In addition, the activation of neural and biochemical mechanisms influence the capacity of venous sinuses within the intramedullary cavity [10]. Phlebographic investigations demonstrate that the venous blood mainly leaves the long bone at its proximal and distal ends [13].
Intramedullary reaming causes a sustained impairment in arterial and venous blood supply [14, 15]. A direct correlation between the extent of intramedullary reaming and the magnitude of the reduction in cortical blood flow has been found [16, 17]. Reaming appears to affect the perfusion of the inner two-thirds of the cortex [14]. The restoration of the bone circulation and perfusion at preoperative levels is obtained at approximately 11 or 12 weeks post-nailing [18, 19]. Moreover, intramedullary reaming may cause relevant blood loss. Studies have shown that reaming-associated perioperative blood loss may reach up to 400 ml [20]. Less severely injured patients (Injury Severity Score (ISS) <16) have been associated with significantly higher blood loss than multiple injured patients with ISS >16 (Table 3.2).
3.2.2 Pressure Changes During and After Reaming
Physiologic intramedullary pressure: local and systemic circulation and activation of adjacent musculature can affect both the intramedullary blood flow and changes in intramedullary pressures [21]. These have been found to correspond with systemic blood pressure changes and respiration [22]. The physiologic intraosseous pressure has been measured in sheep (20–43 mmHg), dogs (51.7 mmHg) and baboons (36 mmHg) [22–24].
The local pressure during reaming causes extravasation of fat into the bloodstream around the long bone. Küntscher discussed the role of bone marrow volume in the generation of the intramedullary pressure [1]. A displacement of 20 ml volume was calculated if a nail (length: 370 mm, diameter: 15 mm) is used. Hammer strokes also generate a pressure above diastolic values. Küntscher postulated that this pressure persists several seconds until it decreases back to physiologic values [1]. Others calculated the volume effect of the reamer and identified a displacement of 50 ml volume if a 15 mm reamer is applied [25].
According to the current literature, there is a widespread agreement concerning the pressure generation within the intramedullary cavity:
Even minor displacement of intramedullary contents are associated with a measurable pressure increases. Subtle instrumentation (e.g. opening of the femoral cavity or even the insertion of the guide wire) have been shown to increase the pressure within the bone cavity [26].
The highest pressure is measured during the initial steps of the reaming procedure when bone marrow is within the intramedullary cavity [26]. In case of a fracture, the maximum pressure is achieved when the reamer enters the distal fragment [15]. The longer this fragment, the higher the pressure rise will be. In the proximal bone fragment intramedullary pressure is reduced due to the leakage of bone marrow into the fracture gap [26].
The characteristics and localization of the fracture also have an influence on the generation of intramedullary pressure [25]. Lower intramedullary pressure was measured in patients with fractured femora in comparison to measurements in non-fractured femora [25]. In simple undisplaced fractures, higher pressure generation is more likely than in a comminuted fracture situation. Moreover, patients with impending or pathological fractures are at risk to develop embolic complications associated with intramedullary nailing [27]. The intramedullary canal is filled with tumor and there is no outflow in the fracture gap. This may lead to massive bone marrow embolism due to pressure generation and large venous sinuses due to enhanced blood flow around the tumor.
Intramedullary pressure up to 200 mmHg can be measured during the reaming process (Fig. 3.1). Several studies analyzed factors and techniques resulting in reduced pressure generation during reaming. The compression force appears to be important for the degree of volume effect [28–30]. Pressure can be reduced by using sharp cutting edges and increased reaming speed (Fig. 3.2a, b) [29, 30]. Reamer design and reaming techniques affect the intramedullary pressure as well [30]. Sharp reamers were associated with reduced intramedullary pressure and attenuated pulmonary artery pressure.



Fig. 3.1
Measurements of the intramedullary pressure during reaming of the endomedullary canal. Blue line demonstrates the intramedullary pressure. Physiologic values were measured with 20–30 mmHg. The initial reaming increased the intramedullary pressure to the values up to 200 mmHg (Published in Pape et al. [103])

Figure 3.2
(a, b) Reamer design and associated pulmonary artery pressure. Blunt reamers (Group A and B) caused increased intramedullary pressure and systemic liberation of intramedullary content leading to obstruction of pulmonary arterial vessels and consecutive increased pulmonary arterial pressure. In sharp reamers (Group H) this relationship has not been observed (Modified according to the publication Pape [104])
3.2.3 Heat Generation
Numerous investigations have measured considerable heat generation as a result of intramedullary reaming (Table 3.3). In some cases, the heat leads to bone necrosis with considerable consequences. Mechanical cutting, friction between reamer and bone and local contact pressure between reamer and bone are the main reasons for the increase of the temperature [31]. Repeated reaming with more than 3–5 steps may result in thermal necrosis of the bone and alterations in endosteal architecture [32]. The temperature of 56 °C is known to be a critical value for the initiation of thermal necrosis. This is the point at which alkaline phosphatase becomes denatured within the bony tissue [33]. Extensive necrosis has been reported when the temperature exceeds 70 °C [34]. However, the duration of the reaming procedure plays a major role as well. A consistent temperature of 47 °C for 1 min results in cortical necrosis and impaired bone formation [35]. The clinical use of large and blunt reamers should be avoided in order to prevent unfavorable elevation of the temperature [30, 36]. Moreover, reaming to 1.5 mm above the appropriate diameter of the nail has been shown to be safe clinical practice [37].
3.2.4 Effects on Bone Healing
Although negative effects on local bone circulation and intramedullary heat generation are present after reaming; clinical observations have documented positive effects on fracture healing [38–40]. Clinical studies have shown that intramedullary reaming enhances the bone healing process and is associated with lower incidence of non-union and delayed union [38–40]. Authors pointed out that this phenomenon may have a multifactorial genesis [41, 42]:
Increased perfusion and oxygenation in adjacent soft tissues due to intramedullary reaming positively affects fracture healing [18, 21, 43].
The osteogenic and osteoinductive properties of reaming debris has also been identified as a factor [44, 45]. Bone debris contains pluripotent stem cells and growth factors that accelerate fracture repair. Clinical and experimental studies support the theory of “autografting”, which stands for local grafting of bone debris into the fracture line occurs during intramedullary reaming [45–48]. Schmidmaier and co-authors measured elevated levels of fibroblast growth factor (FGF), platelet derived growth factor (PDGF), Insulin like growth factor 1 (IGF-1), transforming growth factor β (TGF-β), and bone morphogenetic protein 2 (BMP-2) in bone debris [48]. These factors are known to stimulate the recruitment of progenitor cells and enhance the angiogenesis and osteogenesis within the fracture gap [49, 50]. The measured effects of “autografting” were comparable to conventional bone grafting [48]. In addition, there is evidence indicating that osteoblastic cells may survive the reaming process despite the generation of the higher temperatures [41, 44, 45, 51].
The systemic liberation of relevant growth factors (vascular endothelial growth factor (VEGF), PDGF, IGF-1 and TGF-β) may stimulate the fracture healing as well. Giannoudis and co-authors measured the systemic appearance of these molecules as a result of reaming of the intramedullary cavity [42]. Authors described that in addition to the local production of the graft debris, increased systemic release of growth factors was measurable.
Improved stabilization due to the insertion of nails with large diameter in comparison to unreamed nailing may be another factor affecting improved fracture healing. The nail-to-bone contact (also known as working length) is enlarged after reaming and results in improved stability. However, it must be noted that endosteal bone contributes to the rigidity and strength of the midshaft region only. The cadaveric study performed by Pratt et al. has shown that torsional strength is reduced remarkably when the ratio of endosteal reaming diameter divided by the periosteal diameter exceeds 1/2 [52].
3.3 Effects of the Reaming, Irrigation, Aspiration (RIA) Technique
3.3.1 Indications and Clinical Effects
The application of the reaming, irrigation and aspiration (RIA) system allows the suction of intramedullary content while reaming [15, 26, 53]. It was mainly developed to reduce the amount of systemic fat liberation. Irrigation decreases the viscosity of the bone marrow and facilitates suction prior to the reaming. Moreover, this has been shown to cause less sustained increases in intramedullary pressures. Pape et al. reported a higher systemic response (D-dimer levels and increased pulmonary permeability) in sheep subjected to conventional reamed intramedullary nailing with lung contusion than in animals reamed using the RIA system [54]. These results were confirmed by Schult et al. reporting significant superior results of rinsing-suction-reaming in comparison to conventional reamers in pig femora with regard to development of intramedullary pressure and fat embolization [55]. Whether the RIA-System is feasible to decrease reaming associated embolization and associated systemic complications is still controversial and has to be further studied [53, 56]. Furthermore, lower maximum reaming temperature has been reported by Higgins et al. using RIA compared with traditional reamers [57].
A secondary effect of RIA is the collection of bone, if the eluate is sucked through a filter. It may then be used to harvest intramedullary graft for surgical procedures such as treatment of non-unions and delayed-unions [58]. This technique has been shown to be associated with less donor site morbidity and lower rates of complications when compared with conventional methods (e.g. iliac crest) [59]. Clinical results demonstrate that up to 90 and 33 cm3 of the intramedullary bone graft can be removed from femur and tibia, respectively [60]. The obtained bone graft has been proven to have excellent osteogenic properties [48]. In addition, RIA technique can be applied to patients with acute or chronic osteomyelitis for irrigation and aspiration of potentially infectious intramedullary content.
3.3.2 Technical Application of the RIA
Caution in using this system should be taken in patients with osteoporosis, osteopenia and anatomical abnormalities of femoral bone. Prior to application of the RIA system the exact diameter of the narrow midshaft canal has to be obtained. The great trochanter is used as entry point [61]. After positioning of the guide wire, advance/withdraw/pause/advance technique is applied to maximize the irrigation flow. While reaming, frequent monitoring of the reaming head on both anterior-posterior and lateral plane is recommended to avoid bone perforation or excessive thinning. It must be kept in mind that the endosteal damage of blood vessels can be associated with bleeding. Scharfenberger and Weber evaluated the blood loss during the RIA technique [48]. They reported decreased hemoglobin levels by a mean of 4 g/dl (2.3–8 g/dl). This has been explained by peri-operative dilutional effects. The most relevant complication is the thinning of the inner intramedullary cortex. Lowe et al. reported that femoral fractures may occur after RIA procedure [62]. Especially patients with intramedullary reaming of more than 2 mm of the inner cortex or more than 50 % of the outer diameter are at risk of developing fractures at the post-operative course. In such cases partial weight bearing is recommended. Further minor complications such as femoral anterior cortex perforation and discomfort after intramedullary harvest were reported by Belthur and colleagues [63].
3.4 Systemic Effects and Treatment Strategies
3.4.1 Effects of Fat Intravasation on the Pulmonary System
Clinical and animal studies indicate that increased intramedullary pressure over the diastolic values may result in extra-vasation of bone marrow content [64]. Up to 80 ml of fat have been calculated to be liberated from the lower limbs [64– 67] (Fig. 3.3). Intraoperative analysis with transesophageal echocardiography (TEE) showed that pulmonary embolization takes place when intramedullary pressure exceeds a value of 200 mmHg [68]. Clinical observations have shown that patients subjected to instrumentation of long bones were associated with transient vascular changes (increase of pulmonary arterial pressure, reduced oxygenation, and right heart dilatation) and systemic alterations (Fig. 3.4) [69]. Clinical observations demonstrate a higher rate of acute respiratory distress syndrome (ARDS) in patients with chest injuries if they underwent intramedullary nailing of femoral shaft fractures [70]. In an animal study using a sheep model, reamed nailing and unreamed nailing were compared in the presence of chest trauma [71]. Reamed nailing was associated with a higher degree of fat embolization than did unreamed nailing. Moreover, it was also associated with an increase in pulmonary arterial pressures and worsening of lung function [71]. Clinical studies have revealed that even in the absence of chest trauma, patients who underwent unreamed femoral nailing suffered a transient deterioration in pulmonary oxygenation [72]. This observation was more sustained when reamed nailing was performed [72].



Fig. 3.3
Liberation of the intramedullary fat during reaming of the femur

Fig. 3.4
Comparison of the oxygenation ratio in patients with reamed (RFN) and unreamed (UFN) femoral nailing. In reaming group significantly reduced oxygenation ratio was measured when compared to ratios measured in unreamed patients (Modified according to the publication Pape [104])
Other studies revealed that head injury must also be considered. Fat embolism may get access to the systemic circulation due to the presence of shunt systems (broncho-pulmonary shunt and subpleural shunt) within the lung [69]. They are open when pathologic pulmonary pressures occur and increase the risk of systemic spread and cerebral embolization. Numerous studies have shown lethal outcome in animals with fat embolization related to cerebral spread [73, 74]. The mortality could not be prevented after fat embolization even by improvement of systemic oxygenation [75, 76]. Moreover, it has been discussed whether further “secondary” mechanisms in addition to mechanical obstruction are present and contribute to the aforementioned complications. According to “Cournand Euler Mechanism” local pulmonary hypoxia may cause reflex vasoconstriction in pulmonary vessels [77]. Others have observed neural reactions to pulmonary embolization leading to arterial spasms [76].
3.4.2 Effects of Fat Intravasation on the Inflammatory System
Several inflammatory mediators, such as Serotonin, Prostaglandin or Thromboxane, may stimulate vasoconstriction and bronchial spasms [78, 79]. Especially Thromboxane has been shown to be elevated after reamed intramedullary nailing in a sheep model [80]. Authors postulated that systemically released Thromboxane may exert pulmonary vasoconstriction and an increase in pulmonary arterial pressure after reaming [69]. Moreover, studies indicate that systemically liberated fatty acids and bone debris induce vasculitis of vessels within the lung [81, 82]. After the intra-venous application, fatty acid binds to biologic membranes and blocks sodium and Na + −K + ATPase in epithelial cells [83, 84]. Thereafter, necrosis of epithelial and endothelial cells increases vascular permeability and alveolar congestion occurs. In addition, it has been summarized that neutrophil cell activation may be involved in the pathophysiology of fatty acid induced lung injury [85, 86]. The final common path of the lung injury is the migration of neutrophil cells, due to local release of chemotactic acting chemokines and cytokines (IL-6, IL-1β, TNF-α, etc.) [87]. In addition, enzymes (protease, elastase, etc.), produced by neutrophil cells, damage the lung parenchyma including pneumocytes I and II. It results in interstitial and alveolar oedema, lack of surfactant, alveolar collapse, lung failure (Acute Respiratory Distress Syndrome (ARDS)) and hypoxia [87]. Furthermore, increased activation of the coagulation system has been observed in patients receiving reamed femoral nailing [88]. Moreover, other authors reported that prophylactic reamed intramedullary nailing for pathologic fractures was associated with increased marked coagulation [89]. One explanation might be the observation of increased levels of thromboplastin after musculo-skeletal surgical interventions and associated high risk of thrombosis [90]. Thromboplastin is a known mediator for activation of coagulation system.
3.4.3 Patient Assessment for Staged Management of Reamed Long Bone Fracture Fixation
In patients with penetrating trauma, the “lethal triad” including hemorrhagic shock, hypothermia and coagulopathy has been described [54]. In patients with blunt trauma, soft tissue injuries to extremities and trunk (thorax and abdomen) have been shown to be equally relevant to the patient clinical course [54]. Therefore, in order to avoid life-threatening systemic complications associated with aforementioned fat extravasation, patients with multiple blunt trauma and long bone fractures have to be assessed for “four pathophysiologic cascades” (hemorrhagic shock, coagulopathy, hypothermia and soft tissue injuries) (Fig. 3.5). These cascades are of immense importance for the post-traumatic course due to their common end point inflammatory alterations that result in endothelial damage [54].


Fig. 3.5
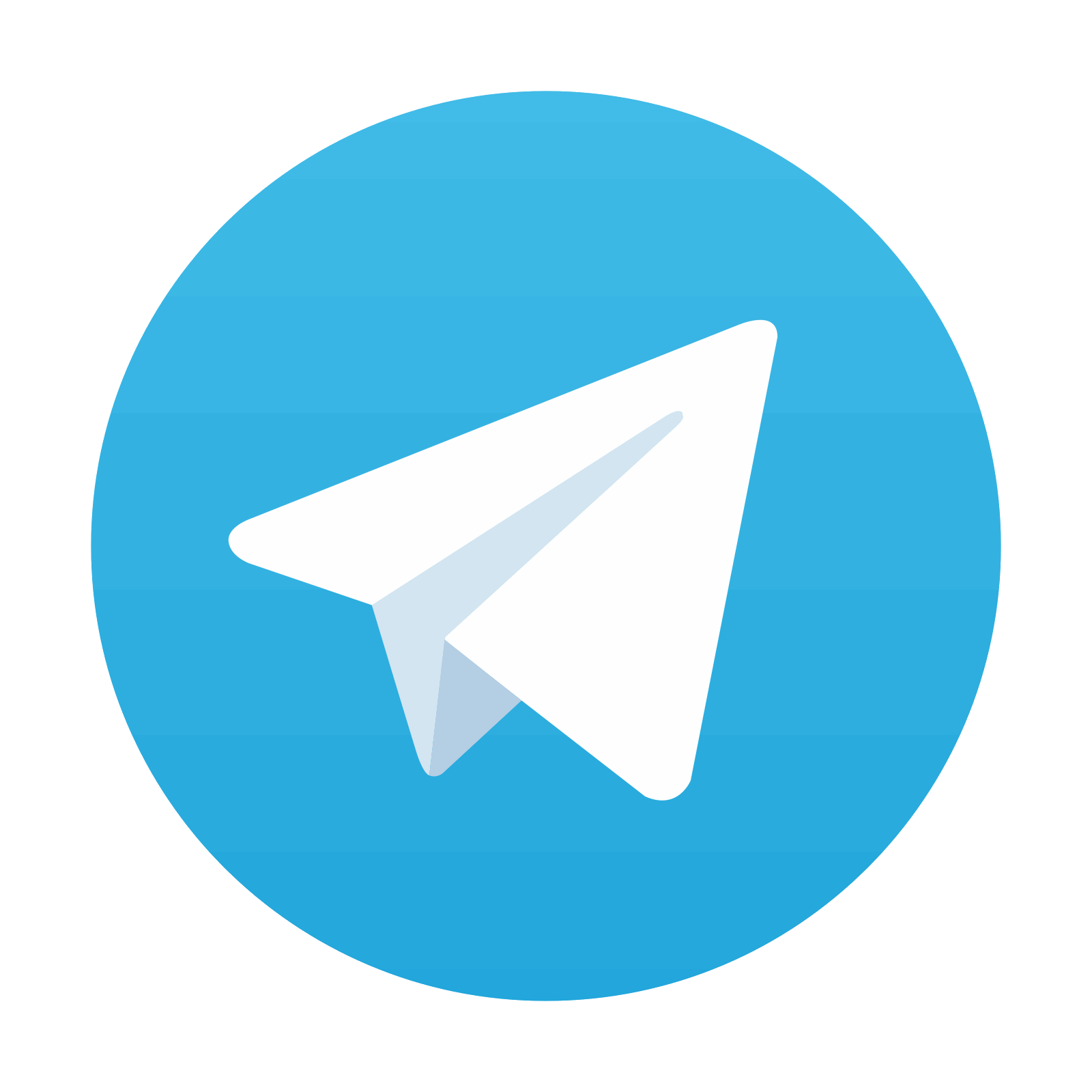
Four vicious cycles demonstrate the pathophysiological cascades associated with the development of post-traumatic immune dysfunction and endothelial damage. The exhaustion of the compensatory mechanisms results in development of systemic complications such as acute respiratory distress syndrome (ARDS) or multiple organ failure (MOF)
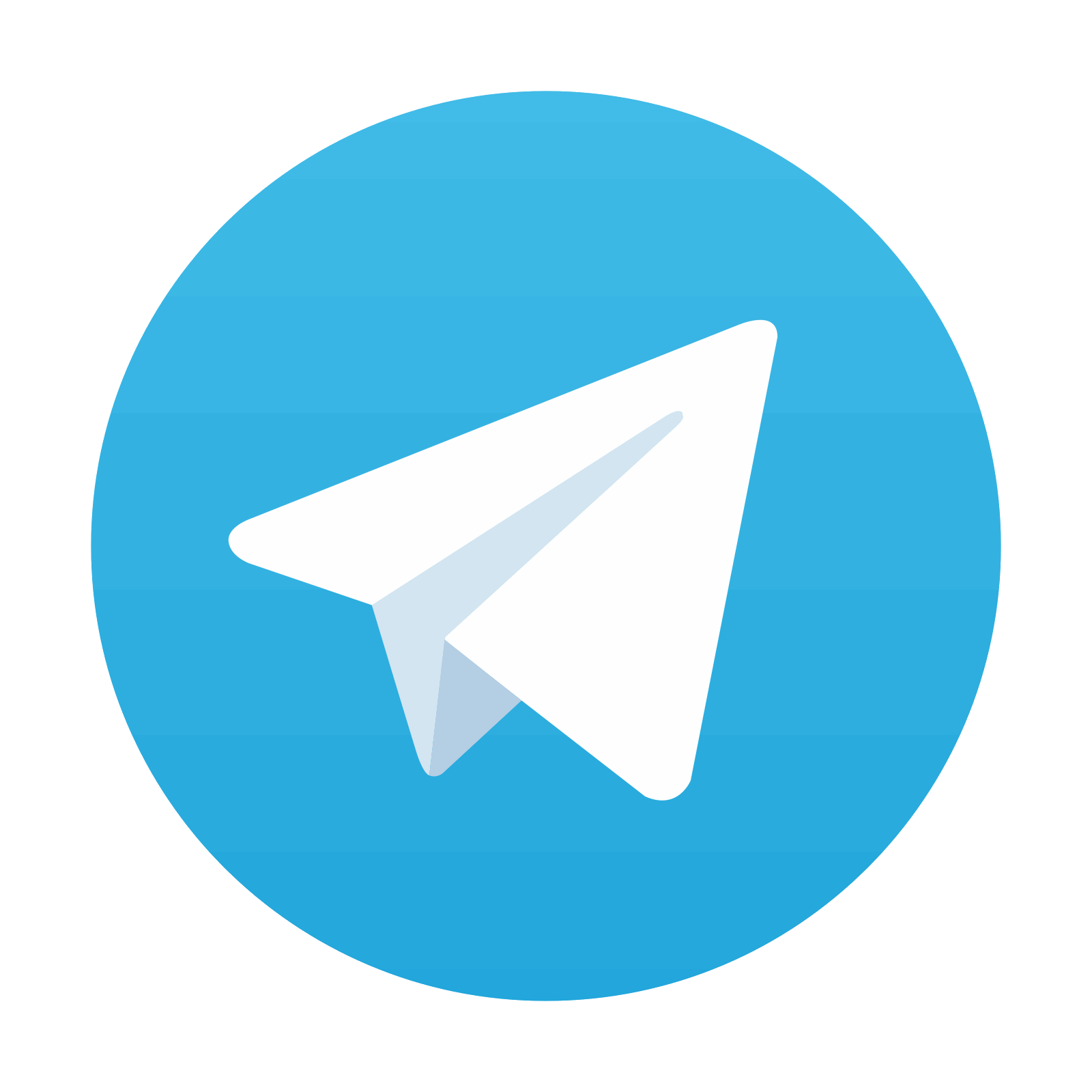
Stay updated, free articles. Join our Telegram channel

Full access? Get Clinical Tree
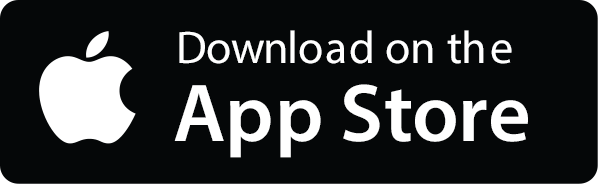
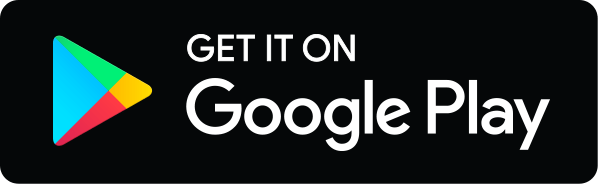
